Abstract
In this paper, we developed a novel strategy of preparing doxorubicin (DOX) nanocrystal (NC) exerting spherical particles with a diameter of 102 nm, which experienced following coating of chondroitin sulphate derivative (CSOA) shell via electrostatic and hydrophobic interactions. Such multifunctional outerwear resulted in drug nanocapsules with high drug loading content up to 70% and high colloidal stability under physiological conditions. It exhibited accelerated drug release behaviour when dispersing in hyaluronidase (HAase) containing medium or incubated with cancer cells. CSOA/NCs were effectively taken up by cancer cells via CD44 receptor-mediated endocytosis, but were rarely internalised into normal fibroblasts. With the comparison of typical drug-loaded micelles system (DOX/PEG-PCL), CSOA/NCs showed greater inhibition to cancer cells due to the targeted and sensitive drug delivery.
Introduction
Chemotherapy is the most diffusely approach for killing cancer cells rapidly and inhibiting cancer cells proliferation. Whereas many anticancer drugs have extremely solubility in aqueous, which limit their clinical application badly. To settle the obstacles such as the poor stability, bioavailability, severe toxicity and non-targeted circulation of anticancer drugs, nanoparticulate drug delivery systems (NDDSs) have developed and revealed great potential in tumour chemotherapy [Citation1]. Such NDDSs including dendritic polymers, micelles, liposomes and so on assembly are thus developed as carriers of drugs. Despite the notable merits of these representative vehicles, there are still two obvious inconsistencies. One is the opposite between high drug loading and high stability, the other is burdensome preparation and possible toxicity or biodegradation problem of man-made multifunctional polymer.
For instance, amphiphilic block copolymers are typically inert to form the core-shell like structure encapsulating hydrophobic drugs. These vesicles, which have no other functions but to make the vesicles possess carry, are usually the major component with weight far bigger than cargos. Thus, it decreased the drug loading capacity of carriers and brought about hidden trouble for metabolism, which would impose an extra burden for patients. Lots of works have hammered at increasing the interactions between drugs and carriers for ensuring the stability, but their payload releasing in response to stimuli needed more attention. Recently, polymeric−drug conjugates are creating new opportunities for improved cancer therapy [Citation2]. The assembly which consisted of small molecule drug/drugs and large size of polymer molecules, affords the vesicles with high drug loading content and the drug with a large hydrodynamic volume [Citation3]. The linkers between them have to be split at tumour environment, and then the drugs were subsequently released. However, promising the pharmacological activity of drugs needed to be considered as the precondition, which required rationally chemical synthesis and modification. In other words, prodrug design might bring risk to the pharmacology and not many drugs were suitable to experience that kind of strict preparation at the present stage.
A new and general strategy was reported, producing drug nanocrystalline with followed surface modification to achieve multifunction. It needs reasonable cooperation of nanoscale crystals formation and layer coating technology. Among these methods for promoting bioavailability and controlled release of anticancer drugs, there are limited successes but most of them are in early stages of development. It remains a few challenges to their bio-applications. Generally, molecule components are easily locked into kinetically stable arrangements of varying topology [Citation4]. The formation stage, of which the small seeds are grown into larger particles, requires rigorous and mild conditions for desirable size and shape. Moreover, most of the works on layer coating were applied to micro-cores without sufficient attention to drug capsules with a nanoscale (about 100 nm) [Citation5], which would improve their passive targeting via EPR effect. Surface PEGylation is usually relied on, and the colloidal stability under physiological conditions needed scrupulous study. Finally, in other to enhance the anticancer efficacy, the nanomedicine system should be equipped multiple functions, including active targeting to the receptors uniquely expressed on some cell surfaces for efficient uptake [Citation6]. and rapidly stimuli-responsive drug release for selective drug deliver and reducing drug resistance in cancer cells [Citation7].
Doxorubicin (DOX) is a broad-spectrum antitumor drug. Unfortunately, its effectiveness is not satisfactory by its short half-life in the blood circulation and insecurity to normal tissues [Citation8]. For clinical applications, repeated administrations of high dose of DOX would cause fearful systemic toxicity. While there is a need to explore an efficient way to increase anticancer activity and decrease the toxicity for DOX deliver, we were interested in developing a DOX nanocrystal (NC) system equipping multifunctional coating outerwear. By the way, because of the water-solubility of DOX·HCL, the DOX NC has not been reported for delivery research. Meanwhile, our study found that DOX NC exhibited wealthily positive character on the surface, which was different from that of another drug NC [Citation9]. Therefore, we planned to design a polyanion derivative shell for DOX NC with the driven force of electrostatic and hydrophobic interactions.
Chondroitin sulphate (CS), an anionic and physiologically specific glycosaminoglycan (GAG), has been used as a potential material in bio-applications for a long time. [Citation10] CS is the most abundant GAG in the human body that was found in extracellular matrix, corneas, skin and cartilage CS signifying its excellent biocompatibility. More importantly, CS could be internalised in cells similarly to hyaluronic acid (HA) via CD44 receptor-mediated endocytosis [Citation11]. CD44 is overexpressed in lots of solid tumour cells, which endowed an effective tumour homing property to CS system. Meanwhile, hyaluronidase (HAase), whose levels are known to increase in kinds of malignant tumours, possesses broad substrate specificity to rapidly cleave most β-1,4-N-acetylgalactosaminidic linkages bound to glucuronic acid (GlcA) in acid environment including CS, leading to readily degradation of it into low molecular weight fragments [Citation12]. HA derivatives have been widely developed as drug carriers for targeted and sensitive delivery, but minority of that for CS. By the way, CS with many sulphate groups furnishes a higher hydrodynamic volume than HA, which may avoid undesirable adsorption during circulation in the blood. Therefore, we aspired to prepare a CS derivative for surface modification of DOX NC.
