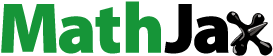
Abstract
Inhalation of silicon dioxide (SD) results in pulmonary inflammatory responses and fibrosis. Isorhynchophylline (Isorhy) is the main alkaloid in the traditional Chinese herb Tripterygium wilfordii, which is reported to have anti-inflammatory activities in the nervous system. However, the effects of Isorhy on SD-induced pulmonary inflammation and fibrosis in mice are unknown. Male mice were exposed to a single dose of SD (2.5 mg/kg, intranasal inoculation) to induce pulmonary fibrosis (PF). The mice were woken up and immediately treated with Isorhy (20 mg/kg, intraperitoneal injection) for 14 or 42 days. The effects of Isorhy on inflammatory responses and lung fibrosis induced by SD were then investigated. After the 14-day treatment, there was a significant reduction in inflammatory cell infiltration in the lungs of mice, with reduced recruitment of inflammatory cells to the lungs. The concentration of pro-inflammatory factors in the bronchoalveolar lavage fluid was reduced, which alleviated inflammatory injury in the lung tissue. After the 42-day treatment, Isorhy alleviated inflammation and inhibited the release of fibrogenic factors in mice with PF. Isorhy also significantly reduced collagen deposition in the lung tissues of mice. Isorhy has the ability to reduce inflammatory responses and fibrosis associated with SD-induced acute lung injury.
Introduction
Pulmonary fibrosis (PF) is a chronic, devastating and lethal fibrotic disorder affecting the human lung [Citation1]. Early characteristics of PF include respiratory dysfunction with destruction of the alveolar capillary membrane, infiltration of peripheral inflammatory cells, and release of several cytokines (i.e. inflammation stage) [Citation2–4]. As the disease progresses, it is characterized by worsening pulmonary function and persistent alteration of the lung parenchyma. This results from fibrotic foci formation by activated fibroblasts/myofibroblasts and excessive production and deposition of extracellular matrix (ECM) components (i.e. fibrosis stage) [Citation5,Citation6]. Fibrotic diseases account for up to 45% of deaths in the developed world [Citation7]. To date, few drugs have been approved for reversing fibrosis or preventing its progression; however, they have limited efficacy. Therefore, new pharmaceuticals are needed for managing the condition.
Exposure to silica can cause respiratory diseases characterized by inflammatory and/or fibrotic changes [Citation8]. A previous study showed that neutrophilic inflammation is induced as early as five hours after intratracheal administration of silica in rats [Citation9]. Acute inflammation induced by silica is the earliest known reaction of the immune system.
Inhaled silica particles are engulfed by alveolar macrophages, leading to the activation of innate immune response [Citation10]. Activated macrophages release inflammatory cytokines such as interleukin (IL)-1β and tumour necrosis factor (TNF)-α, which recruit more monocytes/macrophages [Citation11,Citation12]. This leads to the initiation of lung injury and the lengthy process of silica-induced effects such as fibrosis. These findings indicate that silica is a strong inflammatory agent and can induce an overt and rapid inflammatory response. Acute inflammatory reactions that are induced by lipopolysaccharide and bacterial infections resolve quickly. Additionally, the structure and function of the affected tissue or organ can return to normalcy [Citation13]. However, silica-induced pulmonary inflammation persists and adversely affects normal lung structure and function. Furthermore, under the influence of bioactive factors, fibroblasts proliferate and produce collagen fibres to stimulate the development of PF [Citation14].
Lung injury induced by toxic compounds depends on specific interactions that lead to inflammation and overreaction. Therefore, anti-inflammatory treatments against lung injury induced by toxic chemicals are required [Citation15]. The ideal treatment for silica-induced lung injury should have both anti-inflammatory and anti-fibrotic effects. Isorhynchophylline (Isorhy) is the major alkaloid in Uncaria rhynchophylla (Miq.) Miq. ex Havil. (family Rubiaceae Juss) with multiple pharmacological effects [Citation16]. It is widely used to treat cardiovascular and nervous system diseases such as hypertension, diabetes, tumours, asthma, stroke, platelet accumulation and thrombus formation [Citation17]. It also inhibits inflammatory reactions and induces apoptosis of nerve cells [Citation18]. Furthermore, it inhibits oxidative stress and endothelial cell proliferation [Citation19]. However, it is not clear whether Isorhy can inhibit inflammation in the pulmonary system. Persistent inflammation in the lungs can lead to fibrosis; therefore, there is a need to investigate whether Isorhy can inhibit pulmonary inflammation and prevent the development of PF.
In this study, we investigated the protective effects of Isorhy against silicon dioxide (SD)-induced lung injury and the potential underlying mechanisms of action in mice. This study shows the development of therapeutic agents for PF.
