Abstract
High grade-gliomas are highly invasive and prone to metastasis, leading to poor survival and prognosis. Currently, we urgently need a new treatment strategy to effectively inhibit glioma. In this study, artemether and paclitaxel were used as two agents for tumour suppression. Two functional materials were synthesised and modified on the surface of the micelle as targeting molecules. The addition of two functional materials confers the ability of the micelles to effectively cross the blood-brain barrier (BBB) and then target the glioma cells. Thus, this dual-targeted delivery system allows the drug to play a better role in inhibiting tumour invasion and vasculogenic mimicry (VM) channels. In this paper, the anticancer effects of dual-targeted artemether plus paclitaxel micelles on glioma U87 cells were studied in three aspects: (I) In vitro and in vivo targeting assessment, including the role of penetrating BBB and targeting glioma; (II) In vitro regulation of invasion-associated proteins; (III) Inhibition of VM channels formation and invasion in vitro; (IV) The study of pharmacodynamics in tumour-bearing mice. These results suggest that dual-targeted artemether plus paclitaxel micelle may provide a new strategy to treat glioma via inhibiting invasive and VM channels.
Keywords:
Introduction
Gliomas, as one of the most malignant tumours in the human central nervous system, have high morbidity and mortality, and glioma tumour cells have a terrible characteristic of infiltrating growth, which seriously threatens human life and health [Citation1]. It is generally seen that patients with high-grade gliomas have an extremely short survival time (<15 months) [Citation2–4]. The traditional methods for treating glioma are surgical resection combined with radiotherapy, chemotherapy, etc. [Citation5]. However, the treatment effects are unsatisfactory owing to the presence of the blood-brain barrier (BBB), the generation of vasculogenic mimicry (VM) channels, and the high invasion of glioma cells.
Although chemotherapy is one of the chief means in the treatment of glioma, the therapeutic effect is still affected by BBB to a large extent [Citation6]. BBB is a dynamic barrier between the peripheral circulatory system and brain tissue, and its permeability is low [Citation7]. BBB can prevent more than 90% of the drugs from entering the brain tissue, which makes the drug be difficult to work. Numerous studies have demonstrated that enhancing the permeability of BBB for anticancer agent could improve their efficacy conspicuously.
The leading cause of glioma treatment failure and patient death is the high invasion of glioma cells [Citation8]. Sufficient blood supply to the tumour area is indispensable for maintaining the invasion of glioma. The high metabolic requirements of high-grade gliomas lead to the formation of hypoxic regions, which are bitterly prone to form VM in this hypoxic environment [Citation9]. Along with the formation of VM, a large amount of blood is perfused into the tumour area to provide nutrients for the development of glioma [Citation10]. Studies have shown that the destruction of VM channel and inhibition of tumour cell invasion could notably enhance the therapeutic effects for glioma.
Paclitaxel is a high-efficiency anticancer agent, which is extracted from Taxus genus. Paclitaxel has significant therapeutic effects on the various solid tumours including glioma [Citation11,Citation12]. However, it shows unsatisfactory efficacy against invasive gliomas for the poor distribution in tumour site prevented by the BBB [Citation13]. Artemether is a fat-soluble derivative of artemisinin, which has the advantages of rapid absorption and high permeability, and it is often used for anti-cancer research due to the potent cytotoxic activities against tumour cells [Citation14]. Studies have shown that artemether can significantly enhance the VM damage effects [Citation9], and the mechanism is related to the potential down-regulation of matrix metalloproteinase-2 (MMP-2) and hypoxia-inducible factor-α (HIF-α). However, paclitaxel and artemether are inferior in water solubility, and it is tough to maintain an effective concentration in tumour sites.
Bradykinin (BK) is a 9-peptide substance with cardioprotective effect [Citation15]. It can selectively open the BBB via activating BK type 2 (B2) receptor, hence the newly synthesised BK-PEG2000-DSPE was used as a functional molecule for selectively enhancing the permeability of the BBB [Citation9]. P-aminophenyl-a-D-mannopyranoside (MAN) is a type of mannose analog. According to our previous study, the mannose-vitamin E derivative (MAN-TPGS1000) can penetrate the brain efficiently and increase the affinity of drug vectors to brain tumour cells because the over-expressed glucose transporters (GLUTs) on both BBB and glioma cells [Citation16].
In this study, we constructed a dual-targeted artemether plus paclitaxel micelle for enhancing the transporting ratio across BBB, obtaining the active targeting to gliomas cells, inhibiting VM formation and inhibiting the high invasion of glioma cells. In this micelle, both paclitaxel and artemisia are simultaneously encapsulated inside the micelle vesicles as anticancer drug and modulating agent, respectively. The BK-PEG2000-DSPE conjugate acts as a targeting molecule to transport the micelle across BBB. The MAN-TPGS1000 conjugate possesses dual functions of transporting across the BBB and targeting glioma cells. The dual-targeted artemether plus paclitaxel micelles may bring hope to enhance the anticancer efficacy for gliomas. The objectives of the present study were to study the anticancer effects and evaluate its mechanism of action.
Materials and methods
Materials
ICR mice (Initial weight around 20 g) were purchased from Beijing Vital River Laboratory Animal Technology Co., Ltd. All animal-related operations in this study were approved by the Experimental Animal Care Department of Shanxi University of Traditional Chinese Medicine, and we strictly abide by relevant regulations. Human glioma cell line U87 cells were grown at 37 °C in a 5% CO2 atmosphere. The nutrient supply of U87 cells was derived from DMEM medium (Macgene Biotech Co. Ltd., China) containing 10% foetal bovine serum (FBS) and 1% glutamine (Macgene Biotech). Murine brain microvascular endothelial cells (BMVECs) were obtained from the Institute of Clinical Medicine of China-Japan Friendship Hospital (Beijing, China) and cultured in DMEM culture medium which includes 20% FBS, 100 µg/mL streptomycin, 100 μg/mL penicillin, 40 μg/mL heparin, 100 µg/mL endothelial cell growth factor and 2 mmol/L l-glutamine. BK was purchased from China Peptides Ltd (Shanghai, China). Glutaric acid, paclitaxel, artemether, EDCI, MAN, MES and NHS were purchased from J&K Scientific Ltd (Beijing, China). D-α-tocopherol polyethylene glycol 1000 succinate (TPGS1000) was purchased from Sigma-Aldrich (St. Louis, MO, USA). Polyethylene glycol-distearoyl phosphatidyl ethanolamine (PEG2000-DSPE) and COOH-PEG2000-DSPE was purchased from A.V.T. Pharmaceutical Co., Ltd. (Shanghai). Other reagents are analytical or chromatographic grades (Beijing, China).
