Abstract
Purpose
Postmenopausal osteoporosis (PMOP) is one of systemic bone degenerative diseases characterised by decreased bone mineral density (BMD). Previous studies suggest that the SPON1 gene may be associated with BMD and play an important role in the occurrence and development of PMOP. In this study, we aimed to investigate the potential association between PMOP and the SPON1 gene.
Methods
A total of 8062 postmenopausal women comprising 2684 primary PMOP patients, and 5378 healthy controls were recruited. Forty tag SNPs were selected for genotyping to evaluate the association of the SPON1 gene with PMOP and BMD. Genetic association and bioinformatics analyses were performed for PMOP.
Results
SNP rs2697825 was identified to be significantly associated with the risk of PMOP at both allelic (T-statistics = −3.84, p = .0001) and genotypic levels (χ2=15.86, p = .0004). The G allele of SNP rs2697825 was significantly associated with a decreased risk of PMOP with an OR [95%] of 0.84 [0.77–0.92]. The G allele of SNP rs2697825 was associated with increased BMD at both the lumbar spine and femoral neck.
Conclusions
Our results provide further evidence to support the important role for the SPON1 gene in the aetiology of PMOP, adding to the current understanding of the susceptibility to osteoporosis.
Introduction
Postmenopausal osteoporosis (PMOP) is a common bone degenerative disease affecting more than 40% of postmenopausal women [Citation1]. Insufficient new bone formation or excessive resorption leads to bone remodelling and bone loss, which ultimately results in low bone mineral density (BMD), bone fracture, chronic pain, and even disability [Citation2]. Previous evidence has shown that BMD may be influenced by multiple environmental factors, such as age, vitamin D levels and body weight [Citation3]. However, a strong genetic component to BMD has been firmly established, with genetic factors estimated to account for up to 50–80% [Citation4]. Many susceptibility genes for PMOP, especially cytokines and osteoprotegerin, which is involved in bone remodelling balance, have been identified [Citation5,Citation6].
SPON1 (also known as F-spondin and VSGP), an important member of the thrombospondin (TSR) family, is of particular importance in the regulation of neuronal outgrowth [Citation7]. However, many studies have found that SPON1 is not only expressed in the nervous system but also in the ovary [Citation8], periodontal tissue [Citation9] and osteoarthritic cartilage [Citation10]. Recently, researchers have demonstrated that the TSR domain of SPON1 can bind to and active TGF-β in cartilage explants [Citation11]. In addition, the TSR domain of SPON1 can associate with TGF-β-dependent pathways, which may promote chondrocyte terminal differentiation and osteogenesis in chick tibia [Citation12]. TGF-β is an important regulator of bone metabolism balance and can directly promote preosteoblast differentiation and inhibit mature osteoclasts [Citation13,Citation14]. Hence, SPON1 may influence BMD and contribute to the risk for PMOP by affecting the TGF-β pathway in cartilage and bone. More importantly, SPON1 knockout mice exhibited reduced levels of TGF-β1 in serum and in cultured costal chondrocytes compared with wild-type mice. More importantly, SPON1-/- mice had a 60% higher bone volume of the femurs and tibiae at 6 months of age [Citation15]. In vitro, SPON1 has been shown to directly stimulate the differentiation and proliferation of osteoblasts and decrease osteoclast differentiation in periodontal tissue [Citation16]. These results suggest that SPON1 may be associated with bone remodelling balance and BMD, which may lead to the occurrence and development of PMOP.
Recently, Gregson et al. identified rs2697825 in SPON1 as a novel single nucleotide polymorphism (SNP) for total hip BMD by a genome-wide association study (GWAS) involving over 2195 Caucasian individuals [Citation17], which further indicates that the SPON1 gene is associated with BMD. Moreover, low hip BMD has been reported as a main clinical manifestation in PMOP patients. Therefore, we speculate that the SPON1 gene may be a candidate gene for PMOP. However, until now, the association of the SPON1 gene with BMD was only identified in samples from individuals with European ancestry and has never been validated in Asians. Therefore, given that different ethnic populations may exhibit genetic heterogeneity of BMD and osteoporosis, large-scale studies in non-European populations are necessary, which would not only determine whether the association of the SPON1 gene with BMD can be generalised to the non-European population but also evaluate the association between the SPON1 gene and PMOP. Hence, in order to confirm whether the SPON1 gene was universally associated with the risk of PMOP, in the present study we systematically investigated the relationship between genetic polymorphisms of the SPON1 gene and PMOP in the Han Chinese population.