Here, we elaborated the strategy of designing an architectural shell on DOX NC via electrostatic and hydrophobic interactions. The eager size of DOX NC and multifunctional outerwear were obtained, building a “smart” NDDS, of which we definitely researched the self-assembly behaviour, size and morphology, stability, sensitive antitumor drug release performance, targeted cellular uptake and in vitro antitumor activity.
Materials and methods
Materials
1-Ethyl-3-(3-dimethyllaminopropyl) carbodiimide hydrochloride (EDCI), N-Hydroxysuccinimide (NHS) and triethylamine (TEA) were purchased from GL Biochem. Ltd. Oleic acid (OA), N, N-Dicyclohexylcarbodiimide (DCC), 4-dimethylaminopyridine (DMAP), a-methoxy-amino-u-poly (ethylene glycol) (PEG, Mw = 2000 g/mol), dimethyl sulfoxide-d6 (DMSO-d6), deuteroxide (D2O) and hyaluronidase (HAase, from bovine testes, 400-1000 units/mg) were purchased from Sigma-Aldrich Co. and used as received. Doxorubicin hydrochloride (DOX·HCl) was provided by Shanghai Yingxuan Chempharm Co.Ltd. Chondroitin 4-sulphate sodium salt was obtained from Chengdu best-reagent Co. All the solvents were purchased from Chengdu Kelong Chemical Co. and purified before used. Dulbecco’s Modified Eagle’s Medium (DMEM) medium, foetal bovine serum (FBS), penicillin-streptomycin, trypsin, cell counting kit-8 (CCK-8) and 40,6-diamidino-2-phenylindole (DAPI) were purchased from HyClone Inc. and used for cells test.
Characterizations
The size distribution and ζ-potential of all the particles were measured by DLS (Malvern ZetasizerNano ZS). For SEM images (Hitachi Ltd., S4800), all the samples were prepared by dropping the fresh solution onto the silicon slice and dried overnight at room temperature. Some of these samples were coated with conductive layers of gold (Au) for 10 s, resulting in an approximately 2 nm thick coating. Samples of TEM (FEI Tecnai G2 F20 S-TWIN) and AFM (Digital Instruments, Inc., MFP-3D-Bio) measurements were prepared using the similar way with SEM test, except replacing silicon slice with copper grids with Formvar films and mica sheet, respectively. UV-Vis absorption (Specord 200 PLUS) and fluorescence spectra were used to exam the photometric properties of the DOX NCs and CSOA/NCs.
Synthesis of PEGOA
mPEG (0.34 g, 0.17 mmol), DMAP (0.02 g, 0.18 mmol) and oleic acid (OA, 0.2 g, 0.71 mmol) were dissolved in 5 ml CH2Cl2 in an ice bath under nitrogen atmosphere. A solution of DCC (0.70 g, 3.39 mmol) in 5 ml CH2Cl2 was added dropwise into the mixture. The mixture was stirred for 48 h at room temperature. The white DCU precipitate was removed by filtration, the filtrate was concentrated. The crude product was isolated by precipitation in cold diethylether and then recrystallization in ethanol. The crystal was dissolved in deionised water and dialysed for three days and then freeze-dried to receive PEGOA.
Synthesis of CSOA
CS (0.2 g, 0.035 mmol units) was dissolved in 1 ml deionised water to obtain polysaccharide solution. EDCI (0.2 g, 0.1 mmol), NHS (0.04 g, 0.035 mmol) and OA (0.1 g, 0.035 mmol) were mixed together in 15 ml DMSO for 1 h to activate the carboxyl groups of OA. Then, the mixed solution was added into the CS solution in an ice bath. The reaction was allowed to proceed for 2 days at room temperature. The resulting mixture was dropped into ethanol with vigorous stirring and the precipitation was collected by centrifugation (4000 rpm, 5 min). This crude product was purified by dialysis to remove unreacted agents. Finally, the CSOA was received by lyophilised. The degree of substitution (DS) of CSOA which is defined as the number of OA per 100 sugar residues of CS was estimated using 1H NMR analysis.
Preparation and surface modification of DOX NCs
The preparation of DOX NCs contained two main process including neutralization of DOX and crystal formation via solvent exchange method [Citation13]. Firstly, 0.3 ml of 5 mM doxorubicin hydrochloride/methanol solution was mixed with 0.3 ml of 15 mM TEA/methanol solution under vigorous stirring for 3 h. Then 0.4 ml of DMSO was added into the mixture to proceed for 2 days for the preparation of 1.5 mM DOX solution. Secondly, 0.3 ml of DOX mother solution was injected into 5 ml of water solution at 60°C under vigorous stirring. After mixing for 5 min, the sample was stabilised for 18 h. A certain amount of PEGOA/H2O, CS/H2O and CSOA/H2O solution was added into the DOX NCs, then the mixture was subjected to ultrasonic treatment for 5 min and stored overnight to receive PEGOA/NCs, CS/NCs and CSOA/NCs.
