Abstract
Brain glioma is the most common primary tumour of the central nervous system. Complete surgical removal of the brain glioma is virtually impossible. Chemotherapy is still an important treatment for brain glioma. However, blood–brain barrier (BBB) and vasculogenic mimicry (VM) channels remain two hindrances in regular treatments. Herein, we developed a novel nanoscaled dual targeting daunorubicin plus rofecoxib liposomes which could transport across the BBB, and eliminate brain glioma cells along with the VM channels. The liposomes were modified with two functional materials, and showed round in shape with a diameter about 120 nm. Evaluations were performed on human brain glioma U87MG cells in vitro and on intracranial brain glioma-bearing nude mice. The dual targeting liposomes demonstrated a long circulatory effect in the blood system, were able to transport across the BBB, and were accumulated into the brain. The results indicated that the dual targeting daunorubicin plus rofecoxib liposomes could inhibit the brain glioma VM channels and exhibited a significant efficacy in the treatment of intracranial glioma-bearing nude mice. The mechanisms are related to down regulations MMP-2, MMP-9, FAK and HIF-α. Hence, the established dual targeting liposomes could be a potential formulation to treat the brain glioma along with eliminating VM channels.
Introduction
Brain glioma is the most common type in the central nervous system (CNS) cancers [Citation1]. Despite of recent advances in glioma therapy, the prognosis of this fatal disease is still poor. Brain glioma patients have a median survival time of less than 16 months after comprehensive treatment, which are mainly consisted of surgery, radiotherapy and chemotherapy [Citation2]. However, the highly infiltrating property and the location of brain glioma make complete removal of the cancer cells impossible [Citation3]. While radiotherapy applications are also limited by radiation-related encephalopathy. Chemotherapy is still an important treatment in preoperative tumour reduction and postoperative recurrence prevention.
As for the brain glioma, chemotherapy usually end up with failure because of the inability of anticancer drugs to reach the glioma tissue. Blood–brain barrier (BBB), which is formed by endothelial cell monolayer associated with pericytes and astrocytes, separates the blood transportation from the cerebral tissue preventing the drugs enter the CNS [Citation4]. In fact, the BBB prevents nearly all large-molecule and more than 98% of small-molecule drugs from entering the brain glioma tissue. Another obstacle is the infiltrative growth of the glioma cells, which can form vasculogenic mimicry (VM) channels by remoulding, and the generated VM channels provide nutrients to support the growth or recurrence of brain glioma cells under the condition of hypoxia [Citation5–8].
Hence, a satisfactory chemotherapy need first overcome the limitation in BBB delivery, and then solve the infiltrative growth of the glioma cells [Citation9]. In the present study, we developed a dual modified nanocarrier system, in which two lipid derivative cell-penetrating peptide GGPFVYLI and TPGS1000-MAN were modified onto the surface of the liposomes as functional materials. In the targeting liposomal drugs system, daunorubicin and rofecoxib were incorporated into liposome vesicles as an anticancer agent and modulating agent, respectively. We hypothesize that the dual targeting daunorubicin plus rofecoxib liposomes could transport across the BBB and target the brain glioma along with eliminating the VM channels.
4-Aminophenyl β-d-glucopyranoside (GLU) is a glucose derivative that is transported across the BBB through a glucose transporter (GLUT). It can penetrate the brain efficiently via facilitative GLUTs as the BBB over-expresses GLUTs [Citation10]. A glucose-vitamin E derivative (4-aminophenyl β-d-glucopyranoside-d-α-tocopheryl polyethylene glycol 1000 succinate, GLU-TPGS1000) conjugate was included as an acceptor molecule for transporting drugs across the BBB. Distearoylphosphatidylethanolamine polyethylene glycol2000 (DSPE-PEG2000) has also been used as a functional material for maintaining the stability and long circulation of drug carriers in blood [Citation11], as DSPE-PEG2000 can avoid the rapid clearance of nanoparticles by the reticuloendothelial system [Citation12]. GGPFVYLI is a kind of cell-penetrating peptide (CPP) of small molecule, shows low toxicity and good stability. In the present study, a conjugate of DSPE–PEG2000–GGPFVYLI was synthesized. The CPP can effectively transport liposomes across the cell membrane to improve the uptake of drugs in cancer cells.
The objectives of the present study were to: (i) develop dual targeting daunorubicin plus rofecoxib liposomes; (ii) evaluate the abilities on transportation across the BBB; (iii) evaluate the effects on eliminating brain glioma cells and the VM channels; and (iv) explore the relevant mechanisms.