Methods
Study subjects
A total of 2684 primary PMOP patients were recruited from Luoyang Orthopaedic Hospital of Henan Province (Luoyang City), and 5378 postmenopausal women without PMOP were enrolled from the same hospital. All subjects were unrelated individuals, and born locally to ensure homogeneity in genetic background of study subjects. The exclusion criteria included the following: (1) migration history in the previous three generations; (2) history of medication for the treatment of osteoporosis; (3) history of medication known to affect bone metabolism used within six months; (4) severe diseases, such as tumours or severe dysfunction of important organs (heart, lung, liver, or kidney, etc.). The BMD in the study subjects at the lumbar spine (LS2–4) and femoral neck (FN) were measured by dual-energy X-ray absorptiometry (Lunar Expert 1313, Lunar Corp., USA). The diagnosis of PMOP was based on World Health Organisation standards. Additionally, peripheral blood was collected from all study subjects in the morning after a 12 h fast. Serum levels of multiple bone turnover markers (BTMs), including bone-specific alkaline phosphatase (BAP), C-terminal telopeptide of type I collagen (CTX), osteoprotegerin (OPG), osteocalcin (OST), and soluble receptor activator of nuclear factor kappa-B ligand (sRANKL), were measured using enzyme-linked immunosorbent assay (ELISA) kits (Westtang Biotech Inc., Shanghai, China) according to a standard protocol. The demographic and clinical characteristics of all subjects were obtained from questionnaires and medical records. Written informed consent was provided by all study subjects. This research was performed in accordance with the ethical guidelines of the Declaration of Helsinki (version 2002) and was approved by the Ethics Committee of Luoyang Orthopaedic Hospital of Henan Province.
SNP selection and genotyping
We extracted 622 SNPs with minor allele frequency (MAF) ≥0.1 within the SPON1 gene region from the 1000-Genome Project Han Chinese data. Then, SNP tagging with r2 ≥0.5 was performed on this set of SNPs. A total of 40 tag SNPs were ultimately selected for genotyping (Supplemental Table S1). Genomic DNA was extracted from peripheral blood leukocytes using a Genomic DNA Kit (Axygen Scientific, Inc., CA, USA) according to the manufacturer’s protocol. SNP genotyping was performed by high-throughput Sequenom MassARRAY Platform (Sequenom, San Diego, CA, USA). In order to estimate the genotyping quality, the genotyping of 5% samples was repeated. With a concordance rate of 100%, the quality of genotyping data was confirmed. The case/control status of the samples was blinded to the technicians during the genotyping process.
Statistical analyses
Single marker-based genetic association analyses of the risk of PMOP were performed for all of the genotyped SNPs. Logistic models were fitted for each SNP with age adjusted as a covariate. SNPs were coded in additive mode in which an individual was coded as 0, 1 or 2 when there were 0, 1, or 2 copies of a minor allele. Genotypic analyses were conducted by χ2 tests. Genetic association analyses were also performed by linear Regression on quantitative traits including BMD in the lumbar spine and femoral neck, and linear models were fitted with age adjusted as a covariate. Bonferroni corrections were applied for multiple comparisons. For single marker-based association analyses, 0.05/40 = 0.00125 was utilised as the threshold of p values. Plink [Citation18] was utilised for genetic association analyses, and Haploview [Citation19] was used to make linkage disequilibrium (LD) plots. The functional consequences of the significant SNP were examined through its eQTL signals on SPON1 using data obtained from the GTEx database [Citation20]. Data on the gene expression of SPON1 for different genotypes of the significant SNP were obtained in 47 human tissues.
Results
A total of 8062 postmenopausal women comprising 2684 primary PMOP patients, and 5378 controls were recruited. The characteristic and clinical information of these study subjects are summarised in . No significant differences were found for years after menopause and BMI between PMOP patients and healthy controls. There were significant differences in age, BMD of the lumbar spine and femoral neck, and all five BTMs. The LD plot is shown in Supplemental Figure S1. Among the 40 genotyped SNPs, only one SNP, rs2697825, was identified to be significantly associated with the disease status of PMOP in the study subjects (, Supplemental Table S2). The G allele of SNP rs2697825 was significantly associated with a decreased risk of PMOP with an OR [95%] of 0.84 [0.77–0.92] (). The genetic association signal of SNP rs2697825 was obtained at both allelic (T-statistics = −3.84, p = .0001) and genotypic levels (χ2=15.86, p=.0004). Then, we examined the association between SNP rs2697825 and quantitative traits of PMOP, including BMD at the lumbar spine and femoral neck. SNP rs2697825 was significantly correlated with BMD at both sites (Supplemental Table S3). The G allele of SNP rs2697825 was associated with increased BMD at both the lumbar spine and femoral neck (). This finding was in accordance with the association signal of this SNP with PMOP disease status. In addition to the significant signals from SNP rs2697825, we also identified several SNPs located around rs2697825that were significantly associated with BMD. To confirm whether these signals represented independent effects, we further conducted conditional analyses to explore the association signals after conditioning on SNP rs2697825. The results of the conditional analyses showed that all of the significant signals were dependent on SNP rs2697825 (Supplemental Table S4). All of the signals were no longer significant after controlling for SNP rs2697825 genotypes. We examined the eQTL signals of SNP rs2697825 on SPON1 using data extracted from the GTEx database. Two significant eQTL signals were obtained from this database (). One is from human heart tissue (T-statistics = 6.30, NES = 0.45, p = 1.60 × 10−9), and the other is from testis (Supplemental Table S5). In this study, we recruited postmenopausal women as study subjects; therefore, the signal from the testis was excluded from the present study.