Drug release test
In dialysis method, CSOA/NCs were dispersed in PBS (1 ml, 0.01 M, pH 7.4), ABS (1 ml, 0.01 M, pH 5.0) and ABS with HAase (100 unit/mL), respectively. The mixture was transferred into dialysis membrane tubing (Spectra/Por MWCO = 2000). The tubings were immersed in vials containing 30 ml corresponding medium, respectively. The volume of solution was kept constant by adding medium after each sampling at prescribed time intervals. The released DOX was detected by a fluorescence detector (λex=485 nm and λem=550 nm). The release experiments were conducted in triplicate in sink condition, the mean value was presented.
To estimate DOX release of CSOA/NCs and typical DOX-loaded micelles (PEG-PCL/DOX) in different cells, we detected fluorescence intensities of DOX at predetermined time points after incubation with normal cells (NIH 3T3) or cancer cells (Hela, HepG2). The resulted plates were evaluated by optical imaging technique (Leica TCS SP5) to investigate the fluorescence changes.
Cell viability measurement
The cells (6 × 104 cells/mL) were seeded in 96-well plates with 100 μL mediums for 24 h incubation before the tests. CSOA, PEG-PCL, CSOA/NCs and PEG-PCL/DOX in culture mediums were added to the medium-removed 96-well plates with different concentrations and incubated for 48 h. The culture medium was removed and the wells were rinsed with PBS (pH 7.4). CCK-8 in DMEM (10%) was added to each well. After the cells were incubated for additional 2 h the absorbance was measured in a Thermo scientific MK3 (λ = 450 nm).
The cells (7 × 104 cells/mL) were seeded in 96-well plates with 100 μL mediums for 24 h incubation before the tests. DOX·HCl, blank materials and DOX-loaded system in culture mediums were added to the medium-removed 96-well plates with different concentrations and incubated for 48 h. The culture medium was removed and the wells were rinsed with PBS (pH 7.4). CCK-8 dilution in DMEM (0.1 ml/mL) was added to each well. After the cells were incubated for additional 2 h, the absorbance of each well was measured in a Thermo scientific MK3.
Cellular uptake
In order to investigate whether the uptake of CSOA/NCs was CS receptor-mediated, we carried out competitive inhibition studies using both confocal laser scanning microscopy (CLSM) and flow cytometry. For CLSM studies, HepG2 and NIH 3T3 cells were seeded on (diameter = 35 mm) glass dishes at a cell density of 1 × 104 ml−1, respectively. After incubated for 24 h, cancer cells were pre-treated with free CS (5 mg/mL, pH 7.4) for 2 h, washed, and treated with CSOA/NCs (DMEM, DOX concentration was 10 μg/mL) 4 h at 37 °C. Then the medium was removed and the dishes were rinsed with PBS (pH 7.4). The cell nucleus was stained with DAPI and the culture medium was replaced with PBS. The cells were observed with confocal laser scanning microscopy (CLSM, Leica TCP SP5). DOX was excited at 488 nm with the emission at 590 nm.
For flowcytometry study, HepG2 cells were seeded on 6-well plates at a density of 2 × 105 cells/well and cultured for 24 h. Processing method as above and the cells were resuspended in PBS after centrifugation (1000 rpm/min, 5 min) and measured for the fluorescence intensity on a BD FACS Calibur flow cytometer (Beckton Dickinson).
Results and discussion
The DOX NCs were prepared by a solvent exchange method shown in Scheme 1. As we know, water-soluble hydrochloride of DOX (DOX·HCl) is a broad-spectrum antitumor drug with serious systematic toxicity [Citation14]. Solvent exchange method, which used to prepare nanocrystal, nanorod and nanowire in aqueous solution [Citation15], did require the small molecular for high hydrophobicity and aromaticity in the process of crystal growth. Therefore, the neutralization of DOX in organic solvents plays a key role in nanocrystal formation. At the same time, DOX was known to form a dimer in an aqueous medium due to π–π stacking of its planar aromatic ring, increasing susceptibility and complicacy to DOX for hydrophobization. That might be the reason why scientists did not choose DOX for nanocrystal study in drug delivery system. As expected, unbefitting solvent and dispersion method, ungenerous neutralization and growth time, made the disappointed particles size (Figure S1). After a series of adjustment, DOX NCs with expected nanoscale and narrow size dispersion were obtained in the light of enhancing bioavailability and more effective EPR effect (10–200 nm). exhibited a typical SEM image, which were monodisperse and exhibited spherical shape. showed that the DOX NCs had an average diameter of about 102 nm with a narrow size distribution (PDI = 0.083). AFM 3 D and cross-sectional analysis also confirmed the morphological properties of DOX NCs. Combining with these results and other analysis ( for TEM, for confocal fluorescence images), we ensured the firstly successful preparation of DOX NCs for as-bioapplication.
Scheme 1. An illustration of targeted DOX delivery system by surface modification on doxorubicin nanocrystals.