Materials and methods
Reagents and cell lines
Daunorubicin, rofecoxib, cholesterol and egg phosphatidyl choline (EPC) were purchased from Meilun Biotechnology Co., Ltd. (Dalian, China). DSPE-PEG2000 and DSPE-PEG2000-NHS were obtained from NOF Corporation (Tokyo, Japan). GGPFVYLI peptide was synthesized by Shanghai A-peptide Co., Ltd. (Shanghai, China). Other chemicals were analytical or HPLC grade. GLU, DCC, DMAP, TPGS1000, sulforhodamine-B and Sephadex G-50 were purchased from Sigma-Aldrich (St. Louis, MO). Other reagents were of analytical pure, purchased from Beijing Chemical Reagents (Beijing, China).
Brain glioma U87MG cells were purchased from the Institute of Basic Medical Science, Chinese Academy of Medical Science (Beijing, China). Cell culture medium was Minimum Essential Medium Eagle (MEM, Macgene Beijing Biotechnology Ltd., Beijing, China) supplemented with 10% foetal bovine serum (FBS, Gibco Beijing local agent, Beijing, China). Murine brain microvascular endothelial cells (BMVECs) were obtained from China-Japan Friendship Hospital (Beijing, China). BMVECs were cultured with Dulbecco’s Modified Eagle Medium (DMEM) containing 20% FBS, 100 U/ml penicillin, 100 μg/ml streptomycin, 2 mmol/l l-glutamine, 100 μg/ml endothelial cell growth factor and 40 U/ml insulin. The cells were cultured at 37 °C under an atmosphere of 5% CO2.
Male NU/NU nude mice (18–20 g) were obtained from Peking University Experimental Animal Centre (Beijing, China). Experiments involving human cells and mice were approved by the Ethics Committee of the Health Science Centre of Peking University.
Synthesis of targeting molecules
DSPE–PEG2000–GGPFVYLI was synthesized and characterized according to the methods employed in earlier publication [Citation13]. GLU–TPGS1000 conjugate was synthesized as the following procedures. Glutaric acid (0.4 mmol), DMAP (0.2 mmol) and DCC (0.2 mmol) were dissolved in DMSO (4 ml). The mixture was stirred using a magnetic stirrer at room temperature under nitrogen gas protection for 2 h. After adding TPGS1000 (0.1 mmol), the reaction mixture was stirred using a magnetic stirrer at room temperature for 24 h. The crude product was then transferred to a regenerated cellulose dialysis tubing (molecular weight cut-off point, 1500) and dialysed against deionized water for 48 h to remove uncoupled materials and DMSO. The resultant TPGS1000–COOH was obtained by freeze-drying.
Afterwards, TPGS1000–COOH (10 mmol), EDC (40 mmol) and NHS (70 mmol) were dissolved in pyridine–DMSO (1:1, 2 ml). The mixture was stirred for 30 min, followed by adding GLU (10 mmol), and stirring for 12 h. The crude product was dialysed against deionized water in the regenerated cellulose dialysis tubing for 48 h to remove uncoupled GLU, EDC, NHS, DMSO and pyridine. The resultant product was obtained by freeze-drying. The production mixture was identified and characterized using matrix-assisted laser desorption/ionization time of flight mass spectrometry (MALDI-TOF-MS Instrument, Shimadzu, Kyoto, Japan).
Preparation of liposomes
The dual targeting daunorubicin plus rofecoxib liposomes were prepared as previously reported [Citation14]. The constituents included EPC, cholesterol, GLU–TPGS1000, DSPE–PEG2000–GGPFVYLI (70:30:3:3, molar ratio) and drugs (daunorubicin:rofecoxib = 1:1, molar ratio). Briefly, lipid materials and celecoxib were dissolved in chloroform into a pear-shaped bottle. Then, procedures of filming-rehydration method were undertaken, followed by using ammonium sulphate gradient method to encapsulate daunorubicin. Free daunorubicin, daunorubicin liposomes, rofecoxib liposomes, daunorubicin plus rofecoxib liposomes and GGPFVYLI modified daunorubicin plus rofecoxib liposomes were included as the control formulations in varying evaluation tests. Fluorescently labelled liposomes were prepared by encapsulating coumarin or 1,1-dioctadecyl-3,3,3,3-tetramethylindotricarbocyanine iodide (DiR) into the bilayer of the liposome, and used as the fluorescent probes.
Characterization of the liposomes
Particle morphology of dual targeting daunorubicin plus rofecoxib liposomes was determined by a transmission electron microscopy and an atomic force microscopy. The particle diameter and zeta potential of liposomes were measured by a Zetasizer (Malvern Instruments Ltd., Malvern, UK). The concentration of daunorubicin liposomes formulations was analysed by a high-performance liquid chromatography (HPLC) equipped with a UV detector (Agilent Technologies Inc., Cotati, CA).