Materials and methods
Experimental animals
Adult male Kunming mice weighing 25–30 g (license number, SCXK 20160002) were purchased from SPF Biotechnology Co., Ltd. (Peking, China) for the study. The procedures for the care and use of the animals were approved by the Ethics Committee of Laboratory Animals of Baotou Medical College (Baotou, China). The mice were housed in plastic cages under standard conditions (25 ± 1 °C, 12/12-h light–dark cycle) and had free access to water and food.
Chemicals
Isorhy (batch number, 111927-201804; CAS number, 6859-01-4; molecular formula, C22H28N2O4) was purchased from the National Institutes for Food and Drug Control (Beijing, China) and dissolved in saline at a concentration of 2 mg/mL before it was administered to the mice. SD (lot, C000342814; purity, 99.99%; granulate, 0.2–0.7 mm) was purchased from Umicore (Brussels, Belgium) and suspended in saline at a concentration 50 mg/mL before use.
Development of PF and drug treatments
A mouse model of PF was established as previously described with some modifications [Citation20]. In this experiment, a total of 60 mice were randomized into four groups: control (saline), Isorhy, SD and SD + Isorhy, with each group containing 15 animals. The mice were anaesthetized with 1% pentobarbital sodium (10 mL/kg body weight) via intraperitoneal injection. The control mice were allowed to breathe only room air. Mice in the SD and SD + Isorhy groups received a single dose of SD (2.5 mg/kg) via intranasal inoculation following the anaesthesia. Isorhy was administered at 20 mg/kg to mice in the SD + Isorhy and Isorhy groups by intraperitoneal injection. The mice in the SD + Isorhy group were administered Isorhy immediately after waking up following the intranasal inoculation of SD. Body weight was continuously recorded for 14 or 42 days.
The survival rate of the animals was first studied using 40 mice that were divided in such a way that there were 10 animals in each group, and five remainder mice per group. The number of mice that died was recorded, and a survival rate curve was subsequently plotted. Since there were variations among the groups with respect to the number of animal deaths, the five remainder mice of each group were included in the corresponding experimental groups for increasing the number of animals in each of the experimental groups to 10. All the other experiments were subsequently performed, with the exception of survival rate determination. In case there were any remainder mice after the number of animals in the experimental group had reached 10, the remainder mice were not considered for experimentation. Thus, if there were 10 mice per group, five were sacrificed for the experiment on day 14 following SD intranasal inoculation, and the remaining five mice were sacrificed on day 42 of SD intranasal inoculation.
Body weight and lung index analysis
Body weight was used to evaluate growth, whereas lung index reflected the degree of inflammatory and fibrosis in the lungs of the mice. Body weight and lung index were analysed on days 14 and 42. Lung index was calculated as follows:
Sample collection
After anaesthesia was induced, cardiac perfusion was performed to obtain blood-free pulmonary samples, after which the left lung was ligated. Lavage was performed with 2 mL saline from the bronchus to the right lung to obtain bronchoalveolar lavage fluid (BALF). The BALF samples were centrifuged at 3000 rpm for 10 min. Cell-free supernatants were stored at −80 °C and analysed to detect the expression levels of selected biomarkers by enzyme-linked immunosorbent assay (ELISA). Cell pellets at the bottom of each centrifuge tube were resuspended in 100 μL saline for differentiation using a light microscope (Olympus, Tokyo, Japan). The left and right lungs were then removed and weighed. The left lungs were stored at −80 °C and analysed for their hydroxyproline (HYP) content, whereas the right lungs were fixed in 4% paraformaldehyde for histological examination immediately.
Histological examination
The right lungs were processed as previously described. The paraffin sections were stained with haematoxylin and eosin (H&E) for the evaluation of inflammation and fibrosis under a light microscope. Inflammation [Citation21] and tissue fibrosis [Citation22] were evaluated and scored as follows by a pathologist who was blinded to the animal groups.
Tissue inflammation [Citation21]
0: without inflammation;
1: random location of inflammatory cells;
2: major portions of the bronchi and veins surrounded by a thin layer of inflammatory cells (thickness of 1–5 cells);
3: major portions of the bronchi and veins surrounded by a thick layer of inflammatory cells (a thickness of >5 cells);
4: complete pulmonary inflammation around all veins and bronchi.
Tissue fibrosis [Citation22]
0: normal pulmonary tissue;
1: minimum thicknesses of alveoli and bronchiole walls;
2–3: medium thicknesses of alveoli and bronchiole walls without significant damage to lung structure;
4–5: increase in fibrosis with significant damage to lung structure and formation of small clumps of fibrotic tissue;
6–7: severe destruction of lung structure, large areas of fibrosis and “honeycomb lung”;
8: total fibrosis of lung.