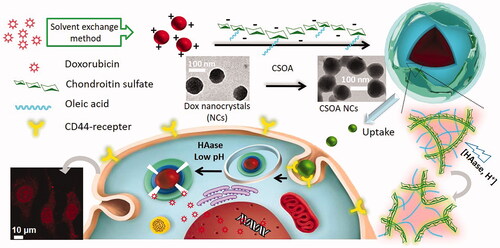
Figure 1. (a) DLS measurement, (b) SEM images and (c) AFM images of DOX NCs. Among them, the inserted graph of (b) was the NCs samples with a 2 nm layer of Au, and the AFM study contained 3D image, height image and cross-sectional analysis.
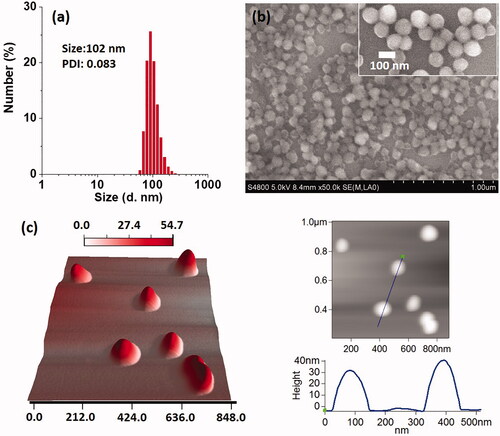
Figure 3. (a) The cummulated DOX release profiles using dialysis method of CSOA/NCs in different medium at 37˚C. Means ± SD (n = 3). (b) Fluorescent quantification of PEG-PCL/DOX and CSOA/NCs, and the optical images of CSOA/NCs after incubation with normal and cancer cells at different time.
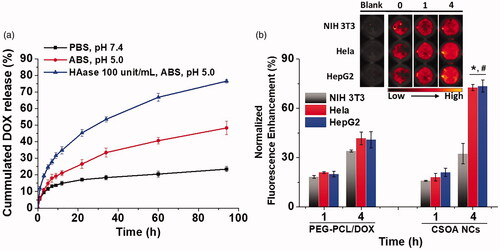
We also used UV-vis and FTIR spectrum to monitor the chemical structure and characteristic absorption after NCs formation. Slightly changes were found in both of the measurements. In the free molecular state, DOX·HCl displayed significant absorption λmax at 488 nm. Upon NCs formation in water, the absorption peak red-shifted to 512 nm (). This bathochromic absorption shift is a typical indication for the J-aggregation of chromophores, indicating the contribution of π–π interaction to crystal growth. After the dissolution of NCs in DMSO, the absorption λmax turned back to 488 nm. These studies showed that the chemical structure and activity did not change in the process of NCs formation. The FTIR spectra of DOX (Figure S2) indicated that the peak assigned to the skeletal vibration of aromatic C = C bonds (1575 cm−1) was greatly decreased after the aggregation in water, that might be attributed to the influence of π-π interaction between benzene rings [Citation16].
Figure 4. (a) UV-vis absorption of DOX•HCl and DOX NCs in different solvents. (b) The mean diameter and count rate change (form DLS measurement) of DOX NCs in water or physiological saline. The inserted photos (b, left) was the DOX NCs in aqueous solution with Tyndall effect and in physiological saline after being left for 12 h.
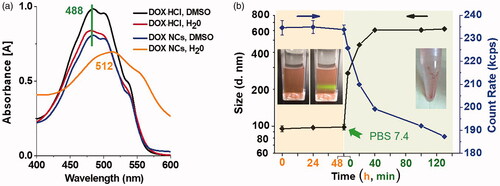
In , the NCs did not exhibit distinct changes in both size and count rate for 48 h standing in aqueous solution. The colloid solution was homogeneous and exhibited bright Tyndall effect. In addition, we examined the surface charge of the NCs (ζ-potential), which was 29.77 ± 0.49 mV (). However, after dispersion in PBS (7.4, 0.01 mM), the original DOX NCs would quickly grow into large aggregations and suffered from significant count rate decrease, due to elimination of the surface charge of DOX NCs by zwitterions in physiological saline. Finally, almost all of DOX precipitated out of water. That is to say, surface functionalization of the nanocrystals is essential to achieve excellent water dispersibility as well as good potential for drug delivery.
Figure 5. The (a) size distribution, (b) mean diameter and PDI, (c) zeta potential, of DOX NCs and modified DOX NCs in different mediums from DLS measurement. (d) The size change of modified DOX NCs in PBS. The inserted photos were the samples in PBS after being left for 12 h. Means ± SD (n = 3).
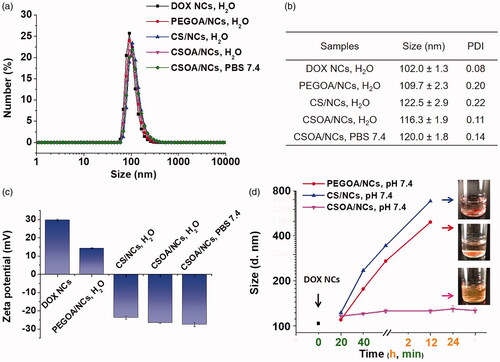
In the beginning of DOX NCs surface modification, PEGOA was prepared as an amphipathic surfactant to cover the nanoparticles via hydrophobic interactions to suppress non-specific adsorption of impurities and provide excellent stability in high salt concentrations. However, it is different from other papers that [Citation17], the coating of PEGOA did not work. Further considering the highly positive charged surface of DOX NCs, for targeted and environment-sensitive drug delivery, we chose CS as a template to develop a surfactant equipping amphipath, electronic potential and multifunction.