The encapsulation efficiency (EE) of daunorubicin or rofecoxib was calculated using the formula: EE = (Wencap/Wtotal)×100%, where Wencap is the measured amount of daunorubicin or rofecoxib in the liposome suspensions after passing over the Sephadex G-50 column, and Wtotal is the measured amount of daunorubicin or rofecoxib in the initial liposome suspensions. The drug-loading capacity was calculated as follows: LE (% )= (Wn/Wm)×100%, where Wn represents the amount of drug encapsulated in the liposomes and Wm represents the total weight of the liposomes. In vitro release of daunorubicin or rofecoxib from the liposomes was performed by dialysis against the release medium (PBS alone, PBS containing 10% FBS, respectively) at 37 °C. Daunorubicin or rofecoxib content in the release medium was measured by the HPLC method. The release rate (RR, %) was calculated using the formula: RR = (Wi/Wtotal) × 100%, where Wi is the measured amount of daunorubicin or rofecoxib at the ith time-point in the release medium, and Wtotal is the total amount of daunorubicin or rofecoxib in the equal volume of liposome suspensions prior to dialysis. Each assay was repeated in triplicate.
Cellular uptake in glioma cells and BMVECs cells
To investigate the binding ability of the varying liposomes formulations to the U87MG cells and BMVECs cells. Coumarin was entrapped into the liposomes and used as fluorescence probe. Briefly, a density of 2 × 105 cells per well was seeded into six-well culture plate and incubated for 24 h at 37 °C. Then, both cells were treated with the varying formulations at a concentration of 1 μM coumarin, with blank medium was used as blank control. Treatment groups were consisted of coumarin liposomes, GGPFVYLI modified coumarin plus rofecoxib liposomes, dual targeting coumarin plus rofecoxib liposomes and free coumarin. After treatment for 4 h, the wells were washed three times and the cells were trypsinized and harvested in 0.3 ml PBS buffer. Fluorescence intensity of coumarin in the samples was analysed by a Flow Cytometer (Becton Dickinson, San Jose, CA) collecting 1 × 104 cells. Three multiple wells were set as each sample.
Cytotoxicity on brain glioma cells
To evaluate the cytotoxic effect of dual targeting daunorubicin plus rofecoxib liposomes, brain glioma U87MG cells were seeded at a density of 6 × 103 cells/well in 96-well culture plates, and were cultured for 24 h. The cells were then treated with daunorubicin liposomes, daunorubicin plus rofecoxib liposomes, GGPFVYLI modified daunorubicin plus rofecoxib liposomes, dual targeting daunorubicin plus rofecoxib liposomes. Both concentrations of daunorubicin and rofecoxib were in the range of 0–10.0 μM (daunorubicin:rofecoxib = 1:1, μM/μM). After incubation for another 48 h, the cytotoxicity was observed with sulforhodamine B (SRB) colorimetric assay [Citation15]. Briefly, culture medium was discarded, and the cells were then fixed with 10% trichloroacetic acid, followed by staining with the SRB. Absorbance values were measured at 540 nm using a microplate reader (HBS-1096A; DeTie Laboratory Equipment, Nanjing, China). The survival ratios were calculated using the following formula: survival rate % = (A540 nm for treated cells/A540 nm for control cells) × 100%, where A540 nm represents the absorbance value.
Transport ability across the BBB
To evaluate the transport ability across the BBB, the in vitro BBB model was established as described previously [Citation5,Citation16]. Briefly, BMVECs were seeded into the gelatine solution coated transwells (0.4 μm pore size, 12 mm diameter and 1.12 cm2 surface area; Corning, NY) at a density of 1 × 104 cells/well, and were incubated for six days. Culture medium was changed every other day. During experiment, tightness of the established BBB model was evaluated by measuring the transepithelial electrical resistance (TEER) values. Once the TEER values were higher than 200 Ω·cm2, the BBB model was applied for experiments. Brain glioma U87MG cells were seeded at the bottom of 12-well culture plate under a transwell at a density of 2 × 104 cells/well. To evaluate the transport ratio of drug-loaded liposomes across the BBB, varying liposomal formulations were added in the transwells at a concentration of 10 μM daunorubicin, respectively. After incubation for 4 h, the inserts were moved away, and brain glioma U87MG cells were further incubated for 24 h. The following procedures were the same as those used in SRB assay. The transport ability of drug-loaded liposomes across the BBB model was evaluated by measuring the survival ratio of brain glioma cells in the lower compartment under the BBB insert.
Inhibitory effects on VM channels
To evaluate the destructive effect of dual targeting daunorubicin plus rofecoxib liposomes, VM channels of brain glioma were established with matrigel based formation method [Citation17]. Briefly, 96-well culture plates were first coated with matrigel (50 μl/well) and allowed to agglutinate at 37 °C for 30 min. Then, brain glioma U87MG cells were seeded into the culture plates at a density of 1 × 104 cells/well in serum-free MEM medium. Varying liposomal formulations including daunorubicin liposomes, rofecoxib liposomes, daunorubicin plus rofecoxib liposomes, GGPFVYLI modified daunorubicin plus rofecoxib liposomes, and dual targeting daunorubicin plus rofecoxib liposomes (5 μM daunorubicin or rofecoxib) were added into the culture system, respectively. Blank medium was used as a control. After incubation for 12 h, each well was photographed and analysed using an inverted microscope (XDS-1B; Chongqing Photoelectric Co., Ltd., Chongqing, China).