Synthesis of targeting molecules
The targeting molecule MAN-TPGS1000 was synthesised and characterised according to our previous methods [Citation9]. BK-PEG2000-DSPE was produced by the following method. 5.22 × 10−3 mmol COOH-PEG2000-DSPE, 0.079 mmol EDCI and 0.203 mmol NHS were added sequentially to 2 ml MES buffer solution (0.1 M, pH 5.6). After the solution was stirred at room temperature for 30 min, 0.10 mmol of BK was added and the stir was continued for 12 h. The product was dialysed (molecular weight cut-off point, 3000) with deionised water for 48 h and then lyophilised to obtain BK-PEG2000-DSPE. A matrix-assisted laser desorption/ionization time of flight mass spectrometry (MALDI-TOF-MS instrument, Shimadzu, Japan) was used to characterise the products.
Construction of dual-targeted artemether plus paclitaxel micelles
The film dispersion method was utilised in preparing dual-targeted artemether plus paclitaxel micelles [Citation17,Citation18]. Briefly, all the materials including PEG2000-DSPE, TPGS1000, TPGS1000-MAN, BK-PEG2000-DSPE, artemether and paclitaxel (mass ratio of 37.4:33.6:2.1:4.2:2.1:1) were dissolved in methanol. Then, the solution was rotary evaporated under vacuum at 40–45 °C to form a dry film. The film was hydrated with an appropriate amount of PBS buffer (10 mM, pH 7.4), and the resulting suspension was incubated in a 50 °C water bath for 0.5 h. The dual-targeted artemether plus paclitaxel micelles were obtained after separating the non-encapsulated drugs by filtration through a polycarbonate membrane (pore size 0.22 μm) (Millipore Co., Bedford, MA). The preparation materials of BK modified artemether plus paclitaxel micelles, MAN modified artemether plus paclitaxel micelles, artemether plus paclitaxel micelles and paclitaxel micelles are shown in . The morphology of dual-targeted artemether plus paclitaxel micelles was viewed by transmission electron microscopy (TEM, NSK Ltd., Tokyo, Japan). Nano Series Zen 4003 Zetasizer (Malvern Instruments Ltd, Malvern, UK) was utilised to determine the particle size of dual-targeted artemether plus paclitaxel micelles. In order to detect the in vitro release rate, the micelles were dialysed against a serum-containing release medium for 48 h according to our previous methods, and samples were taken at 2, 12, 24, and 48 h to determine the content of paclitaxel or artemether in the medium.
Table 1. Preparation material of micelles.
Cytotoxicity effects
U87 cells in the exponential growth phase were seeded in 96-well plates at a density of 5.3 × 103 cells/well and incubated overnight. Then, a calculated amount of free paclitaxel, free artemether, a fixed concentration of artemether plus free paclitaxel, blank micelles, paclitaxel micelles, artemether plus paclitaxel micelles, BK modified artemether plus paclitaxel micelles, MAN modified artemether plus paclitaxel micelles and dual-targeted artemether plus paclitaxel micelles were added to the wells separately. The final concentration of paclitaxel was 0-5 μM, and the culture medium was used as blank control. After the cells were incubated for 48 h, the cell viability was measured by the sulforhodamine-B staining (SRB) method, and the absorbance was measured at 540 nm using a microplate reader (HBS-1096A, DeTie, Nanjing, China). Cell Survival rate was calculated by the formula: Survival rate % = A540nm for treated cells/A540nm for control cells × 100%, where the absorbance at 540 nm is expressed as A540nm, Dose-survival curve based on three parallel experimental data. The IC50 values were calculated by GraphPad Prism software Version 6.0 (GraphPad Software Inc., La Jolla, CA).
Cell cycle arrest
U87 cells in the exponential growth phase were inoculated into a 6-well plate at a density of 4 × 105 cells/well. After incubation for 12 h, blank micelles, paclitaxel micelles, artemether plus paclitaxel micelles, BK modified artemether plus paclitaxel micelles, MAN modified artemether plus paclitaxel micelles and dual-target artemether plus paclitaxel micelles were added to each well and continued to incubate for 12 h. The final concentrations of paclitaxel and artemether were set to 5 μM and 30 μM, respectively. The cells were harvested and fixed overnight at −20 °C. Then, all the samples were incubated with 45 µg/ml propidium iodide (PI, Sigma-Aldrich), 45 µg/ml Rnase A (Fermentas) and 0.25% Triton X-100 for 0.5 h in the dark at room temperature. A FACScan flow cytometer (Becton Dickinson Biosciences) was used to evaluate the stained nuclei, and DNA histograms were analysed by FlowJo software.
Induction of apoptosis in U87 cells
A FACScan flow cytometer and a fluorescein isothiocyanate annexin V staining kit (KeyGen Biotechnology Co., Ltd, China) were used to detect the apoptosis effect on U87 cells. In summary, U87 cells were seeded to 6-well plates at a density of 3.8 × 105 cells/well and then incubated overnight. When U87 cells were grown to 85% confluency, the calculated amount of paclitaxel micelles, artemether plus paclitaxel micelles, BK modified artemether plus paclitaxel micelles, MAN modified artemether plus paclitaxel micelles, dual-targeted artemether plus paclitaxel micelles were added to each well. The final concentrations of paclitaxel and artemether were set to 5 µM and 30 µM, respectively. Blank micelles were used as bland control. After 12 h of co-incubation of cells and drugs. The cells were evaluated according to the kit instructions, and the experiments were performed in parallel three times.
Cell uptake and targeting effects
Cell uptake assays were carried out to determine the active targeting ability of dual-targeted micelles to U87 cells in vitro. Briefly, U87 cells were seeded to 6-well plates at a density of 4.5 × 105 cells/well. After incubation for 24 h, coumarin micelles, BK modified coumarin micelles, MAN modified coumarin micelles, dual-targeted coumarin micelles and free coumarin were separately added to each well, and incubation was continued for 4 h. Culture medium was used as blank control. The cells were harvested and washed with cold PBS three times. The amount of coumarin was measured by the FACScan flow cytometer, and the test was performed in parallel three times.
The BMVEC/U87 co-cultured BBB model is established to evaluate the potential dual-targeting of the dual-targeted artemether plus paclitaxel micelles according to our previous reports [Citation9]. In a nutshell, BMVECs cells were seeded to the top of insert (Corning, NY, USA) at a density of 1.5 × 104 cells and U87 cells were seeded to the lower portion at a density of 16,000 cells per compartment. After culturing for 5 days at 37 °C and 5% CO2, the models were added into the varying formulations, including blank micelles, paclitaxel micelles, artemether plus paclitaxel micelles, BK modified artemether plus paclitaxel micelles, MAN modified artemether plus paclitaxel micelles and the dual-targeted artemether plus paclitaxel micelles. The final concentration of paclitaxel was set to 5 μM. After incubation for 48 h, all the samples were analysed under an inverted microscope (Advanced Microscopy Group, Carlsbad, CA, USA) and the survival of U87 cells was measured by SRB method.