The synthesis procedure of PEGOA and CSOA was accomplished according to the esterification illustrated in and Figure S3. First, PEGOA and CSOA were prepared via DCC/EDC-mediated condensation reaction, respectively, and characterised by 1H NMR. The signals for the aliphatic chain of OA (1.24 ppm), polyethylene glycol (3.4–3.7 ppm), methylene and hydroxyl groups at the sugar unit (3.3–4.0 ppm) of CS, were confirmed in 1H NMR spectra, indicating successful synthesis of PEGOA and CSOA. The MALDI-TOF spectra of PEGOA (Figure S3c) show a bell-shaped distribution with a 265 (m/z) increase, indicating the completely coupling of OA to PEG. FTIR were also demonstrated the preparation of CSOA conjugates, as shown in . Compare with pure CS, a shoulder peak occurred at 1702 cm-1 for the carbonyl of ester bond was observed in the spectrum of CSOA, indicating that –COOH was introduced to OA. After calculation to , the percentage of OA grafted onto CS was estimated to be 7.2%. The negative charged CS chain was connected with hydrophobic and flexible OA, that could improve the integration between the coating and DOX NCs by both hydrophobic and electrostatic interactions.
Figure 6. (a) Synthetic route of CSOA. (b) 1H NMR spectrum in D2O/DMSO-d6 (V: V = 1:2) and (c) FTIR spectrum of CS and CSOA.
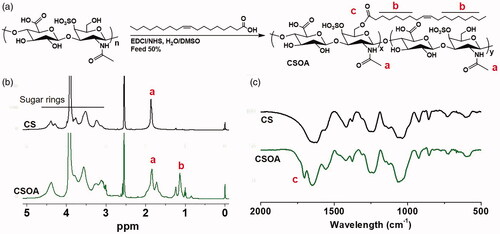
Our DOX delivery system was complete after surface functionalization of NCs for high drug loading and multifunctional drug delivery. Pre-set (drug loading: 70 wt%) amounts of PEGOA and CSOA solutions (H2O) were added into the original NCs solution and the systems experienced sufficient ultrasonication for coating, respectively. It was found that the functionalised DOX NCs exhibited a small swelling in diameter as shown in DLS measurement (). The bigger diameter of CS/NCs and CSOA/NCs was probably due to a higher hydrodynamic volume of sulphate groups on the CS chain that may inhibit undesirable adsorption. The size change of modification NCs in PBS were examined in . It was worth noting that CSOA/NCs exhibited great stability in PBS without significant size change even in two days incubation, whereas PEGOA/NCs and CS/NCs only showed slower diameter growth rate than that of DOX NCs. Meanwhile, we studied the surface charge changes after surface functionalization. showed that the zeta-potential of PEGOA/NC, CS/NCs and CSOA/NCs were respectively 14.2 mV, −23.3 mv, −26.6 mV, that proved the successful incorporation of DOX NCs and coatings. The modification of PEGOA just decreased the charge intensity of NCs, but that of CS and CSOA reversed it to a steady negative surface with the nature of carboxyl and sulphate groups protecting itself from adsorption and destroy. However, CS could not stabilise the NCs without the assist of OA. The union of hydrophobic and electrostatic interactions between the DOX NCs and CSOA was the driving premise for bioapplication, both of which could be omitted. The morphology of CSOA/NCs was monitored by TEM () and SEM (Figure S4) Both of DOX NCs and CSOA/NCs were spherical and monodispersed. It should be noted that CSOA/NCs had a misty membrane surrounding the particles and a slightly bigger size than the original DOX NCs as evidenced. Consist of all these results, we ensured the successful preparation of CSOA/NCs, which showed high stability in physiological environment and high content of drug loading.
The release behaviour of DOX from CSOA/NC was firstly quantificationally analysed using fluorescence spectra (). As a hydrophobic fluorescent substance, DOX stayed in the quenched state after crystal formation in water. The reinforced fluorescent signal initiated from DOX of the drug nanocapsules, which could be detected to evaluate the amount of DOX released [Citation18]. Either DOX NCs or CSOA/NCs showed low fluorescence intensity in aqueous solution, while showed high fluorescence in same amount in DMSO. As previously described, only CSOA/NCs could maintain the stability and dispersibility in PBS. When we replaced PBS with ABS (pH 5.0, 0.01 M), significant fluorescence increase was found in DOX NCs solution due to the protonation to amino group of DOX making its dissolution, but that of CSOA/NCs solution just experienced a little bit augment attributed to the protection of CSOA coating. Hyaluronidase cleaves the GalNAc-HexA bond of CS via hydrolytic cleavage. After HAase was added (100 unit/mL) into CSOA/NCs solution, fluorescence intensity rose notably to the similar level with DOX NCs in ABS. The HAase urged the degradation of CS in acid environment, destroy the coating protection, and accelerated the DOX release. This situation was more obvious with the increase of time.