Assessment of inflammatory cell counts in BALF
The resuspended BALF samples were pelleted onto glass slides by cytocentrifugation (Cytospin 4; Thermo Fisher Scientific, Waltham, MA) at 800 rpm for 10 min, followed by differentiation by light microscopy. The glass slides were then subjected to Giemsa staining (Sigma-Aldrich, St. Louis, MO). Differential cell counts were estimated based on standard morphological criteria and calculated as percentages of the total number of cells that were the various cell types. For monocyte/macrophage quantification, data were acquired by counting 400 monocytes and macrophages per slide. Neutrophil/macrophage/lymphocyte quantification analysis was done by counting 500 of all cell types per slide.
Total collagen in lung tissue and cytokine levels in BALF
Collagen deposition was estimated by measuring HYP content in the left lung tissue. The collagen content per 500 mg lung tissue was measured using a commercial ELISA kit (MyBioSource, San Diego, CA) according to the manufacturer’s instructions. The levels of IL-1β, IL-6, TNF-α, platelet-derived growth factor (PDGF)-BB and transforming growth factor (TGF)-β1 in BALF were measured with an ELISA kit (R&D Systems, Minneapolis, MN) according to the manufacturer’s instructions. Assays were performed in duplicate wells and the mean of the data was determined for each sample. Absorbance was measured at 450 nm with a microplate reader (Thermo Fisher Scientific, Waltham, MA). The data obtained were used to construct standard curves to calculate the levels of the various cytokines in each sample.
Statistical analysis
Data are presented as mean ± standard error of the mean. Statistical significance was assessed by parametric methods using two-way analysis of variance. p Values<.05 were considered statistically significant. Statistical analysis was carried out using GraphPad Prism software (version 6.01; GraphPad Software Inc., San Diego, CA).
Results
Condition of the mice after exposure to SD
There were no significant changes in the mental state and fur of the control and Isorhy groups from day 0 to 14. However, the appetite and autonomous activities of mice exposed to SD significantly decreased. The furs were dull and lustrous, accompanied by continuous weariness, weak breathing and occasional choke. The Isorhy-treated mice showed significant improvement in mental state. Additionally, their appetite and autonomous activities increased, choking hardly occurred, and the furs did not show any marked difference.
On days 15–42, the mental state and fur of the control and Isorhy mice were normal. Some mice in the SD group still showed mental weariness, loss of appetite, and decreased activity; however, there was no choking or obvious abnormality in their fur. The mental state, fur and appetite of mice in the SD + Isorhy groups were normal.
Isorhy improves survival rate, body weight and lung index in SD-treated mice
The mice were treated with 20 mg/kg Isorhy (chemical structure is shown in ) by intraperitoneal injection daily after SD intranasal inoculation (). The highest number of deaths was recorded on the day the mice were exposed to SD. The percent survival was almost 60% initially; however, on the seventh day, the percent survival dropped to approximately 50% and was maintained until the animals were sacrificed (). The percent survival of the SD + Isorhy mice was significantly better than that of the mice treated with SD only. No death was recorded on the day of exposure to silica; however, the final percent survival was nearly 70%.
Figure 1. Isorhy improves the percent survival and reduces weight loss and the lung index in mice with silicon dioxide-induced pulmonary fibrosis. (A) Intervention dosing regimen of Isorhy in the experimental mouse model of pulmonary fibrosis. (B) Chemical structure of Isorhy. (C) Mouse body weight at different time points. (D) The percent survival in mice. (E) Mouse lung index.
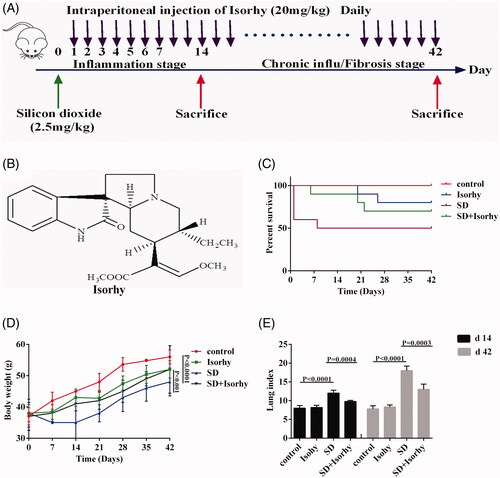
Changes in body weight were measured to assess SD-induced pulmonary toxicity. The body weights of the SD-treated mice significantly decreased within seven days after the PF model was developed; however, the body weights kept increasing from the seventh day. The final body weight was markedly lower in the SD group than in the control group (p < .0001). Although the body weight of mice in the SD + Isorhy group was significantly higher than that of mice in the SD group (p < .001), it was lower than that of control group mice. This suggests that Isorhy improved the body weight loss induced by SD. Body weight changes were normal in the control mice and mice treated with only Isorhy ().