Inhibitory effects on VM channels formation
Matrigel-based experiments were utilised in determining the inhibitory effects on VM channels formation after treatments with varying formulations. Briefly, U87 cells were seeded to 6-well plates at a density of 4.7 × 105 cells/well. When the confluence was about 85%, the varying formulations were added to the wells, including blank micelles, paclitaxel micelles, artemether plus paclitaxel micelles, BK modified artemether plus paclitaxel micelles, MAN modified artemether plus paclitaxel micelles and dual-targeted artemether plus paclitaxel micelles. The final concentration of paclitaxel was 2 μΜ. After the cells were incubated for 6 h, the cells were digested and seeded to 96-well plates with 50 μL of Matrigel previously added. After incubation for another 4 h, the images were acquired with an inverted microscope under random field of view.
Inhibitory effects on the invasion of U87 cells
The transwell assay was used to determine the inhibitory effects on the invasion of U87 cells in vitro after treatments with varying formulations [Citation19]. Briefly, 600 μL of complete DMEM medium was added to the lower layer of the transwell chamber. U87 cells were suspended in serum-free DMEM medium and added to the upper layer of the transwell chamber at a density of 1.3 × 104 cells per well. Then, the varying formulations were separately added to each well with the final concentration of 5 μM paclitaxel. After incubation for about 10 h, the chamber was removed and the non-invasive U87 cells on the upper layer were gently wiped with a cotton ball. The chambers were fixed in 700 μL paraformaldehyde (4%) for 0.5 h and then placed in 700 μL of crystal violet dye solution for 20 min. The number of U87 cells on the lower surface was observed under an inverted microscope in a random field of view.
Wound healing experiments were used to further investigate the anti-invasive effects of the varying formulations. Briefly, U87 cells were seeded in 6-well plates at a density of 4.7 × 105 cells/well. When the cell confluence was about 80%, a 10 μL pipette tip was employed in drawing a straight line through the origin of each well. After washing with PBS buffer to remove the fallen cell debris in the wound, fresh culture medium was added to the 6-well plate, and then the calculated amount of each micelle formulation was added to each well with the final concentration of 5 μM paclitaxel. Blank micelles were as a control. The cell wound images were photographed under an inverted microscope at 0 and 24 h.
Regulation of apoptotic enzyme and invasion related protein in U87 cells
Expression of the apoptotic enzyme (caspase 3) and three invasion related protein (HIF-1α, PI3K and MMP-2) in U87 cells was detected by the enzyme-linked immunosorbent assay kits (Cusabio Biotech Co. Ltd., Beijing, China). In short, U87 cells were cultured in a culture flask with a growth area of 25 cm2 (Corning, NY, USA). When the cell confluence was about 80%, the varying micelle formulations were added to each flask, and the concentration of the paclitaxel was adjusted to 5 μM. After cultivation for 12 h, the cells are obtained and lysed, and the content of the above factors was determined according to the instructions of the kit.
In vivo imaging and anticancer efficacy
The glioma-bearing ICR mice models were established to explore the targeting effects of dual-targeted artemether plus paclitaxel micelles according to previous reports [Citation20]. After transplanting U87 cells for 2 weeks, the model mice were divided into five groups (three mice in each group). The physiological saline, DiR micelles, BK modified DiR micelles, MAN modified DiR micelles and the dual-targeted DiR micelles were injected into the mice through the tail vein. The dose of DiR in each formulation was carried out at 1 mg/kg. The mice were then imaged respectively by a Kodak multimodal imaging system (Carestream Health, Inc., USA) at 1, 4, 8, 12, 24, and 48 h to monitor the distribution of dual-targeted micelles in mice.
After tumour inoculation for 10 days, 45 glioma-bearing mice were randomly divided into five groups. One group of mice was injected with physiological saline as blank control. The other groups of mice were injected with free paclitaxel, artemether plus paclitaxel micelles, BK modified artemether plus paclitaxel micelles, and dual-targeted artemether plus paclitaxel micelles. The dose of paclitaxel in each formulation was carried out at 3 mg/kg and drug injections were performed every two days. On the 17th day after the last injection, three mice were randomly selected and sacrificed in each group, their brains were taken out and frozen sections (each thickness was 10 μm) were made. Nissl staining test was used for measuring the anticancer effects according to our previous reports. The heart, liver, spleen, lungs and kidneys were taken out and embedded in paraffin. After sectioning, H&E staining was performed to observe whether the formulations had any damage to the normal organs. The remaining six mice in each group were used for monitoring survival, and survival time was calculated from day 0 (tumour inoculation) to the day of death. Kaplan-Meier survival curves were plotted for each group.
Statistical analyses
In this study, all data were presented as mean ± standard deviation (SD) and ANOVA was applied to determine the significance among all the groups.
Results
Characterisation of targeting molecules
) shows the MALDI-TOF-MS spectra of COOH-PEG2000-DSPE and the targeting molecule BK-PEG2000-DSPE, respectively. During the synthesis, EDCI and NHS acted as coupling agents to acylate the -NH2 on BK with -COOH on COOH-PEG2000-DSPE to form the targeting compound. It could be known by the MALDI-TOF-MS spectrum that the average mass of [COOH-PEG2000-DSPE]+ was m/z 2854.9, while the average mass of [BK-PEG2000-DSPE + Na]+ was m/z 3856.6 (M + 23). The difference between the two molecules was equal to the mass of BK, indicating the successful synthesis of BK-PEG2000-DSPE.
Figure 1. The characterisations of targeting molecular materials and dual-targeted artemether plus paclitaxel micelles. (A) The MALDI-TOF-MS spectra of COOH-PEG2000-DSPE (A1) and BK-PEG-DSPE (A2); (B) The MALDI-TOF-MS spectra of TPGS1000 (B1), COOH-TPGS1000 and MAN-TPGS1000 (B2); (C) The schematic diagram of dual-targeted artemether plus paclitaxel micelle; (D) TEM images; (E) Size distribution; (F) The in vitro release rates of artemether (F1) and paclitaxel (F2) from micelles in the simulated body fluids. Data are presented as mean ± standard deviation (n = 3).