Figure 2. BMD at the lumbar spine and femoral neck in different genotype groups of SNP rs2697825. (a) BMD at the femoral neck. (b) BMD at the lumbar spine.
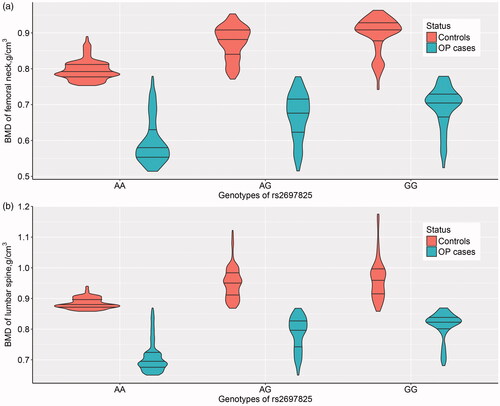
Table 1. Information on the characteristics of the study subjects recruited in this study.
Table 2. Genetic association between SNP rs2697825 and postmenopausal osteoporosis.
Discussion
In the present study, we identified a significant association signal from SPON1 that contributes to the risk of PMOP in the Han Chinese population. To the best of our knowledge, our study is the first to investigate the association between SPON1 and the genetic susceptibility to PMOP in Chinese populations. In the previous GWAS of BMD conducted by Gregson et al. [Citation17], the G allele of SNP rs2697825 was associated with an increase in hip BMD (β = 0.310, p = 4.93 × 10−8). The BMD of the lumbar spine was also examined in a previous study but was not reported as significant because of corrections for multiple comparisons. The effect size of BMD of the lumbar spine was 0.188 and was in the same direction as the effect size in our study (β = 0.073 in PMOP patients and 0.054 in controls). Therefore, our findings of the association between BMD of the femoral neck and the lumbar spine and SNP rs2697825 could validate previous GWAS findings based on populations with European ancestry. In addition, we took our analysis a step further to show that this SNP was also significantly associated with PMOP and that its minor allele had a protective effect on PMOP.
SNP rs2697825 is an intronic variant in SPON1; thus, it cannot alter the protein sequence encoded by SPON1. In a previous GWAS study, researchers identified SNPs in LD with rs2697825 to be associated with the gene expression of BTBD10, and these eQTL signals survived Bonferroni correction. However, BTBD10 has not been previously associated with bone regulation. In the present study, we examined the eQTL signal of rs2697825 on SPON1 in multiple human tissues using data from the GTEx database. We identified significant signals in human heart tissue, and according to GTEx data, the G allele of rs2697825 was associated with increased expression levels of SPON1. However, this finding could only provide indirect evidence for the functional consequences of SNP rs2697825. In the present study, the G allele of this SNP was associated with increased BMD levels and a decreased risk for PMOP. An early study using animal models identified that, compared to wild-type mice, spon1 knockout mice exhibited reduced levels of TGF-β1 in serum and in cultured costal chondrocytes and increased bone mass of femurs and tibiae at 6 months of age [Citation15]. They concluded that Spon1 deletion leads to a local and systemic reduction of TGF-β levels resulting in increased BMP signalling and increased bone deposition in adult mice. Therefore, the G allele of SPON1 is supposed to be associated with a decreased level of SPON1 expression. Limited by the study design, we cannot test the eQTL signal in PMOP-related tissue, such as osteoblasts or osteoclasts, in the present study. In the future, eQTL analyses focussing on SNP rs2697825 would be informative to unravel the functional consequence of SNP rs2697825.