The lung index was determined to confirm whether Isorhy alleviates SD-induced PF in mice. The data showed that the lung index was significantly higher in the SD group than in the control group on days 14 and 42 days after SD exposure (p < .0001); however, it was significantly lower in the Isorhy-treated group than in the SD group (p < .001). There was no difference in the lung index between the control group and the Isorhy-only group ().
Isorhy attenuates SD-induced pulmonary inflammation and fibrosis
The lung tissue structure was complete with no thickening of the alveolar septum in the control and Isorhy-only group. However, the alveoli of mice treated with only SD were severely damaged. Additionally, the alveolar septum was significantly widened with high inflammatory cell infiltration on day 14 and fibroblast proliferation and focal aggregation on day 42 (). Additionally, inflammation and fibrosis scores were significantly higher in the SD group than in the control group (p < .0001, ). Importantly, these SD-induced effects were significantly alleviated on days 14 or 42 by Isorhy (p < .01 and <.0001, respectively). These findings indicate that Isorhy may be an effective treatment for SD-induced PF.
Figure 2. Isorhy ameliorates silicon dioxide-induced pulmonary inflammation and fibrosis in mice. (A, B) The low (10 × 20) and high magnification (10 × 40) images of the tissues, demonstrating the changes in tissue structure and cellular morphology by 14 and 42 days, respectively. (C, D) The inflammation scores and fibrosis scores at different time points. Data presented are mean ± SEM of five mice per group.
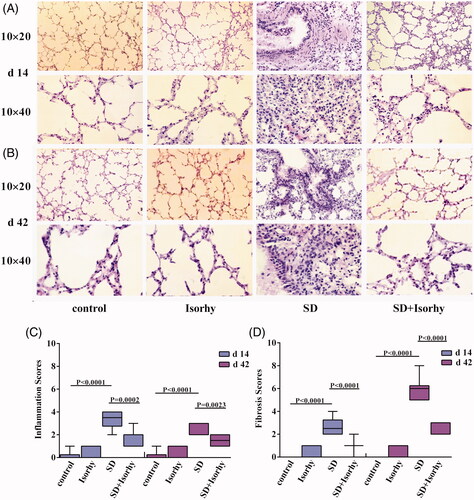
Anti-fibrotic effects of Isorhy are associated with decreased accumulation of inflammatory cells
On day 14, significant lung inflammation and injury were apparent in the mice, which were evidenced by an increase in cellularity (). SD induced inflammatory cell recruitment (mainly neutrophils and lymphocytes) to the alveoli (; p < .0001 and .05, respectively). Additionally, the percentage of macrophages in the mononuclear/macrophage system was significantly higher in the mice treated with only SD than in the control group (p < .0001, ). However, after the 14-day treatment, Isorhy significantly reduced the proportion of inflammatory cells, which had been increased by SD (p < .05, ).
Figure 3. Effects of Isorhy on inflammatory cell accumulation in the bronchoalveolar lavage fluid samples of mice with silicon dioxide-induced pulmonary fibrosis. (A) Giemsa-stained images of the bronchoalveolar lavage fluid cells from different groups of mice by 14 and 42 days (10 × 100). (B) The percentages of monocytes and macrophages by 14 and 42 days. (C) Cell counts. Data presented are mean ± SEM of five mice per group.
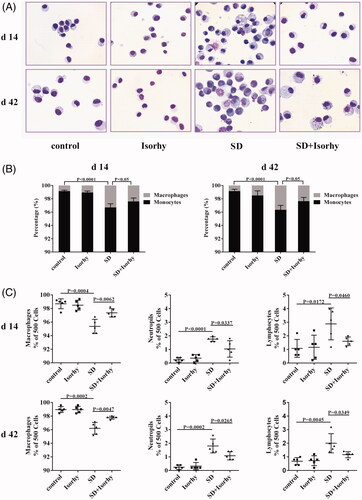
The number of inflammatory cells in the BALF remained elevated 42 days after the treatment with SD. Neutrophil and lymphocyte counts were approximately ninefold and twofold higher, respectively, in the SD group than in the control group (p < .01). Consistent with the changes in the monocyte/macrophage system on day 14, the percentage of macrophages in the SD group were still significantly increased on this day (p < .0001). Nevertheless, Isorhy notably reduced neutrophil and lymphocyte counts and the percentage of macrophages in the monocyte/macrophage system (p < .05, ). Moreover, there was no effect on inflammatory cells on day 14 and 42 in the Isorhy-only group. These results indicate that Isorhy effectively suppresses SD-induced inflammatory cell accumulation in the early stage of inflammation and in the late stage of chronic inflammation and fibrosis.