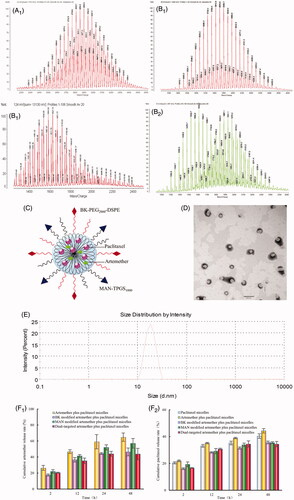
Figure 2. Cytotoxicity of the varing formulation to U87 cells within 48 h. (A) Cytotoxic effects of free drug on U87 cells. (B) Cytotoxic effects of various micelle formulations on U87 cells. p < .05; I, vs. free artemether; II, vs. free paclitaxel; III, vs. 10 μM free artemether plus free paclitaxel; IV, vs. 15 μM free artemether plus free paclitaxel; 1, vs. paclitaxel micelles; 2, vs. artemether plus paclitaxel micelles; 3, vs. BK modified artemether plus paclitaxel micelles; 4, vs. MAN modified artemether plus paclitaxel micelles.
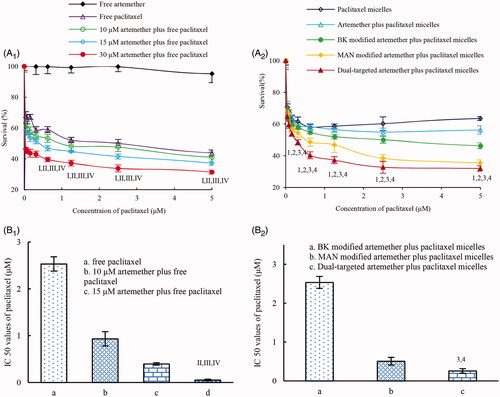
MAN-TPGS1000 was synthesised according to our earlier publication. proclaims the MALDI-TOF-MS spectra of TPGS1000, COOH-TPGS1000 and MAN-TPGS1000. The MALDI-TOF-MS spectrum showed that the average masses of [TPGS1000]+, [MAN-TPGS1000 + Na]+, and [COOH-PGS2000]+ were m/z 1538.7, m/z1931.1 and m/z 1654.7, respectively. The mass difference between MAN-TPGS1000 and TPGS1000 was accurately identical to the mass of MAN after adding mass of glutaric acid while subtracting the mass of 2 mole water, indicating a successful synthesis of the targeting molecule MAN-TPGS1000.
Characterisation of the dual-targeted artemether plus paclitaxel micelles
shows schematic diagrams of dual-targeted artemether plus paclitaxel micelles and the images taken under TEM, respectively. Overall, the micelles were roughly spherical and had a smooth surface. The micelle particles were monodisperse and had a diameter of about 20 nm (). The encapsulation efficiencies of artemether and paclitaxel in different micelles were more than 80% (). ) indicates the release rate of artemether and paclitaxel in the release medium containing 10% FBS. For dual-targeted artemether plus paclitaxel micelles, the release rates of artemether and paclitaxel at 48 h were 43.72 ± 7.14% and 34.40 ± 2.02%, respectively.
Table 2. Characterisation of micelles.
Cytotoxicity of each agent
denotes the cytotoxic effects of free drug on glioma U87 cells. As the results revealed, when artemether alone acted on U87 cells, the toxicity was minimal. While, paclitaxel co-treated with a fixed concentration of artemether exhibited an enhanced cytotoxic effect with dose-dependent. demonstrates the cytotoxic effects of the varying micelles on glioma U87 cells. Results showed that the rank of cytotoxic effects on U87 cells was dual-targeted artemether plus paclitaxel micelles > MAN modified artemether plus paclitaxel micelles > BK modified artemether plus paclitaxel micelles > artemether plus paclitaxel micelles > paclitaxel micelles. The IC50 values were 0.26 ± 0.06 μM for dual-targeted artemether plus paclitaxel micelles, 0.51 ± 0.10 μM for MAN modified artemether plus paclitaxel micelles and 2.53 ± 0.19 μM for BK modified artemether plus paclitaxel micelles, respectively.
Cell cycle arrest
shows the cell cycle arrest of U87 cells caused by the varying micelle formulations. Results showed that paclitaxel micelles resulted in the cell cycle arrest in the G2/M phase. Micelles loaded both artemether and paclitaxel led to the cell cycle arrest in the S phase. Compared with all the other micelle formulations, dual-targeted artemether plus paclitaxel micelles exerted the most obvious increase in the S phase.
Figure 3. The effects of various micellar formulations on inducing apoptosis and stalling cell cycle. (A1) The plots by flow cytometry of cell cycle arrest effects; (A2) The blocking rate of U87 cell cycle induced by various preparations; (B1) Plots by flow cytometry of apoptosis-inducing effects in U87 cells; (B2) Apoptosis inducting effects after treatments with the varying formulations. a. blank micelles; b. paclitaxel micelles; c. artemether plus paclitaxel micelles; d. BK modified artemether plus paclitaxel micelles; e. MAN modified artemether plus paclitaxel micelles; f. dual-targeted artemether plus paclitaxel micelles; p < .05; 1, vs. a; 2, vs. b; 3, vs. c; 4, vs. d. Data are presented as the mean ± standard deviation (n = 3).
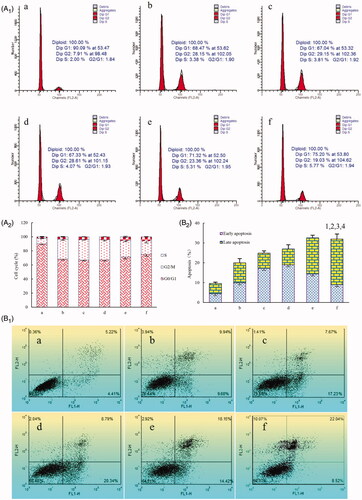
Induction of apoptosis in U87 cells
shows the induction of apoptosis of U87 cells by the varying micelle formulations evaluated by flow cytometry. Apoptosis induction was assessed by the total proportion of early and late apoptotic cells. After addition of the blank micelles, U87 cells showed negligible apoptosis. According to , the total percentage of apoptosis in glioma U87 cells was 9.63 ± 2.23% for blank micelles, 20.11 ± 1.18% for paclitaxel micelles, 24.24 ± 1.73% for artemether plus paclitaxel micelles, 27.22 ± 1.49% for BK modified artemether plus paclitaxel micelles, 31.54 ± 2.11% for MAN modified artemether plus paclitaxel micelles and 32.37 ± 2.45% for dual-targeted artemether plus paclitaxel micelles, respectively.