Regulation of VM associated proteins
To explore the inhibitory mechanism on glioma cell VM formation, the contents of MMP-2 (matrix metalloproteinase-2), MMP-9 (matrix metalloproteinase-9), FAK (focal adhesion kinase) and HIF-1α (hypoxia inducible factor-1α) were determined by the ELISA assay. Briefly, brain glioma U87MG cells were cultured to 80% confluence, and then treated with daunorubicin liposomes, rofecoxib liposomes, daunorubicin plus rofecoxib liposomes, GGPFVYLI modified daunorubicin plus rofecoxib liposomes, and dual targeting daunorubicin plus rofecoxib liposomes at a concentration of 5 µM daunorubicin or/and 5 µM rofecoxib. The culture medium was used as blank control. After incubation for 12 h, the cells were harvested and lysed. The cell lysates were analysed using the microplate reader according to the manufacturers’ instructions of the kits. The protein activity ratio was calculated using the following formula: activity ratio % = (A450nm for treated cells/A562nm for treated cells)/(A450nm for control cells/A562nm for control cells), where A450nm and A562nm represent absorbance values, respectively.
In vivo imaging and pharmacodynamics study in glioma-bearing mice
To evaluate the in vivo distribution and the anticancer efficacy, brain glioma-bearing mice model was established by intracranial implantation [Citation18]. A total of 50 male NU/NU nude mice were included for the study. Briefly, the nude mice were anaesthetized by 4% chloral hydrate (10 μl/g) and incised through the skin to expose the cranium. The coordinate of inoculation point was set at 1.0 mm anterior from the coronal suture, 3.5 mm right-lateral from the sagittal suture, and 3.0 mm in depth. A volume of 3 μl brain glioma U87MG cells (1 × 105 cells/μl) was implanted into the inoculation point of each nude mouse using a stereotaxic instrument (RWD Life Science Co. Ltd., Shenzhen, China).
To evaluate in vivo drug distribution in brain glioma site after treatment, targeting DiR liposomes were intravenously administrated as the fluorescent probe. Briefly, 12 male nude mice were in situ inoculated with brain glioma U87MG cells as above. After inoculation at day 14, the mice were randomly divided into four groups, and administered physiological saline, free DiR, DiR liposomes and dual-targeting DiR plus rofecoxib liposomes via tail vein at a dose of 1 μg DiR per mouse. Fluorescent images and X-ray images were captured using an in vivo imaging system (Carestream, Fx Pro, Rochester, NY) at varying time-points (1, 3, 6, 12, 24 and 48 h).
To study the overall treatment efficacy, the mice were randomly divided into six groups (six nude mice/group) at day 14 post inoculation. Saline, free daunorubicin, daunorubicin liposomes, daunorubicin plus rofecoxib liposomes, GGPFVYLI modified daunorubicin plus rofecoxib liposomes, and dual-targeting daunorubicin plus rofecoxib liposomes were administered to mice via tail vein at a dose of 5 mg/kg daunorubicin (daunorubicin:rofecoxib = 1 μM:1 μM in rofecoxib containing formulations), respectively. Treatments were made every other day for consecutive four times. The survival of the mice was observed and the survival curve was made accordingly.
To evaluate the potential toxicity after treatment with targeting daunorubicin plus rofecoxib liposomes, the heart, liver, spleen, lung and kidney tissues were removed from sacrificed mice during survival investigation. The tissues were fixed in 10% formalin, decalcified in 10% ethylene diamine tetraacetic acid disodium (EDTA-2Na), embedded in paraffin, and then stained with hematoxylin–eosin (H&E). The toxic effect on major organs between group saline and targeting daunorubicin plus rofecoxib liposomes was assessed under a light microscope according to a standard protocol.
Statistical analysis
Data were presented as mean ± standard deviation (SD). One-way analysis of variance was used to estimate the significance among groups. Post-hoc tests with the Bonferroni correction were used for multiple comparisons between individual groups. A value of p< .05 was considered to be significantly different.
Results and discussion
Synthesis of targeting molecule
shows the MALDI-TOF-MS spectra of TPGS1000 () and TPGS1000-GLU (). From the spectra, the average mass values of TPGS1000 and TPGS1000–GLU were about 1710 and 2124, respectively. The molecular mass difference between these two molecules is exactly the molecular mass of GLU and the linker glutaric acid, indicating that the targeted molecule has been synthesized successfully.