Isorhy delays the progression of SD-induced PF
To investigate the anti-fibrotic effects of Isorhy and the mechanisms underlying them, cytokine levels in the BALF were analysed. There was a significantly higher expression of IL-1β, TNF-α, IL-6, PDGF-BB and TGF-β1 in the group exposed to SD alone than in the control group (p < .01, –E)). On day 14 after exposure to SD, the expression levels of IL1β and TNF-α were the highest, indicating that pulmonary inflammation was high at this time. On day 42, the expression levels of IL-6, TGF-β1 and PDGF-BB were significant, which indicated continued inflammation and a significant change in fibrosis. Isorhy markedly reduced the expression levels of the cytokines in the BALF (p < .05).
Figure 4. Effects of Isorhy on the expression levels of selective biomarkers in the bronchoalveolar lavage fluid samples of mice with silicon dioxide-induced pulmonary fibrosis. (A–E) ELISA for interleukin (IL)-1β, tumour necrosis factor (TNF)-α, interleukin (IL)-6, platelet-derived growth factor (PDGF)-BB and transforming growth factor (TGF)-β1 standard curve and quantification, respectively, in bronchoalveolar lavage fluid collected 14 and 42 days after intranasal inoculation. (F) ELISA for HYP assay to quantify standard curve and collagen content in the lungs of mice 14 and 42 days after intranasal inoculation. Data presented are mean ± SEM of five mice per group.
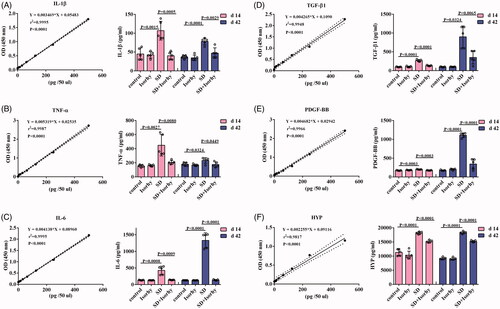
We measured HYP content in the lung tissues as it is a key indicator of PF. On days 14 and 42, there were significant increases in HYP levels in the lung tissues of mice treated with only SD (p < .0001, ); however, Isorhy reduced SD-induced increase in HYP levels (p < .0001).
Discussion
The pathogenesis of PF has not been fully elucidated, and a few treatment options are available. At present, it is believed that PF is mainly due to persistent inflammation causing injury to the pulmonary epithelium. Here, we showed that intraperitoneal injection of Isorhy ameliorated SD-induced PF in mice. We also analysed its effects on inflammatory responses and sequent fibrosis (). A large number of cytokines are secreted in response to inflammation in the lung, and the secreted growth factors promote the recruitment and differentiation of mesenchymal precursor cells into myofibroblasts, which produce large amounts of collagen. Two phases were noted in the development of PF in mice that were treated with SD. During the first 14 days after treatment, inflammation was triggered and observed to progress; however, fibrosis was detected with time. The level of inflammation remained the same after 15 days; however, fibrosis continued to develop for up to 42 days [Citation23,Citation24]. Pathological changes in PF include a decreased number of alveolar epithelial cells, infiltration of the lungs with inflammatory cells, excessive proliferation of fibroblasts, and collagen deposition.
In the present study, we observed enhanced inflammation and fibrosis in the lungs of SD-treated mice. Additionally, we noted destruction of lung architecture, recruitment and accumulation of inflammatory cells, and changes in the appearance and number of fibroblasts. The fibroblasts showed focal aggregation and the formation of fibrous foci, indicating that SD-induced inflammation does not completely resolve but occurs throughout PF. In addition, the lung index, and index reflecting the degree of PF, increases [Citation25]. During PF development, the lung weight of mice exposed to SD increased significantly due to inflammatory cell infiltration, cell swelling and collagen deposition. Owing to disease progress, the weight of the mice increased slowly, and the lung index of mice showed a significant increase [Citation26]. However, these changes were significantly alleviated with Isorhy treatment.