Figure 7. Fluorescent quantification of DOX NCs and CSOA/NCs after incubation in PBS 7.4 (0.01 M) and ABS 5.0 (0.01 M, with or without 100 unit/mL HAase) for different time at 37°C. The inserted curve was the emission spectrum. (**p<.01).
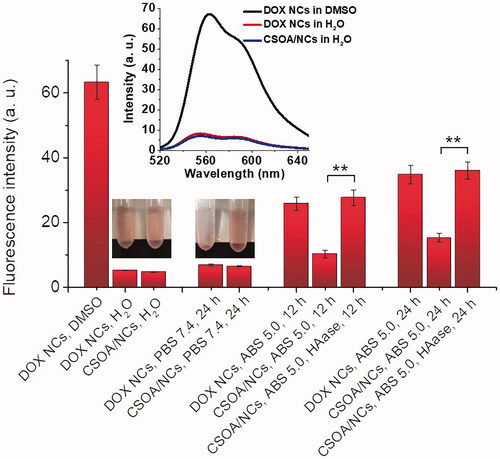
The release behaviour of DOX from CSOA/NCs was also determined using a dialysis method in different medium situation (). Control experiments showed that, less than 20% of DOX diffused out of the spherical aggregates in the whole process in PBS. With the decrease of pH, protonation of DOX inspired the drug spill, with nearly 25% release of DOX at 24 h. Upon HAase treatment, the red colour of the mixture in the dialysis bag shallow a lot in the initial stage. About 50% of the DOX was release from CSOA/NCs at 24 h and complete release achieved at about 94 h. This enzyme-triggered release was slower than that of some other drug delivery system. Because HAase cut the CS into oligosaccharides, which produced more gaps on the particle surface for drug diffusion in acid environment, but essentially did not disrupt the interaction and attachment of CSOA to DOX NCs. Exactly, CS could first bind to CD44 on the surface of the cancer cell, forming caveolae. As the caveolae become endosomes and finally fuse with lysosomes, the pH value decreases to about 5.0 and CS is degraded by HAase creating channel for drug delivery in such an acid cytoplasm.
Finally, we monitored the enzyme-triggered drug release of CSOA/NCs in various types of cells using optical imaging technology and quantitative fluorescence measurement. As shown in , we prepared a typical drug-loaded polymeric micelles system (PEG-PCL/DOX) as a control to examine the selectively release of CSOA/NCs in different cellular culture. To our knowledge [Citation19] and previous description, the fluorescence was highly quenched when DOX was encapsulated into the hydrophobic core of micelles or made into nanocrystal. The fluorescence enhancement could be used to estimate the DOX release after incubation with cells. When PEG-PCL/DOX were incubated with the three cells, the signal enhancements were similar with each other at a low level within a short time. This situation of typical drug-loaded polymeric micelles system was seemed similar with that of CSOA/NCs at 1 h incubation. However, with the longer time, the DOX signal enhancements of CSOA/NCs were dramatically increase to a high level in two cancer cells group comparing with normal fibroblasts (NIH 3T3). This result was confirmed using optical imaging technology, we can easily recognise the stronger red fluorescence increase when CSOA/NCs were treated with HepG2 and Hela cells. These results implied that CSOA/NCs selectively release DOX in cancer cells environments with the cooperation of CD44 and HAase.
The PEG-PCL/DOX micelles and CSOA/NCs were incubated with HepG2 and Hela cells at different drug concentrations to study the in vitro anticancer activities. Firstly, the two blank materials were incubated with 3T3 fibroblasts to evaluate their biocompatibility. As the results presented in . the cell viability of CSOA group were higher than 90% even at the high concentrations of 1 mg/mL. However, the slight toxicity was appeared when the concentrations of PEG-PCL reach 1 mg/mL. The similar results were found as we replaced normal cells with cancer cells (). By the way, the drug-loading content of PEG-PCL/DOX was just 17.8%, which was much lower than CSOA/NCs (70%). That is to say, when high dose treatment was needed, this kind of typical polymer would introduce toxicity. Moreover, the difficult degradation of man-made polymer in living body should be considered. Because it was a nature glycosaminoglycan, CS possesses excellent biocompatibility that was confirmed in our study.
Figure 8. Cell viability of (a) blank materials against NIH 3T3 cells. Cell viability of blank materials, PEG-PCL/DOX and CSOA/NCs after incubation with (b) Hela and (c) HepG2 cells for 48 h, of which (d) the drug loading content and IC50 were exhibited in the table.