Characterization of the liposomes
shows the characterization of dual targeting daunorubicin plus rofecoxib liposomes. depicts TEM images of dual targeting daunorubicin plus rofecoxib liposomes. The liposomes were basically spherical, which were further confirmed as three dimensional spheroids with smooth surfaces from AFM results (). The dual targeting daunorubicin plus rofecoxib liposomes showed slightly electronegative with diameter about 120 nm (). shows the encapsulation efficiencies, particle sizes, polydispersity indexes and zeta potential of the varying liposomes. The average particle sizes of dual targeting daunorubicin plus rofecoxib liposomes were 118.76 ± 3.86 nm with narrow polydispersity indexes. In all liposomal formulations, the encapsulation efficiencies of daunorubicin and rofecoxib were greater than 80% with narrow polydispersity indexes (≤0.20). The loading capacities of daunorubicin and emodin in R8GD modified daunorubicin liposomes and R8GD modified emodin liposomes were 1.1 ± 0.18% and 3.31 ± 0.23%, respectively. The in vitro RRs of daunorubicin and rofecoxib from the liposomes in the simulated body fluids were less than 10% within the initial 6 h. Over 48 h, the RRs of daunorubicin and rofecoxib were approximately 40%. PBS containing 10% FBS showed a faster RR than those of PBS alone. Liposomes have the advantages of small particle size, high encapsulation rate and high bioavailability. They can encapsulate both hydrophilic drugs and hydrophobic drugs with their phospholipid bilayer structures. They can reduce the toxicity of antitumor drugs and enhance the antitumor efficacy. In addition, the lipid bilayer of liposomes is composed of phospholipid and cholesterol, which are quite similar to the cell membrane, and thus showing an excellent biocompatibility. Compared with cubosomes and spongosomes, liposomes have more products on the market and are more widely used in clinical cancer treatment [Citation19,Citation20] ().
Figure 2. Characterization of dual targeting daunorubicin plus rofecoxib liposomes. (A) TEM image of dual targeting daunorubicin plus rofecoxib liposomes, (B) two-dimension AFM image of dual targeting daunorubicin plus rofecoxib liposomes, (C) three-dimension AFM image of dual targeting daunorubicin plus rofecoxib liposomes, (C) size distribution of dual targeting daunorubicin plus rofecoxib liposomes and (D) zeta value of dual targeting daunorubicin plus rofecoxib liposomes.
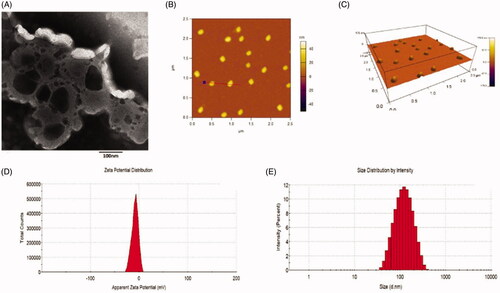
Figure 3. In vitro release rates of drugs from varying liposomes in the simulated body fluids. (A) Release rate of daunorubicin from varying formulations in PBS solution containing 10% FBS, (B) release rate of daunorubicin from varying formulations in PBS, (C) release rate of rofecoxib from varying formulations in PBS solution containing 10% FBS, (D) release rate of rofecoxib from varying formulations in PBS. Data are presented as mean ± standard deviation (SD) (n = 3).
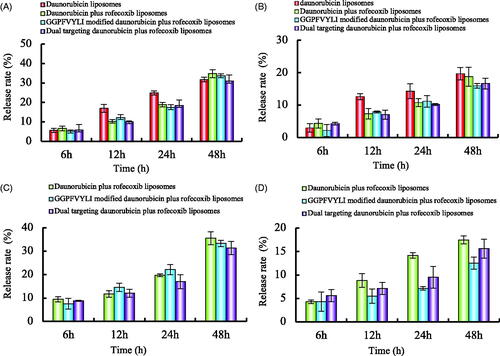
Table 1. Characterization of the liposomes.
Cellular uptake in glioma cells and BMVECs cells
After treatments with varying drug formulations, the fluorescent intensity in brain glioma U87MG cells and BMVECs cells measured by flow cytometry demonstrated that the GGPFVYLI modified coumarin plus rofecoxib liposomes were internalized more than coumarin liposomes. While the dual targeting coumarin plus rofecoxib liposomes showed the highest fluorescent intensity in the three liposomal formulations in both brain glioma cells () and BMVECs cells (). Results from semi-quantitative evaluation by flow cytometry showed the rank of cellular uptake was free coumarin > dual targeting coumarin plus rofecoxib liposomes > GGPFVYLI modified coumarin plus rofecoxib liposomes > coumarin liposomes > blank medium. The mechanism could be related to the targeting molecules GGPFVYLI peptide and GLU, which increase the affinity by electrostatic attraction and receptor-mediated endocytosis, respectively.