Once the epithelial cells in the lungs are injured, monocytes are recruited to the inflammatory site and they differentiate into macrophages. Macrophages are important innate immune cells and the first-line defence against invading foreign bodies. They also orchestrate structural destruction of the lung tissue. Consequently, the number of macrophages is positively correlated with early lung inflammation and the degree of fibrosis [Citation27,Citation28]. Following lung injury, activated macrophages release cytokines such as TNF-α, IL-8, IL-6 and IL-1β [Citation10, Citation29]. Together with activated macrophages, these cytokines induce the recruitment and aggregation of several cells including neutrophils, lymphocytes and eosinophils [Citation30,Citation31]. The increase in neutrophil count is another important molecular mechanism of fibrosis. Therefore, reducing the numbers of macrophages and neutrophils can effectively control the degree of inflammation and delay PF progression following lung injury. On days 14 and 42 after exposure to SD, the amounts of macrophages, neutrophils and lymphocytes in BALF significantly increased. This suggests that SD induced acute and chronic inflammation and fibrosis in the lungs of the mice. Additionally, inflammation occurred throughout the process. Nevertheless, Isorhy significantly decreased the amounts of macrophages, neutrophils and lymphocytes in BALF and improved the histological features of the lung tissue. This suggests that the inhibitory effect of Isorhy on SD-induced PF in mice involves reduction in the amounts of macrophages and neutrophils to alleviate inflammation.
The expression levels of TNF-α, IL-1β and IL-6 in BALF were measured to determine if Isorhy has an anti-fibrotic effect. Macrophages in the lung secrete cytokines that regulate fibroblast recruitment, proliferation and activation; TNF-α and IL-1β are the earliest factors among these cytokines [Citation10, Citation29]. IL-1 (mainly composed of IL-1α and IL-1β) is an important factor in inflammation and fibrosis. Its expression is high in epithelial and endothelial cells; however, IL-1β is mainly secreted by monocytes and macrophages. Some other cells, such as fibroblasts, T cells, neutrophils and bronchoalveolar epithelial cells, can also produce IL-1. A high IL-1β expression in the mouse lung can lead to pulmonary inflammation [Citation32]. Moreover, IL-1β and TNF-α produced by activated alveolar epithelial cells and macrophages induce the formation of lung fibroblasts. TNF-α, secreted by macrophages, also induces an inflammatory response in the lung by promoting cytokine production. Furthermore, it is an early-response cytokine that can influence the generation of chemotactic mediators that are responsible for neutrophil recruitment to the lungs [Citation33]. TNF-α overexpression induces pathological changes such as thickening of the pleural septum and increase in collagen production, which also occur in inflammatory lung disease and PF [Citation34,Citation35]. IL-6 can favour a change of pro-inflammatory classical macrophages into pro-fibrotic alternative macrophages. It can also mediate cell proliferation, growth and differentiation [Citation36–38].
Therefore, neutralization of IL-1β, TNF-α and IL-6 attenuated SD-induced PF [Citation11]. In the present study, SD induced recruitment of inflammatory cells to the lungs and increased the secretion of IL-1β, TNF-α and IL-6 in BALF. However, these cytokine levels were significantly reduced by Isorhy. These results indicate that the anti-fibrotic effect of Isorhy results in reduced cytokine secretion via reduction in the number and recruitment of inflammatory cells.
We also studied the levels of fibrogenic factors, particularly TGF-β, in SD-induced PF. TGF-β participates in the formation of internal and external stroma. Furthermore, it induces inflammatory cell chemotaxis to lesion sites, promotes the proliferation and differentiation of fibroblasts, promotes the synthesis of ECM, produces collagen and fibronectin, inhibits collagen degradation, and promotes fibrosis [Citation39,Citation40]. PDGF is another crucial factor for fibrogenesis. Its target cells include fibroblasts, macrophages and leucocytes. It induces fibroblast recruitment and proliferation. Several studies have indicated that macrophage-derived PDGF works with TGF-β1 in the development of lung, liver and renal fibrosis [Citation41,Citation42]. Consistent with previous findings, the expression levels of TGF-β1 and PDGF-BB were significantly upregulated in the SD-treated mice; however, they were significantly reduced by Isorhy, which suggests that Isorhy can delay PF development. Collagen contains a high level of HYP (a component of the ECM); however, the ECM also affects the structure of normal pulmonary tissue [Citation43]. Therefore, HYP concentration in the lungs is indicative of collagen deposition and the severity of PF. Collagen level was significantly lower in the SD + Isorhy group than in the SD group, which is suggestive of the protective role of Isorhy against SD-induced PF.
We observed that SD elicits lung injury characterized by the activation of inflammatory signalling and increased collagen production. Additionally, Isorhy protects against SD-induced PF mainly by inhibiting inflammatory responses. Furthermore, it inhibits collagen deposition and the release of fibrogenic factors to delay the progression of PF.
Disclosure statement
No potential conflict of interest was reported by the author(s).