Cell uptake and targeting effects
depictes the fluorescence intensities and the uptake ratios at 4 h after addition of the varying formulations. Results showed that the rank of the intracellular uptake level was dual-targeted coumarin micelles > MAN modified coumarin micelles > BK modified coumarin micelles > coumarin micelles. The result indicated that the dual-targeted micelles had the best targeting effect on U87 cells. depictes the dual-targeting effects in co-culture model of BMVEC/U87 cells in vitro. After addition of dual-targeted artemether plus paclitaxel micelles, MAN modified artemether plus paclitaxel micelles, BK modified artemether plus paclitaxel micelles, artemether plus paclitaxel micelles, and paclitaxel micelles, the survival rates (%) of U87 cells were 34.70 ± 4.79%, 42.98 ± 3.96%, 48.04 ± 4.88%, 56.91 ± 5.26% and 63.81 ± 6.05%, respectively. Those phenomena indicated that the dual-targeted micelles could transport drugs across the BBB and subsequently target glioma cells, thereby demonstrating forceful inhibition to glioma cells.
Figure 4. The cellular uptake and targeting effects after incubation with the varying formulations. a, coumarin micelles; b, BK modified coumarin micelles; c, MAN modified coumarion micelles; d, dual-targeted coumarin micelles; e, free coumarin; p < .05, 1, vs. a; 2, vs. b; 3, vs. c. Data are presented as mean ± standard deviation (n = 3).
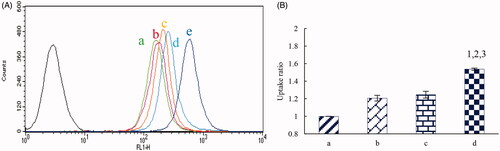
Figure 5. Dual-targeting effect in co-culture model of BMVEC/U87 glioma cells after addition of the varying formulations in vitro. A. Microscopic photographs of dual-targeting effects, B. Survival rates (%) of U87 cells in co-culture model. a. blank micelles; b. paclitaxel micelles; c. artemether plus paclitaxel micelles; d. BK modified artemether plus paclitaxel micelles; e. MAN modified artemether plus paclitaxel micelles; f. dual-targeted artemether plus paclitaxel micelles; p < .05; 1, vs. b; 2, vs. c; 3, vs. d; 4, vs. e. Data are presented as the mean ± standard deviation (n = 3).
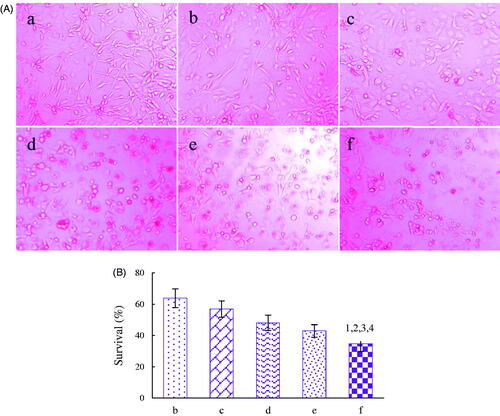
Inhibitory effect on VM channel formation
shows the inhibitory effects on VM channel formation of U87 cells after treatment with the varying micelle formulations. Images showed that the U87 cells treated with blank micelle formed a complete VM channel with a clear channel backbone and a large number of meshes. The VM channels of cells treated with other micelle formulations were more or less damaged. The rank of the inhibitory effects on VM channel formation was blank micelles < paclitaxel micelles < artemether plus paclitaxel micelles ≈ BK modified artemether plus paclitaxel micelles < MAN modified artemether plus paclitaxel micelles < dual-targeted artemether plus paclitaxel micelles.
Figure 6. Inhibitory effects on VM channels, invasion and wound healing of U87 cells. (A) Inhibiting effects on VM channels formation in vitro after treatments with the varying micellar formulations. (B) Blocking invasive effects on U87 cells in vitro after treatments with the varying micellar formulations. (C) Blocking wound healing effects on U87 cells in vitro after treatments with the varying micellar formulations. a. blank micelles, b. paclitaxel micelles; c. artemether plus paclitaxel micelles; d. BK modified artemether plus paclitaxel micelles; e. MAN modified artemether plus paclitaxel micelles; f. dual-targeted artemether plus paclitaxel micelles.
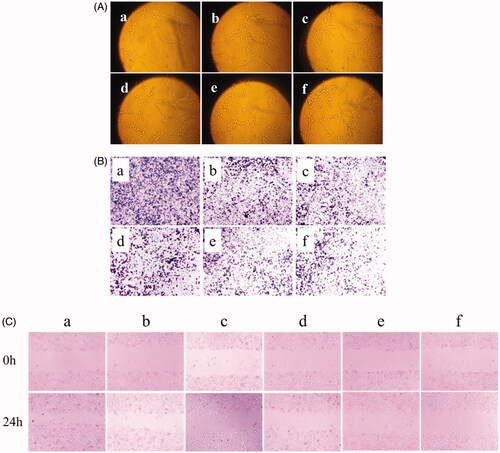
Resistance to invasion of U87 cells
expresses the blocking effect on U87 cell invasion after treatment with the varying micelle formulations. The number of cells that penetrated the lower surface of the transwell chamber was used as the criterion for cell invasion. According to the image, the general trend of the anti-invasive ability of each micelle was as follows: blank micelles < paclitaxel micelles < artemether plus paclitaxel micelles ≈ BK modified artemether plus paclitaxel micelles < MAN modified artemether plus paclitaxel micelles < dual-targeted artemether plus paclitaxel micelles. shows the inhibitory effect on wound healing of U87 cells after treatment with the varying micelle formulations. In all the micelle formulations, dual-targeted artemether plus paclitaxel micelles had the smallest change in width at 24 h compared to the scratch width at 0 h, which indicated that dual-targeted micelles could inhibit the invasion of U87 cells.
Regulation of apoptotic enzyme and invasion related protein in U87 cells
depicts the regulatory effects of the varying micelle formulations on the levels of apoptotic enzymes (caspases-3) and invasion related proteins (HIF-1α, PI3K and MMP-2) in U87 cells. For the three invasion related proteins, dual-targeted artemether plus paclitaxel micelles showed the largest inhibitory effect. After treatments with dual-targeted artemether plus paclitaxel micelles for 12 h, the expression levels of HIF-1α, PI3K and MMP-2 were 0.62 ± 0.15, 0.70 ± 0.11 and 0.61 ± 0.07, respectively. For apoptotic enzymes, all the drug-loaded micelles could increase the expression rate of caspases-3. The expression levels of caspase-3 in U87 cells treated with paclitaxel micelles, artemether plus paclitaxel micelles, BK modified artemether plus paclitaxel micelles, MAN modified artemether plus paclitaxel micelles and dual-targeted artemether plus paclitaxel micelles were 1.20 ± 0.14, 1.42 ± 0.28, 1.81 ± 0.18, 2.06 ± 0.27, 1.96 ± 0.31, respectively.
Figure 7. Regulating effects on invasion related proteins and apoptotic enzyme in U87 cells after treatments with the varying formulations. a. blank micelles, b. paclitaxel micelles; c. artemether plus paclitaxel micelles; d. BK modified artemether plus paclitaxel micelles; e. MAN modified artemether plus paclitaxel micelles; f. dual-targeted artemether plus paclitaxel micelles; p < .05, 1, vs. a; 2, vs. b; 3, vs. c; 4, vs. d.