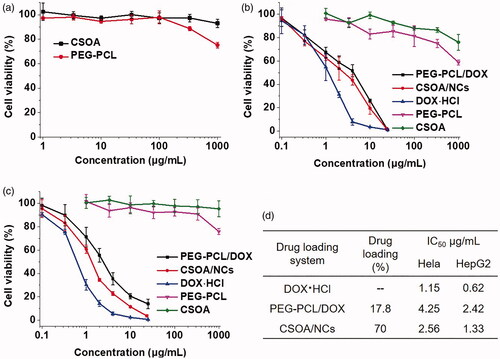
PEG-PCL or CSOA did not show obvious influence to cancer cells at low concentrations, so the anticancer activity of the two DOX-loaded systems was caused by the released drug from them. As shown in , to investigate whether the CSOA/NCs had an enhanced anticancer efficiency to cancer cells, we measured the viability of HepG2 cells and Hela cells by CCK-8 assay using PEG-PCL/DOX micelles as a control. All materials showed dose-dependent cytotoxicity to these two cancer cells. The IC50 (the half maximal inhibitory concentration) values of free DOX, PEG-PCL/DOX, and CSOA/NCs were measured as 0.62, 2.42, 1.33 μg/mL to HepG2 cells, and 1.15, 4.25, 2.56 μg/mL to Hela cells, respectively. Free DOX showed a highest potency as a comparation. Given that DOX is a hydrophilic small molecule, it would be quickly transport into cells and enter the nuclei by passive diffusion [Citation20]. As lots of papers reported [Citation1,Citation21,Citation22], the lower inhibition effect of DOX-loaded system was attributed to the slow cellular uptake via endocytosis and drug release, which retarded the diffusion of drug to the nuclei. More importantly, the cytotoxicity of CSOA/NCs was significantly higher than that of DOX-loaded PEG-PCL micelles. This result might be due to the faster uptake of CSOA/NCs by CD44 receptor-mediated endocytosis, and accelerated DOX release from them in an intracellular compartment. Then we especially study the cellular uptake of CSOA/NC against HepG2 and NIH 3T3 cells.
In order to check the targeted effects on cellular uptake of CSOA/NC in cancer cells over expressing CD44 (). In , after cells were pre-treated with free CS (5 mg/mL, pH 7.4) for 2 h, the red fluorescence was negligible due to the lower intracellular DOX. Pre-treatment with free CS to HepG2 cells led to the partial inhibition of CSOA/NCs accumulation, which provided the direct involvement for receptor-mediated mechanism of uptake. The interaction of flexible OA moieties with the cellular membrane also played a synergism role in cellular internalization of CSOA/NCs, that was a congenital advantage to another high drug-loading system such as prodrug (HA-drug) [Citation22] or PEG-drug conjugates After the 4 h-incubation, CSOA/NCs have passed into the cytoplasm of cells. A mass of drug was delivered around the nucleus, and bits of them even entered into the nucleus. With the longer incubation time, almost all of the drug had transported into the nucleus. We also used NIH 3T3 cells as CD44-receptor-negative cell line to study the cellular uptake of CSOA/NCs (Figure S5). The fluorescence intensity of DOX from CSOA/NCs in NIH 3T3 cells was much weaker than that in HepG2 cells. In , HepG2 cells were used as a negative control for flow cytometry measurement. In the cells treated with CSOA/NCs, with and without pre-treatment of CS solution for 0.5, 4, and 12 h, respectively, the fluorescence intensity of cells treated with CSOA/NCs without pre-treatment of CS solution for 12 h was the highest, which agreed with the results of CLSM. It is saying that CSOA/NC could be effectively taken up by cells through CD44 receptor-mediated endocytosis. As cellular internalization, HAase cut the CS into oligosaccharides creating gaps on the surface of CSOA/NCs. The drug diffusion was speeded up in such an acid environment leading to the rapid release of DOX into cytoplasm of cells.
Figure 9. (a′) Confocal microscopy images of HepG2 cells treated with CSOA/NCs, (a, b) with and (c, d, e) without pre-treatment of CS solution for (a, c) 0.5 h, (b, d) 4 h and (e) 12 h, respectively. The white arrows mean the cumulation of DOX in cells. The scale bar was 10 μm. (b′) The diagrams of flow cytometry histogram profiles of HepG2 cells from a to e were the corresponding CLSM results.
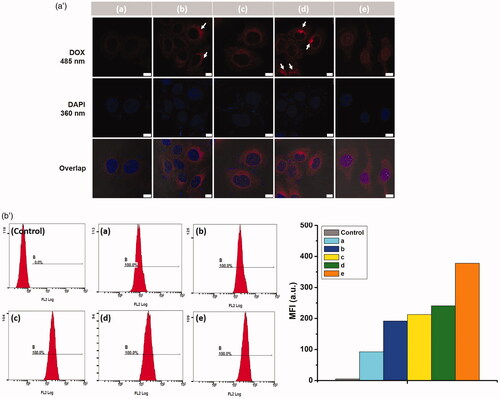
Conclusions
In summary, we successfully developed a strategy for fabricating targeted DOX delivery system by surface modification on doxorubicin nanocrystals. In the process of preparation, we found that lots of factors would influence the size of DOX NCs deeply, of which a desired scale (102 nm) was particularly selected. The common adsorption of amphiphilic molecules or opposite charged polyelectrolyte would not stabilized DOX NCs under physiological conditions, while the coating of chondroitin sulphate derivative (CSOA) via the combination of electrostatic and hydrophobic interactions could be qualified. This outerwear endowed the drug nanocapsules with high drug loading content up to 70%, and accelerated DOX release in response to hyaluronidase or exposure in internal environment of cancer cells. Along with the CS-specific receptor-mediated uptake, CSOA/NCs could rapidly transport and release DOX to tumour cells, leading to the improved antitumor activity compared with PEG-PCL/DOX. The novel concept shown here may develop a rationally preparation and utilization of drug nanocrystalline in controlled release study, and for the first time afforded a sensitive and targeted DOX nanocrystal delivery system.