Two potential explanations could be made for the genetic association signals of SNP rs2697825. The first is that this SNP itself plays a role in the regulation of SPON1 gene expression. Another possibility is that this SNP is a surrogate of a DNA variant with true biological effects that has not yet been genotypes. In this present study, we do not have any evidence to confirm either assumption. In future studies, functional analyses on SNP rs2697825 could offer information on its role in the regulation of SPON1 gene expression. In addition, several previous genetic studies of complex disorders have shown that rare variants could also contribute significantly to the risk of diseases [Citation21–23]. Therefore, to investigate the genetic architecture of SPON1, a sequencing-based study with all potential DNA variants genotyped was necessary.
With the fast development of sequencing technology, although numerous genetic variants have been reported to be associated with complex diseases [Citation24–27], those variants account for only a small percentage of the estimated heritability in some populations [Citation28–30]. Although some significant association signals were identified in the study, our study has several limitations. First, in the present study, we only recruited postmenopausal women, and this might be a problem for the generalisation of our study results. Although our results were comparable with the previously published GWAS based on study subjects of both genders, study subjects from both genders are still needed in future replication studies. Another limitation is that potential population stratification might have confounding effects on the significant signals. As this is a candidate gene-based association study, we cannot apply statistical procedures such as principal component analysis to correct for this potential confounder in the statistical analysis process. This problem might be even worse when considering that the Chinese Han population has a highly diverse genetic background [Citation31]. One measure we have taken is to restrict the genetic background of our study subjects in the recruitment process. Study subjects with migration histories in the previous three generations were excluded from this study. We believe that in this way, we could, at least partly, control the potential population stratification in our study. Finally, some potential confounders influencing the risk of PMOP, such as breastfeeding, alcohol consumption, and physical activity were not taken into consideration in the study. Thus, it would be necessary to confirm our results in other ethnical populations in future studies.
In summary, our results provide further evidence supporting the association of the SPON1 gene with PMOP in postmenopausal Han Chinese women, which suggests that rs2697825 in the SPON1 gene conferring risk of PMOP may be useful in the informative assessment of the genetic risk for reduced BMD.
Author contributions
Authors Ma WL and Wang KZ conceived and designed the study. Ma WL and Chen K carried out candidate SNPs selection and statistical analyses. Ma WL, Chen K, Xiao WQ, Tang HT and Wang SH conducted subject screening. Ma WL, Tang HT and Wang SH contributed to the collection and preparation of control DNA samples. Ma WL wrote the paper.
Supplemental_materials01.docx
Download MS Word (171.3 KB)Disclosure statement
No potential conflict of interest was reported by the author(s).
References
- Rachner TD, Khosla S, Hofbauer LC. Osteoporosis: now and the future. Lancet. 2011;377(9773):1276–1287.
- Cummings SR, Melton LJ. Epidemiology and outcomes of osteoporotic fractures. Lancet. 2002;359(9319):1761–1767.
- Liu S, Li J, Sheng Z, et al. Relationship between body composition and age, menopause and its effects on bone mineral density at segmental regions in Central Southern Chinese postmenopausal elderly women with and without osteoporosis. Arch Gerontol Geriat. 2011;53(2):e192–197.
- Deng HW, Chen WM, Conway T, et al. Determination of bone mineral density of the hip and spine in human pedigrees by genetic and life-style factors. Genet Epidemiol. 2000;19(2):160–177.
- Sengul T, Gamze A, Nurten K, et al. Association between osteoporosis and polymorphisms of the IL-10 and TGF-beta genes in Turkish postmenopausal women. Human Immunol. 2013;74(9):1179–1183.
- Wolski H, Drews K, Bogacz A, et al. The RANKL/RANK/OPG signal trail: significance of genetic polymorphisms in the etiology of postmenopausal osteoporosis. Ginekol Pol. 2016;87(5):347–352.
- Klar A, Baldassare M, Jessell TM. F-spondin: a gene expressed at high levels in the floor plate encodes a secreted protein that promotes neural cell adhesion and neurite extension. Cell. 1992;69(1):95–110.
- Miyamoto K, Morishita Y, Yamazaki M, et al. Isolation and characterization of vascular smooth muscle cell growth promoting factor from bovine ovarian follicular fluid and its cDNA cloning from bovine and human ovary. Arch Biochem Biophys. 2001;390(1):93–100.
- Kitagawa M, Ao M, Miyauchi M, et al. F-spondin regulates the differentiation of human cementoblast-like (HCEM) cells via BMP7 expression. Biochem Biophys Res Commun. 2012;418(2):229–233.