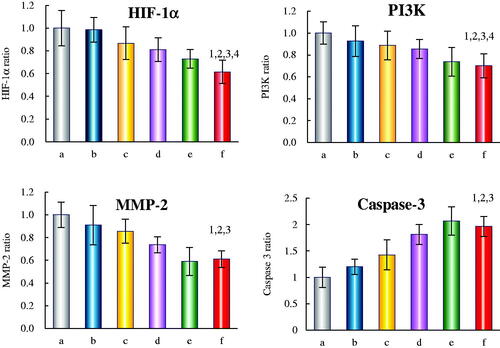
In vivo imaging and anticancer efficacy
shows the distribution of the varying DiR formulations in glioma-bearing mice. From the real-time image, the glioma-bearing mice treated with dual-targeted DiR micelles had the highest fluorescence level and the longest duration in the brain. On the contrary, the fluorescent signal of free DiR was rapidly distributed in the abdominal and rapidly weakened after 24 h. The rank of fluorescence intensity in the tumour site was dual-targeted DiR micelles > MAN modified DiR micelles and BK modified DiR micelles > DiR micelles.
Figure 8. In vivo real-time imaging observation after intravenous administration of the varying formulations. a. blank control. b. DiR micelles. c. BK modified DiR micelles; d. MAN modified DiR micelles; e. dual-targeted DiR micelles.
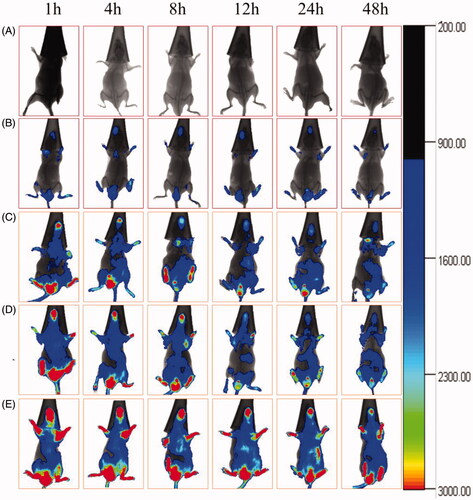
displays the Nissl staining of brain section after treatments with the varying formulations in vivo. Results showed that significant reduction was found in intracranial abnormal cells in glioma-bearing mice after treatments with dual-targeted artemether plus paclitaxel micelles. represents the Kaplan-Meier survival curve after treatments with varying formulations. The median survival time of glioma-bearing mice treated with the dual-targeted artemether plus paclitaxel micelles (30 days) was longer than that of mice treated with physiological saline (18 days, p = .0005), artemether plus paclitaxel (22 days, p = .002), artemether plus paclitaxel micelles (24 days, p = .007), and BK modified artemether plus paclitaxel micelles (26 days, p = .052), respectively. shows a comparison of H&E staining of heart, liver, spleen, lung, and kidney in normal mice and glioma-bearing mice treated with dual-targeted artemether plus paclitaxel micelles. Overall, there was no obvious histopathological abnormalities or lesions between the two groups, which indicated the safety of dual-targeted artemether plus paclitaxel micelles.
Figure 9. Antitumor effects and safety examination in U87 cells xenografts mice after treatments with the varying formulations, with a dose of 3 mg/kg paclitaxel and 6.3 mg/kg artemether at days 11, 13, 15, and 17 from inoculation. (A) Nissl staining; (B) Kaplane Meier survival curves; (C) H&E staining; a. healthy mice; b. xenografts mice with physiological saline; c. xenografts mice with free paclitaxel; d. xenografts mice with artemether plus paclitaxel micelles; e xenografts mice with BK modified artemether plus paclitaxel micelles; f. xenografts mice with dual-targeted artemether plus paclitaxel micelles. Images were obtained under EVOS microscope using a 40× objective.
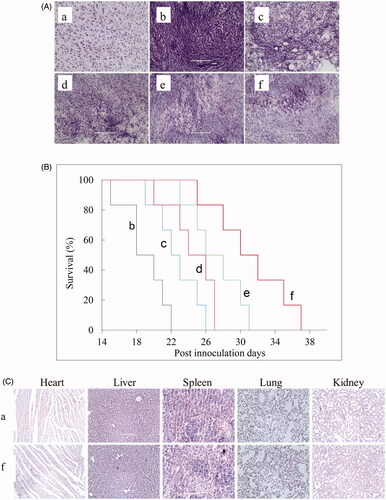
Discussion
Brain gliomas are one of the most harmful tumours in patients. Although modern medicine gradually optimises the treatment of glioma, the curative effect is still unsatisfactory, the entire prognosis is disappointing, and the survival time is short. Chemotherapy for invasive brain glioma is still challenging due to the following reasons. Firstly, surgery cannot clean up all the brain glioma cells for the strong invasion of glioma, thus leading to high recurrence rates [Citation21]. Secondly, BBB is a highly selective physico-biological barrier and most drugs cannot penetrate BBB [Citation22,Citation23], so the chemotherapy effect is unsatisfactory. Thirdly, the non-selective distribution of drugs leads to the obvious toxicity and side effects [Citation24].
In this study, the dual-targeted artemether plus paclitaxel micelles was successfully prepared to resolve the above questions. It could be seen from that the targeting molecules of BK-PEG2000-DSPE and MAN-TPGS1000 were smoothly synthesised, and shows that both artemether and paclitaxel were efficiently encapsulated in the micellar core. Dual-targeted artemether plus paclitaxel micelles had exceptional release behaviour in the simulated body fluids () and this was beneficial for keeping stable in the process of drug delivery and increasing the active targeting to glioma cells. Those characters indicated that the micelle had been very likely to cross the BBB and enrich the drug in the glioma site.
In cytotoxic assays of free drugs, artemether had little cytotoxicity on U87 cells in the range of experimental concentration. However, it significantly increased the cytotoxic and apoptotic activity of paclitaxel against U87 cells. In cytotoxic assays of micellar formulations, with the mighty assistance of BK-PEG2000-DSPE and MAN-TPGS1000, dual-targeted artemether plus paclitaxel micelles showed the strongest growth inhibition and apoptosis-inducing effects on U87 cells ( and ). Further action mechanism showed that dual-targeted artemether plus paclitaxel micelles completely increased the expression level of caspase-3 and induced apoptosis of U87 cells to some extent () [Citation25–27].