Supplementary_Information-LABB-2019-2211-revised.docx
Download MS Word (8.4 MB)Acknowledgement
We appreciate the help from Engineer Yong Lv of Hisense Electronic Information Group R&D Centre.
Disclosure statement
No potential conflict of interest was reported by the author(s).
Correction Statement
This article has been republished with minor changes. These changes do not impact the academic content of the article.
Additional information
Funding
References
- Liang Y, Deng X, Zhang L, et al. Terminal modification of polymeric micelles with π-conjugated moieties for efficient anticancer drug delivery. Biomaterials. 2015;71:1–10.
- Davis ME, Chen ZG, Shin DM. Nanoparticle therapeutics: an emerging treatment modality for cancer. Nat Rev Drug Discov. 2008;7(9):771–782.
- Chen X, Parelkar SS, Henchey E, et al. PolyMPC-doxorubicin prodrugs. Bioconjug Chem. 2012;23(9):1753–1763.
- Wang Y, Fu H, Peng A, et al. Distinct nanostructures from isomeric molecules of bis(iminopyrrole) benzenes: effects of molecular structures on nanostructural morphologies. Chem Commun. 2007;16(16):1623–1625.
- Shutava TG, Pattekari PP, Arapov KA, et al. Architectural layer-by-layer assembly of drug nanocapsules with PEGylated polyelectrolytes. Soft Matter. 2012;8(36):9418–9427.
- Xiong XB, Lavasanifar A. Traceable multifunctional micellar nanocarriers for cancer-targeted co-delivery of MDR-1 siRNA and doxorubicin. ACS Nano. 2011;5(6):5202–5213.
- Liang Y, Gao W, Peng X, et al. Near infrared light responsive hybrid nanoparticles for synergistic therapy. Biomaterials. 2016; 100:76–90.
- Duhem N, Danhier F, Pourcelle V, et al. Self-Assembling doxorubicin-tocopherol succinate prodrug as a new drug delivery system: synthesis, characterization, and in vitro and in vivo anticancer activity. Bioconjug Chem. 2014;25(1):72–81.
- Li W, Yang Y, Wang C, et al. Carrier-free, functionalized drug nanoparticles for targeted drug delivery. Chem Commun. 2012;48(65):8120–8122.
- Lü S, Li B, Ni B, et al. Thermoresponsive injectable hydrogel for three-dimensional cell culture: chondroitin sulfate bioconjugated with poly(N-isopropylacrylamide) synthesized by RAFT polymerization. Soft Matter. 2011;7(22):10763–10772.
- Kurosaki T, Kitahara T, Kawakami S, et al. The development of a gene vector electrostatically assembled with a polysaccharide capsule. Biomaterials. 2009;30(26):4427–4434.
- Zaia J, Costello CE. Compositional analysis of glycosaminoglycans by electrospray mass spectrometry. Anal Chem. 2001;73(2):233–239.
- Li W, Liao P, Su C, et al. Formation of oligonucleotide-gated silica shell-coated Fe-O-Au core-shell nanotrisoctahedra for magnetically targeted and near-infrared light-responsive theranostic platform. J Am Chem Soc. 2014;136(28):10062–10075.
- Morelli D, Ménard S, Colnaghi MI, et al. Oral administration of anti-doxorubicin monoclonal antibody prevents chemotherapy-induced gastrointestinal toxicity in mice. Cancer Res. 1996;56(9):2082–2085.
- Zhang X, Zhang X, Zou K, et al. Single-crystal nanoribbons, nanotubes, and nanowires from intramolecular charge-transfer organic molecules. J Am Chem Soc. 2007;129(12):3527–3532.
- Lai Y, Lei Y, Xu X, et al. Polymeric micelles with π–π conjugated cinnamic acid as lipophilic moieties for doxorubicin delivery. J Mater Chem B. 2013;1(34):4289–4296.
- Williford JM, Santos JL, Shyam R, et al. Shape control in engineering of polymeric nanoparticles for therapeutic delivery. Biomater Sci. 2015;3(7):894–907.
- Deng X, Liang Y, Peng X, et al. A facile strategy to generate polymeric nanoparticles for synergistic chemo-photodynamic therapy. Chem Commun. 2015;51(20):4271–4274.
- Cui C, Xue Y, Wu M, et al. Cellular uptake, intracellular trafficking, and antitumor efficacy of doxorubicin-loaded reduction-sensitive micelles. Biomaterials. 2013;34(15):3858–3869.
- Li N, Cai H, Jiang L, et al. Enzyme-sensitive and amphiphilic PEGylated dendrimer-paclitaxel prodrug-based nanoparticles for enhanced stability and anticancer efficacy. ACS Appl Mater Interfaces. 2017;9(8):6865–6877.
- Wang X, Li F, Yan X, et al. Ambient aqueous synthesis of ultrasmall Ni0.85Se nanoparticles for noninvasive photoacoustic imaging and combined photothermal-chemotherapy of cancer. ACS Appl Mater Interfaces. 2017;9(48):41782–41793.
- He Y, Nie Y, Cheng G, et al. Viral mimicking ternary polyplexes: a reduction-controlled hierarchical unpacking vector for gene delivery. Adv Mater Weinheim. 2014;26(10):1534–1540.