- Attur MG, Palmer GD, Al-Mussawir HE, et al. F-spondin, a neuroregulatory protein, is up-regulated in osteoarthritis and regulates cartilage metabolism via TGF-beta activation. FASEB J. 2009;23(1):79–89.
- Ribeiro SM, Poczatek M, Schultz-Cherry S, et al. The activation sequence of thrombospondin-1 interacts with the latency-associated peptide to regulate activation of latent transforming growth factor-beta. J Biol Chem. 1999;274(19):13586–13593.
- Palmer GD, Piton AH, Thant LM, et al. F-spondin regulates chondrocyte terminal differentiation and endochondral bone formation. J Orthop Res. 2010;28(10):1323–1329.
- Sun J, Zhang C, Xu L, et al. The transforming growth factor-β1 (TGF-β1) gene polymorphisms (TGF-β1 T869C and TGF-β1 T29C) and susceptibility to postmenopausal osteoporosis: a meta-analysis. Medicine (Baltimore). 2015;94(4):e461.
- Chen G, Deng C, Li YP. TGF-β and BMP signaling in osteoblast differentiation and bone formation. Int J Biol Sci. 2012;8(2):272–288.
- Palmer GD, Attur MG, Yang Q, et al. F-spondin deficient mice have a high bone mass phenotype. PLOS One. 2014;9(5):e98388.
- Oka H, Mori M, Kihara H. F-spondin inhibits migration and differentiation of osteoclastic precursors. J Periodontol. 2011;82(12):1776–1783.
- Gregson CL, Newell F, Leo PJ, et al. Genome-wide association study of extreme high bone mass: Contribution of common genetic variation to extreme BMD phenotypes and potential novel BMD-associated genes. Bone. 2018;114:62–71.
- Chang CC, Chow CC, Tellier LC, Vattikuti S, et al. Second-generation PLINK: rising to the challenge of larger and richer datasets. Gigascience. 2015;4:7.
- Barrett JC, Fry B, Maller J, et al. Haploview: analysis and visualization of LD and haplotype maps. Bioinformatics. 2005;21(2):263–265.
- Consortium GT. The Genotype-Tissue Expression (GTEx) project. Nat Genet. 2013;45(6):580–585.
- Zhang TX, Saccone NL, Bierut LJ, et al. Targeted sequencing identifies genetic polymorphisms of flavin-containing monooxygenase genes contributing to susceptibility of nicotine dependence in European American and African American. Brain Behav. 2017;7(4):e00651.
- Zhang T, Hou L, Chen DT, et al. Exome sequencing of a large family identifies potential candidate genes contributing risk to bipolar disorder. Gene. 2018;645:119–123.
- Zheng HF, Forgetta V, Hsu YH, UK10K Consortium, et al. Whole-genome sequencing identifies EN1 as a determinant of bone density and fracture. Nature. 2015;526(7571):112–117.
- Guan F, Zhang B, Yan T, et al. MIR137 gene and target gene CACNA1C of miR-137 contribute to schizophrenia susceptibility in Han Chinese. Schizophr Res. 2014;152(1):97–104.
- Jia X, Zhang T, Li L, et al. Two-stage additional evidence support association of common variants in the HDAC3 with the increasing risk of schizophrenia susceptibility. Am J Med Genet. 2016;171(8):1105–1111.
- Zhang T, Zhu L, Ni T, et al. Voltage-gated calcium channel activity and complex related genes and schizophrenia: a systematic investigation based on Han Chinese population. J Psychiatr Res. 2018;106:99–105.
- Han W, Zhang T, Ni T, et al. Relationship of common variants in CHRNA5 with early-onset schizophrenia and executive function. Schizophr Res. 2019;206:407–412.
- Liu X, Hou Y, Yan T, et al. Dopamine D3 receptor-regulated NR2B subunits of N-methyl-d-aspartate receptors in the nucleus accumbens involves in morphine-induced locomotor activity. CNS Neurosci Ther. 2014;20(9):823–829.
- Zhu L, Li J, Dong N, et al. mRNA changes in nucleus accumbens related to methamphetamine addiction in mice. Sci Rep. 2016;6:36993.
- Li J, Zhu L, Guan F, et al. Relationship between schizophrenia and changes in the expression of the long non-coding RNAs Meg3, Miat, Neat1 and Neat2. J Psychiatr Res. 2018;106:22–30.
- Tian-Xiao Z, Li Y, Sheng-Bin L. Y-STR haplotypes and the genetic structure from eight Chinese ethnic populations. Leg Med (Tokyo)). 2009;11(Suppl 1):S198–S200.