Active targeting effects of the drug-loaded micelles in vitro were evaluated by intracellular uptake and co-culture BBB model in our study. Results indicated that dual-targeted artemether plus paclitaxel micelles could obviously enhance intracellular uptake in U87 cells. The enhanced intracellular uptake was related to the addition of BK-PEG2000-DSPE and MAN-TPGS1000 in the preparation of dual-targeted micelles. It was reported that bradykinin b2 receptor was overexpressed on the surface of glioma cells, and BK could enhance adhesion of drug delivery system to endothelial cells and increase BBB permeability via activation of bradykinin b2 receptor. In addition, the level of glucose uptake and glucose metabolism in tumour cells was higher than normal cells and GLUT was acted as an important pathway for tumour cells to transport and metabolise glucose [Citation28]. Our previous reports had showed that MAN-TPGS1000 could transport drugs across BBB via targeting GLUT. In co-culture BBB model, TEER values were measured in the process of experiments to make sure the accuracy of the BBB model. Results indicated dual-targeted artemether plus paclitaxel micelles exhibited the strongest transporting ability across the BBB ( and ).
The invasiveness of glioma cells is one of the important reasons for the difficulty in curing glioma [Citation29]. VM channels are non-endothelial “vascular-like” structure in vascular-dependent solid tumours, and composed of tumour cells with endothelial function [Citation30]. It is a special blood supply pattern for tumours in highly invasive malignant tumours, such as melanoma, glioma and small cell lung cancer. Because of the absence of vascular endothelial barrier, VM is more conducive to the invasion and metastasis of tumour cells. In the invasive process of glioma cells into normal tissues, the extracellular matrix of the tumour cells is first degraded, thereby losing the connection with the basement membrane and entering the microvessels. After the new VM channels are generated, the tumour cells continue to invasive the surrounding normal cells [Citation31,Citation32]. To evaluate the inhibitory effects on VM channels formation, the Matrigel-based model was formed using glioma U87 cells. Results showed that dual-targeted artemether plus paclitaxel micelles could significantly inhibit the VM channel formation, thereby blocking the blood supply to the tumour area and inhibiting tumour cell invasion (). At the same time, the anti-invasive ability of dual-targeted artemether plus paclitaxel micelles was also confirmed by transwell test and wound healing test in this study ().
Hypoxic microenvironment is an important feature of solid tumours. HIF-1α is a key regulator in hypoxia [Citation33], it can promote tumour cells to better adapt to hypoxia and enhance their own survival [Citation34–36]. Matrix metalloproteinases (MMPs) is a group of endopeptidases that can degrade extracellular matrix protein in tumour microenvironment [Citation37], thereby causing tumour cells to lose their connection to the basement membrane [Citation38]. The PI3K/Akt/m TOR signalling pathway is a very important pathway in the cell signalling pathway [Citation39,Citation40]. PI3K signalling disorders are closely related to the occurrence and development of malignant tumours, and affect the formation of VM channels [Citation41]. In this study, dual-targeted artemether plus paclitaxel micelles significantly reduced the synthesis of invasion related proteins (HIF-1α, MMP-2, PI3K) in U87 cells (). The down-regulation of these proteins may be the action mechanism for destroying VM channels and inhibiting U87 cells invasion.
The targeting effect of dual-targeted micelles in vivo was testified in glioma-bearing ICR mice. The DiR dye was used as a fat-soluble fluorescent probe to indicate the distribution of micelles. Results showed that dual-targeted DiR micelles had a long-lasting stability in the blood circulation, and displayed a higher accumulation in the brain tumour region (). The obvious distribution in tumour masses were related to the increasing drug transport ability across BBB and the enhancing intracellular uptake by glioma U87 cells. Anticancer efficacy was assessed on brain glioma-bearing mice from the survival time and the Nissl staining of pathological brains. After treatment with dual-targeted artemether plus paclitaxel micelles, the survival time of the xenograft mice was significantly prolonged, and the number of the glioma cells was significantly reduced. Meanwhile, no toxic reaction to normal tissues was observed by H&E staining ().
Conclusion
In this study, the dual-targeted micelles are produced by thin-film method. The enhanced anticancer efficacy in treating glioma-bearing mice could be explained by the following aspects: (i) the combinational use of artemether and paclitaxel is able to improve the cytotoxic effects. (ii) the modifications of BK-PEG2000-DSPE and MAN-TPGS1000 on the surface of micelles enhance the transporting ability across the BBB and exhibit obvious targeting effect to invasive brain glioma cells. (iii) the addition of artemether inhibits the VM channels formation and the invasion of glioma cells. (iv) dual-targeted artemether plus paclitaxel micelles down-regulates the expression of HIF-1α, MMP-2 and PI3K. Thereby, dual-targeted artemether plus paclitaxel micelles offer an opportunity for the potential clinical treatment of brain glioma.
Disclosure statement
No potential conflict of interest was reported by the author(s).
Additional information
Funding
References
- Venkatesh HS, Tam LT, Woo PJ, et al. Targeting neuronal activity-regulated neuroligin-3 dependency in high-grade glioma. Nature. 2017;549(7673):533–537.
- Hochberg FH, Atai NA, Gonda D, et al. Glioma diagnostics and biomarkers: an ongoing challenge in the field of medicine and science. Expert Rev Mol Diagn. 2014;14(4):439–452.
- Jaimes C, Poussaint TY. Primary neoplasms of the pediatric brain. Radiol Clin North Am. 2019;57(6):1163–1175.
- Lulla RR, Saratsis AM, Hashizume R. Mutations in chromatin machinery and pediatric high-grade glioma. Sci Adv. 2016;2(3):e1501354
- Mackay A, Burford A, Molinari V, et al. Molecular, pathological, radiological, and immune profiling of non-brainstem pediatric high-grade glioma from the HERBY phase II randomized trial. Cancer Cell. 2018;33(5):829–842. e825,
- Fan K, Jia X, Zhou M, et al. Ferritin nanocarrier traverses the blood brain barrier and kills glioma. ACS Nano. 2018;12(5):4105–4115.
- Tang W, Fan W, Lau J, et al. Emerging blood-brain-barrier-crossing nanotechnology for brain cancer theranostics. Chem Soc Rev. 2019;48(11):2967–3014.
- Watkins S, Robel S, Kimbrough IF, et al. Disruption of astrocyte-vascular coupling and the blood-brain barrier by invading glioma cells. Nat Commun. 2014;5:4196
- Li X-Y, Zhao Y, Sun M-G, et al. Multifunctional liposomes loaded with paclitaxel and artemether for treatment of invasive brain glioma. Biomaterials. 2014;35(21):5591–5604.
- Feng X, Yao J, Gao X, et al. Multi-targeting peptide-functionalized nanoparticles recognized vasculogenic mimicry, tumor neovasculature, and glioma cells for enhanced anti-glioma therapy. ACS Appl Mater Interfaces. 2015;7(50):27885–27899.