Additional information
Funding
References
- Oku H, Shimizu T, Kawabata T, et al. Antifibrotic action of pirfenidone and prednisolone: different effects on pulmonary cytokines and growth factors in blemomycin-induced murine pulmonary fibrosis. Eur J Pharmacol. 2008;590(1–3):400–408.
- Kim HR, Shin DY, Chung KH. A review of current studies on cellular and molecular mechanisms underlying pulmonary fibrosis induced by chemicals. Environ Health Toxicol. 2018;33(3):e2018014.
- Inchingolo R, Condoluci C, Smargiassi A, et al. Are newly launched pharmacotherapies efficacious in treating idiopathic pulmonary fibrosis? Or is there still more work to be done? Expert Opin Pharmacother. 2017;18(15):1583–1594.
- Loomis-King H, Flaherty KR, Moore BB. Pathogenesis, current treatments and future directions for idiopathic pulmonary fibrosis. Curr Opin Pharmacol. 2013;13(3):377–385.
- Fernandez IE, Eickelberg O. New cellular and molecular mechanisms of lung injury and fibrosis in idiopathic pulmonary fibrosis. Lancet. 2012;380(9842):680–688.
- Tzouvelekis A, Bonella F, Spagnolo P. Update on therapeutic management of idiopathic pulmonary fibrosis. Ther Clin Risk Manag. 2015;11:359–370.
- Oruqaj G, Karnati S, Vijayan V, et al. Compromised peroxisomes in idiopathic pulmonary fibrosis, a vicious cycle inducing a higher fibrotic response via TGF-β signaling. Proc Natl Acad Sci USA. 2015;112(16):E2048–E2057.
- Li W, Xie L, Ma J, et al. Genetic loss of Gas6/Mer pathway attenuates silica-induced lung inflammation and fibrosis in mice. Toxicol Lett. 2019;313:178–187.
- Yuen IS, Hartsky MA, Snajdr SI, et al. Time course of chemotactic factor generation and neutrophil recruitment in the lungs of dust-exposed rats. Am J Respir Cell Mol Biol. 1996;15(2):268–274.
- Rimal B, Greenberg AK, Rom WN. Basic pathogenetic mechanisms in silicosis: current understanding. Curr Opin Pulm Med. 2005;11(2):169–173.
- Guo J, Gu N, Chen J, et al. Neutralization of interleukin-1 beta attenuates silica-induced lung inflammation and fibrosis in C57BL/6 mice. Arch Toxicol. 2013;87(11):1963–1973.
- Leung CC, Yu IT, Chen W. Silicosis. Lancet. 2012;379(9830):2008–2018.
- Zhou Z, Su Y, Fa XE. Isorhynchophylline exerts anti-inflammatory and anti-oxidative activities in LPS-stimulated murine alveolar macrophages. Life Sci. 2019;223:137–145.
- Chen S, Cui G, Peng C, et al. Transplantation of adipose-derived mesenchymal stem cells attenuates pulmonary fibrosis of silicosis via anti-inflammatory and anti-apoptosis effects in rats. Stem Cell Res Ther. 2018;9(1):110.
- Wigenstam E, Elfsmark L, Ågren L, et al. Anti-inflammatory and anti-fibrotic treatment in a rodent model of acute lung injury induced by sulfur dioxide. Clin Toxicol. 2018;56(12):1185–1194.
- Zhou JY, Zhou SW. Isorhynchophylline: a plant alkaloid with therapeutic potential for cardiovascular and central nervous system diseases. Fitoterapia. 2012;83(4):617–626.
- Zhang WB, Chen CX, Sim SM, et al. In vitro vasodilator mechanisms of the indole alkaloids rhynchophylline and isorhynchophylline, isolated from the hook of Uncaria rhynchophylla (Miquel). Naunyn Schmiedebergs Arch Pharmacol. 2004;369(2):232–238.
- Xian YF, Ip SP, Li HQ, et al. Isorhynchophylline exerts antidepressant-like effects in mice via modulating neuroinflammation and neurotrophins: involvement of the PI3K/Akt/GSK-3β signaling pathway. FASEB J. 2019;33(9):10393–10408.
- Yuan D, Ma B, Yang JY, et al. Anti-inflammatory effects of rhynchophylline and isorhynchophylline in mouse N9 microglial cells and the molecular mechanism. Int Immunopharmacol. 2009;9(13–14):1549–1554.
- Shoeb M, Mustafa GM, Joseph P, et al. Initiation of pulmonary fibrosis after silica inhalation in rats is linked with dysfunctional shelterin complex and DNA damage response. Sci Rep. 2019;9(1):471.
- Sur S, Wild JS, Choudhury BK, et al. Long term prevention of allergic lung inflammation in a mouse model of asthma by CpG oligodeoxynucleotides. J Immunol. 1999;162:6284–6293.