Figure 4. Cellular uptake after incubation with varying formulations. (A) Cellular uptake of U87MG cells and (B) cellular uptake of BMVECs cells. (a) Blank control; (b) coumarin liposomes; (c) GGPFVYLI modified coumarin plus rofecoxib liposomes; (d) dual targeting coumarin plus rofecoxib liposomes; (e) free coumarin.
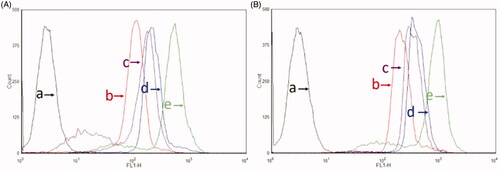
Cytotoxicity on brain glioma cells and transport ability of BBB
Daunorubicin is widely used in treating a variety of cancers. The underlying mechanism that daunorubicin takes effects is mainly by interfering DNA and RNA synthesis in the cellular nuclei [Citation21]. Rofecoxib is a cyclooxygenase 2 selective inhibitor, which is used for the treatment of inflammations. Increasing evidence has exhibited that rofecoxib could inhibit the formation of VM channels in the tumour tissues [Citation22]. In this study, a type of dual targeting drug-loaded lipid vesicles was developed by modifying the liposomes with two functional materials, TPGS1000–GLU and DSPE–PEG2000–GGPFVYLI. The constructed nanoscaled liposomes could be transported across the BBB by via receptor-mediated endocytosis and adsorptive mediated endocytosis, thereby eliminating brain glioma by triggering their necrosis and apoptosis.
Results from cytotoxicity assay showed that the targeting daunorubicin plus rofecoxib liposomes demonstrated the strongest cytotoxic effect to brain glioma cells (). The transportability of targeting daunorubicin plus rofecoxib liposomes was evidenced by the inhibitory effect to brain glioma U87MG cells after transporting across the BBB. Results showed that the survival rate rank of brain glioma cells was targeting daunorubicin plus rofecoxib liposomes < GGPFVYLI modified daunorubicin plus rofecoxib liposomes < daunorubicin plus rofecoxib liposomes < daunorubicin liposomes (), demonstrating the strongest transportability of the dual targeting drug-loaded liposomes across the BBB, followed by killing effect to brain glioma cells.
Inhibitory effects on VM channels and the related mechanisms
Brain glioma cells could form VM channels to support the continuous growth. Therefore, destruction of VM channels becomes an important scientific issue in anti-glioma treatment. In this evaluation, brain glioma U87MG cells were ready to form VM channels in 3D matrigel. After treatment with different formulations, the rank of inhibitory effect on the VM channels was dual targeting daunorubicin plus rofecoxib liposomes > GGPFVYLI modified daunorubicin plus rofecoxib liposomes > daunorubicin plus rofecoxib liposomes > rofecoxib liposomes > daunorubicin liposomes ().
Figure 7. Destruction of VM channels in vitro formed by brain glioma U87MG cells in the three dimension (3D) matrigel after treatment with dual targeting daunorubicin plus rofecoxib liposomes. (1) Blank control; (2) daunorubicin liposomes; (3) rofecoxib liposomes; (4) daunorubicin plus rofecoxib liposomes; (5) GGPFVYLI modified daunorubicin plus rofecoxib liposomes; (6) dual targeting daunorubicin plus rofecoxib liposomes. p<.05. (a) vs. 1; (b) vs. 2; (c) vs. 3; (d) vs. 4.
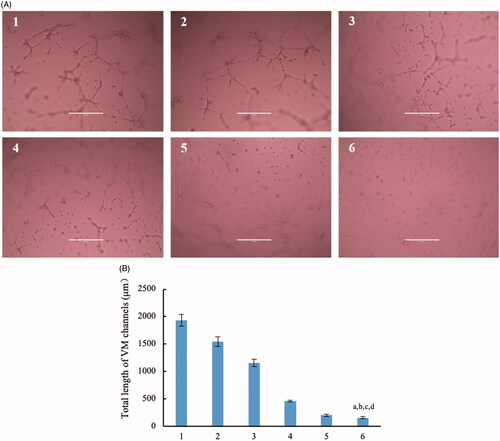
The phenotype-transformed tumour cells in VM channels are induced by the specific microenvironment and the secreted induction factors, such as HIF-1α, under the condition of hypoxia. The expressions of the VM channel indicators were evaluated on brain glioma U87MG cells. After treatments with daunorubicin liposomes, rofecoxib liposomes, daunorubicin plus rofecoxib liposomes, dual targeting daunorubicin plus rofecoxib liposomes, the expressions of MMP-2, MMP-9, FAK and HIF-1α were significantly inhibited in brain glioma cells (). In comparison of the effects among different formulations, the dual-targeting daunorubicin plus rofecoxib liposomes exhibited the most significant inhibitory effects on these indicators. The mechanism study demonstrates that the down regulations of MMP-2, MMP-9, FAK and HIF-1α are involved in the destruction of brain glioma VM channels.