- Liu Y, Mei L, Yu Q, et al. Multifunctional tandem peptide modified paclitaxel-loaded liposomes for the treatment of vasculogenic mimicry and cancer stem cells in malignant glioma. ACS Appl Mater Interfaces. 2015;7(30):16792–16801.
- Nance E, Zhang C, Shih T-Y, et al. Brain-penetrating nanoparticles improve paclitaxel efficacy in malignant glioma following local administration. ACS Nano. 2014;8(10):10655–10664.
- Sofias AM, Dunne M, Storm G, et al. The battle of “nano” paclitaxel. Adv Drug Deliv Rev. 2017;122:20–30.
- Efferth T. From ancient herb to modern drug: artemisia annua and artemisinin for cancer therapy. Semin Cancer Biol. 2017;46:65–83.
- Marcos-Contreras OA, Martinez de Lizarrondo S, Bardou I, et al. Hyperfibrinolysis increases blood-brain barrier permeability by a plasmin- and bradykinin-dependent mechanism. Blood. 2016;128(20):2423–2434.
- Nomura N, Verdon G, Kang HJ, et al. Structure and mechanism of the mammalian fructose transporter GLUT5. Nature. 2015;526(7573):397–401.
- Ju R-J, Mu L-M, Li X-T, et al. Development of functional docetaxel nanomicelles for treatment of brain glioma. Artif Cells Nanomed Biotechnol. 2018;46(sup1):1180–1190.
- Wang X, Cheng L, Xie H-J, et al. Functional paclitaxel plus honokiol micelles destroying tumour metastasis in treatment of non-small-cell lung cancer. Artif Cells Nanomed Biotechnol. 2018;46(sup2):1154–1169.
- Li X-T, Tang W, Xie H-J, et al. The efficacy of RGD modified liposomes loaded with vinorelbine plus tetrandrine in treating resistant brain glioma. J Liposome Res. 2019;29(1):21–34.
- Liu J-J, Tang W, Fu M, et al. Development of R8 modified epirubicin-dihydroartemisinin liposomes for treatment of non-small-cell lung cancer. Artif Cells Nanomed Biotechnol. 2019;47(1):1947–1960.
- Giese A, Bjerkvig R, Berens ME, et al. Cost of migration: invasion of malignant gliomas and implications for treatment. J Clin Oncol. 2003;21(8):1624–1636.
- van Tellingen O, Yetkin-Arik B, de Gooijer MC, et al. Overcoming the blood-brain tumor barrier for effective glioblastoma treatment. Drug Resist Updat. 2015;19:1–12.
- Liebner S, Dijkhuizen RM, Reiss Y, et al. Functional morphology of the blood-brain barrier in health and disease. Acta Neuropathol. 2018;135(3):311–336.
- Zhou W, Chen C, Shi Y, et al. Targeting glioma stem cell-derived pericytes disrupts the blood-tumor barrier and improves chemotherapeutic efficacy. Cell Stem Cell. 2017;21(5):591–603. e594.
- Shen X, Burguillos MA, Osman AM, et al. Glioma-induced inhibition of caspase-3 in microglia promotes a tumor-supportive phenotype. Nat Immunol. 2016;17(11):1282–1290.
- Rogers C, Erkes DA, Nardone A, et al. Gasdermin pores permeabilize mitochondria to augment caspase-3 activation during apoptosis and inflammasome activation. Nat Commun. 2019;10(1):1689
- Giampazolias E, Zunino B, Dhayade S, et al. Mitochondrial permeabilization engages NF-κB-dependent anti-tumour activity under caspase deficiency. Nat Cell Biol. 2017;19(9):1116–1129.
- Nagarajan A, Dogra SK, Sun L, et al. Paraoxonase 2 facilitates pancreatic cancer growth and metastasis by stimulating GLUT1-mediated glucose transport. Mol Cell. 2017;67(4):685–701. e686.
- Zhang L, Wang J, Fu Z, et al. Sevoflurane suppresses migration and invasion of glioma cells by regulating miR-146b-5p and MMP16. Artif Cells Nanomed Biotechnol. 2019;47(1):3306–3314.
- Talasila KM, Soentgerath A, Euskirchen P, et al. EGFR wild-type amplification and activation promote invasion and development of glioblastoma independent of angiogenesis. Acta Neuropathol. 2013;125(5):683–698.
- Depner C, Zum Buttel H, Böğürcü N, et al. EphrinB2 repression through ZEB2 mediates tumour invasion and anti-angiogenic resistance. Nat Commun. 2016;7:12329.
- Hamidi H, Ivaska J. Every step of the way: integrins in cancer progression and metastasis. Nat Rev Cancer. 2018;18(9):533–548.
- LaGory EL, Giaccia AJ. The ever-expanding role of HIF in tumour and stromal biology. Nat Cell Biol. 2016;18(4):356–365.
- Kim Y, Nam HJ, Lee J, et al. Methylation-dependent regulation of HIF-1α stability restricts retinal and tumour angiogenesis. Nat Commun. 2016;7:10347.
- Du R, Lu KV, Petritsch C, et al. HIF1alpha induces the recruitment of bone marrow-derived vascular modulatory cells to regulate tumor angiogenesis and invasion. Cancer Cell. 2008;13(3):206–220.
- Kim Y-J, Lee H-J, Kim T-M, et al. Overcoming evasive resistance from vascular endothelial growth factor a inhibition in sarcomas by genetic or pharmacologic targeting of hypoxia-inducible factor 1α. Int J Cancer. 2013;132(1):29–41.
- Qin C, He B, Dai W, et al. The impact of a chlorotoxin-modified liposome system on receptor MMP-2 and the receptor-associated protein ClC-3. Biomaterials. 2014;35(22):5908–5920.
- Goncalves-Ribeiro S, Sanz-Pamplona R, Vidal A, et al. Prediction of pathological response to neoadjuvant treatment in rectal cancer with a two-protein immunohistochemical score derived from stromal gene-profiling. Ann Oncol. 2017;28:2160–2168.
- Andeol Y, Bonneau J, Gagné LM, et al. The phosphoinositide 3-kinase pathway and glycogen synthase kinase-3 positively regulate the activity of metal-responsive transcription factor-1 in response to zinc ions. Biochem Cell Biol. 2018;96(6):726–733.
- Hanker AB, Kaklamani V, Arteaga CL. Challenges for the clinical development of PI3K inhibitors: strategies to improve their impact in solid tumors. Cancer Discov. 2019;9(4):482–491.
- Jain P, Surrey LF, Straka J, et al. Novel FGFR2-INA fusion identified in two low-grade mixed neuronal-glial tumors drives oncogenesis via MAPK and PI3K/mTOR pathway activation. Acta Neuropathol. 2018;136(1):167–169.