- Ashcroft T, Simpson JM, Timbrell V. Simple method of estimating severity of pulmonary fibrosis on a numerical scale. J Clin Pathol. 1988;41(4):467–470.
- Uchiyama M, Nagao T, Hattori A, et al. Pulmonary alveolar proteinosis in a patient with Behcet's disease. Respirology. 2009;14(2):305–308.
- Noth I, Martinez FJ. Recent advances in idiopathic pulmonary fibrosis. Chest. 2007;132(2):637–650.
- Xu L, Li LC, Zhao P, et al. Total polysaccharide of Yupingfeng protects against bleomycin-induced pulmonary fibrosis via inhibiting transforming growth factor-β1-mediated type I collagen abnormal deposition in rats. J Pharm Pharmacol. 2014;66(12):1786–1795.
- Xin X, Chen C, Hu Y-Y, et al. Protective effect of genistein on nonalcoholic fatty liver disease (NAFLD). Biomed Pharmacother. 2019;117:109047.
- Hamilton RF Jr., Thakur SA, Holian A. Silica binding and toxicity in alveolar macrophages. Free Radic Biol Med. 2008;44(7):1246–1258.
- Huaux F. New developments in the understanding of immunology in silicosis. Curr Opin Allergy Clin Immunol. 2007;7(2):168–173.
- Davis GS, Pfeiffer LM, Hemenway DR. Persistent overexpression of interleukin-1beta and tumor necrosis factor-alpha in murine silicosis. J Environ Pathol Toxicol Oncol. 1998;17(2):99–114.
- Murugan V, Peck MJ. Signal transduction pathways linking the activation of alveolar macrophages with the recruitment of neutrophils to lungs in chronic obstructive pulmonary disease. Exp Lung Res. 2009;35(6):439–485.
- Retamales I, Elliott WM, Meshi B, et al. Amplification of inflammation in emphysema and its association with latent adenoviral infection. Am J Respir Crit Care Med. 2001;164(3):469–473.
- Garofalo R, Mei F, Espejo R, et al. Respiratory syncytial virus infection of human respiratory epithelial cells up-regulates class I MHC expression through the induction of IFN-beta and IL-1 alpha. J Immunol. 1996;157:2506–2513.
- Castro P, Legora-Machado A, Cardilo-Reis L, et al. Inhibition of interleukin-1beta reduces mouse lung inflammation induced by exposure to cigarette smoke. Eur J Pharmacol. 2004;498(1–3):279–286.
- Sullivan DE, Ferris M, Nguyen H, et al. TNF-alpha induces TGF-beta1 expression in lung fibroblasts at the transcriptional level via AP-1 activation. J Cell Mol Med. 2009;13(8B):1866–1876.
- Piguet PF, Collart MA, Grau GE, et al. Tumor necrosis factor/cachectin plays a key role in bleomycin-induced pneumopathy and fibrosis. J Exp Med. 1989;170(3):655–663.
- Saito F, Tasaka S, Inoue K, et al. Role of interleukin-6 in bleomycin-induced lung inflammatory changes in mice. Am J Respir Cell Mol Biol. 2008;38(5):566–571.
- Pantelidis P, Fanning GC, Wells AU, et al. Analysis of tumor necrosis factor-alpha, lymphotoxin-alpha, tumor necrosis factor receptor II, and interleukin-6 polymorphisms in patients with idiopathic pulmonary fibrosis. Am J Respir Crit Care Med. 2001;163(6):1432–1436.
- Qiu Z, Fujimura M, Kurashima K, et al. Enhanced airway inflammation and decreased subepithelial fibrosis in interleukin 6-deficient mice following chronic exposure to aerosolized antigen. Clin Exp Allergy. 2004;34(8):1321–1328.
- Daniels CE, Wilkes MC, Edens M, et al. Imatinib mesylate inhibits the profibrogenic activity of TGF-beta and prevents bleomycin-mediated lung fibrosis. J Clin Invest. 2004;114(9):1308–1316.
- Mauviel A. Transforming growth factor-beta: a key mediator of fibrosis. Methods Mol Med. 2005;117:69–80.
- Bonner JC. Regulation of PDGF and its receptors in fibrotic diseases. Cytokine Growth Factor Rev. 2004;15(4):255–273.
- Allen JT, Spiteri MA. Growth factors in idiopathic pulmonary fibrosis: relative roles. Respir Res. 2002;3:13.
- Li LC, Xu L, Hu Y, et al. Astragaloside IV improves bleomycin-induced pulmonary fibrosis in rats by attenuating extracellular matrix deposition. Front Pharmacol. 2017;8:513.