Figure 8. Inhibition on the VM indicators in brain glioma cells after treatment with dual targeting daunorubicin plus rofecoxib liposomes. (1) Blank control; (2) daunorubicin liposomes; (3) rofecoxib liposomes; (4) daunorubicin plus rofecoxib liposomes; (5) dual targeting daunorubicin plus rofecoxib liposomes. p<.05. (a) vs. 1; (b) vs. 2; (c) vs. 3; (d) vs. 4. Data are presented as the mean ± SD (n = 4).
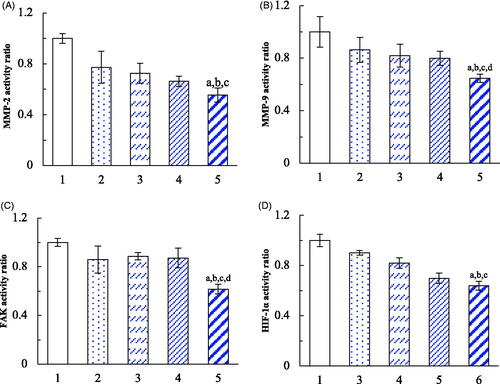
In vivo distribution and treatment efficacy in brain glioma-bearing mice
After the administration of dual targeting DiR plus rofecoxib liposomes, strong fluorescent signals were observed in the blood circulation and in the brain glioma site (). Furthermore, the fluorescent signal of the dual-targeting DiR plus rofecoxib liposomes maintained up to 48 h in the brain location. After the administration of DiR liposomes, the fluorescent signal was also found in the blood circulation and in brain location till 12 h. For that of free DiR, the fluorescent signal accumulated in livers of the mice. These results indicate that the dual-targeting drug-loaded liposomes show a long circulatory effect in the blood system, and could effectively accumulate in brain glioma sites in nude mice.
Figure 9. In vivo real-time imaging observation after intravenous administration of the varying formulations.
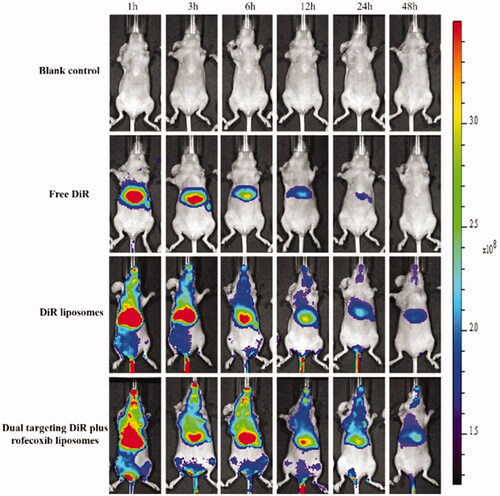
The Kaplan–Meier survival curves of brain glioma-bearing nude mice were obtained by recording the survival days of each mouse after inoculation (). Results exhibited that the mice treated with dual-targeting daunorubicin plus rofecoxib liposomes had the longest survival time, exhibiting the strongest efficacy as compared to the control formulations in the brain glioma-bearing nude mice. The toxicity to the major organs was evaluated by using H&E staining their paraffin sections. As compared to the blank control, dual-targeting daunorubicin plus rofecoxib liposomes did not cause obvious damage to the major organs, which were consisted of heart, liver, spleen, lung and kidney (). The preliminary evaluation indicates that dual-targeting daunorubicin plus rofecoxib liposomes could be rather safety and efficacy in vivo.
Conclusions
The developed dual-targeting daunorubicin plus rofecoxib liposomes could effectively inhibit brain glioma VM channels and show significant efficacy on intracranial glioma-bearing nude mice. The evaluation results reveal that the nanoscaled dual targeting daunorubicin plus rofecoxib liposomes are able to transport across the BBB, accumulate more in the brain glioma region, have been targeted uptake by brain glioma cells, and then kill brain glioma cells along with eliminating VM channels. The action mechanisms are related to the downregulations of MMP-2, MMP-9, FAK and HIF-α. It is concluded that the dual targeting daunorubicin plus rofecoxib liposomes could be a promising formulation to treat the brain glioma, and to eliminate their VM channels.
Disclosure statement
No potential conflict of interest was reported by the author(s).
Additional information
Funding
References
- Tang W, Fan W, Lau J, et al. Emerging blood–brain-barrier-crossing nanotechnology for brain cancer theranostics. Chem Soc Rev. 2019;48(11):2967–3014.
- Weil RJ, Palmieri DC, Bronder JL, et al. Breast cancer metastasis to the central nervous system. Am J Pathol. 2005;167(4):913e20.
- Wu JS, Mu LM, Bu YZ, et al. C-type natriuretic peptide-modified lipid vesicles: fabrication and use for the treatment of brain glioma. Oncotarget. 2017;8(25):40906–40921.
- Pollak TA, Drndarski S, Stone JM, et al. The blood–brain barrier in psychosis. Lancet Psychiatry. 2018;5(1):79–92.
- Ju RJ, Zeng F, Liu L, et al. Destruction of vasculogenic mimicry channels by targeting epirubicin plus celecoxib liposomes in treatment of brain glioma. Int J Nanomedicine. 2016;11:1131–1146.
- Gao Y, Yu H, Liu Y, et al. Long non-coding RNA HOXA-AS2 regulates malignant glioma behaviors and vasculogenic mimicry formation via the MiR-373/EGFR axis. Cell Physiol Biochem. 2018;45(1):131–147.
- Liu Y, Mei L, Yu Q, et al. Multifunctional tandem peptide modified paclitaxel-loaded liposomes for the treatment of vasculogenic mimicry and cancer stem cells in malignant glioma. ACS Appl Mater Interfaces. 2015;7(30):16792–16801.
- Liu JJ, Tang W, Fu M, et al. Development of R8 modified epirubicin-dihydroartemisinin liposomes for treatment of non-small-cell lung cancer. Artif Cells Nanomed Biotechnol. 2019;47(1):1947–1960.
- Belhadj Z, Ying M, Cao X, et al. Design of Y-shaped targeting material for liposome-based multifunctional glioblastoma-targeted drug delivery. J Control Release. 2017;255:132–141.
- Ying X, Wen H, Lu WL, et al. Dual-targeting daunorubicin liposomes improve the therapeutic efficacy of brain glioma in animals. J Control Release. 2010;141(2):183–192.
- Pang X, Wang T, Jiang D, et al. Functionalized docetaxel-loaded lipid-based-nanosuspensions to enhance antitumor efficacy in vivo. Int J Nanomedicine. 2019;14:2543–2555.
- Perli G, Pessoa ACSN, Balbino TA, et al. Ionic strength for tailoring the synthesis of monomodal stealth cationic liposomes in microfluidic devices. Colloids Surf B Biointerfaces. 2019;179:233–241.
- Cai D, Gao W, He B, et al. Hydrophobic penetrating peptide PFVYLI-modified stealth liposomes for doxorubicin delivery in breast cancer therapy. Biomaterials. 2014;35(7):2283–2294.
- Ju RJ, Li XT, Shi JF, et al. Liposomes, modified with PTD(HIV-1) peptide, containing epirubicin and celecoxib, to target vasculogenic mimicry channels in invasive breast cancer. Biomaterials. 2014;35(26):7610–7621.
- Kolesnik DL, Pyaskovskaya ON, Yakshibaeva YR, et al. Time-dependent cytotoxicity of dichloroacetate and metformin against Lewis lung carcinoma. Exp Oncol. 2019;41(1):14–19.
- Li XY, Zhao Y, Sun MG, et al. Multifunctional liposomes loaded with paclitaxel and artemether for treatment of invasive brain glioma. Biomaterials. 2014;35(21):5591–5604.
- Zhang F, Zhang CM, Li S, et al. Low dosage of arsenic trioxide inhibits vasculogenic mimicry in hepatoblastoma without cell apoptosis. Mol Med Rep. 2018;17(1):1573–1582.
- Zhao M, Zhao M, Fu C, et al. Targeted therapy of intracranial glioma model mice with curcumin nanoliposomes. Int J Nanomedicine. 2018;13:1601–1610.
- Angelova A, Garamus VM, Angelov B, et al. Advances in structural design of lipid-based nanoparticle carriers for delivery of macromolecular drugs, phytochemicals and anti-tumor agents. Adv Colloid Interface Sci. 2017;249:331–345.
- Zerkoune L, Lesieur S, Putaux JL, et al. Mesoporous self-assembled nanoparticles of biotransesterified cyclodextrins and nonlamellar lipids as carriers of water-insoluble substances. Soft Matter. 2016;12(36):7539–7550.
- Mayer LD, Tardi P, Louie AC. CPX-351: a nanoscale liposomal co-formulation of daunorubicin and cytarabine with unique biodistribution and tumor cell uptake properties. Int J Nanomedicine. 2019;14:3819–3830.
- Punganuru SR, Madala HR, Mikelis CM, et al. Conception, synthesis, and characterization of a rofecoxib-combretastatin hybrid drug with potent cyclooxygenase-2 (COX-2) inhibiting and microtubule disrupting activities in colon cancer cell culture and xenograft models. Oncotarget. 2018;9(40):26109–26129.