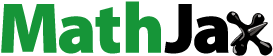
Abstract
In this study, gold nanoparticles (AuNPs) were synthesised using seed extract of mango (Mangifera indica) which is considered as waste and generally thrown away into the environment. The bioactive molecules in the seed act as reducing agent to synthesise AuNPs without using any external agent. The characterisation of green synthesised AuNPs was done using various spectroscopic techniques. Visual colour change from colourless to ruby red colour confirmed the formation of AuNPs which was further confirmed by maximum absorption peak at 550 nm by UV-spectra. Crystalline nature was confirmed by XRD technique while round, triangle and irregular shape and 19.45 nm size was confirmed by TEM and SAED analysis. FTIR analysis confirmed the presence of alcohol or phenol, carboxylic acid, ketones, amines, aromatic amines, aliphatic amines, alkyl halides and alkynes in M. indica seed which were responsible for the reduction of gold to AuNPs. The green synthesised AuNPs were evaluated for their antimicrobial, antioxidant and cytotoxic potential. They showed moderate antibacterial, cytotoxic and dose-dependent antioxidant activity. Seeds of M. indica instead of discarding can be successfully utilised for AuNPs synthesis which can be used as a natural source of antimicrobial, antioxidant and anticancer agent.
Green synthesis of gold nanoparticles (AuNPs) from fruit (Mangifera indica) waste (seed).
Characterisation using various spectroscopic techniques: UV-Vis spectroscopy, Zeta potential, FTIR, XRD and TEM analysis.
Synthesized AuNPs were round, triangle and irregular in shape and 19.45 nm in size.
Antimicrobial activity of AuNPs against 14 microorganisms.
Antioxidant activity of AuNPs in terms of DPPH, SO and ABTS.
Cytotoxic activity against HeLa, MCF-7 and fibroblast normal cell lines.
Highlights
Introduction
Seeds and peels of vegetables and fruits are generally thrown into the environment as waste material causing pollution and their disposal is a major problem; but these parts like any other part of the plant are endowed with phytoconstituents which can be therapeutically exploited [Citation1]. This will solve the problem of waste disposal on one hand and it is the best use of biowaste. Fruit and vegetable peels as a source of antimicrobics are reported by various researchers [Citation2,Citation3]. The biowaste seeds and peels show many promising biological activities like antimicrobial, antioxidant, antidiabetic, hepatoprotective, anti-cancer, anti-inflammatory, wound healing, anti-ulcer, etc [Citation4] and thus can be used for pharmacological or pharmaceutical purposes.
Mangifera india L. belongs to Anacardiaceae family. It is one of the main tropical fruits in the world. All parts of the plant like leaves, bark, fruit peel and flesh, roots, and flowers have many medicinal properties and are traditionally used to treat many diseases and ailments [Citation5]. Infectious diseases and cancer are two major health hazards globally and are one of the leading causes of death. The infectious diseases are dealt with many antibiotics but they are becoming static since multidrug-resistant microorganisms are rising. Antibiotics which were once considered as a boon and worked extraordinally are not working anymore. Cancer too has become a deadly dangerous disease. Treatment options are few and many anticancer drugs in use are of natural origin. The synthetic drugs in use are becoming less popular because of their many side effects, costly and increase in chances of reoccurrence [Citation6]. Considering all these, it is of utmost importance to find newer ways of tackling this urgent, needy problem.
Recently the attention has turned towards nanotechnology. It has revolutionized the new era. Nanoparticles are nothing but particles with a size range of 1–100 nm. The nanoparticles show entirely different properties than the bulk materials from which they are synthesized. Metal nanoparticles like silver, gold, zinc, copper, palladium, etc can be synthesised by various physical, chemical and biological methods. The physical and chemical methods have disadvantages hence biological methods are preferred. Biological methods make use of different parts of the plant like leaf, stem, peel, seed, flower, fruit, root, etc. These parts are rich in a variety of phytoconstituents which act as reducing and stabilizing agent in nanoparticle formation. There is no need of adding any other reducing or stabilizing agent. The medicinal plants themselves are a natural source and act as antimicrobics, antioxidant, anticancer, etc agent and nanoparticles synthesized using them is all the more exhilarating since nanoparticles because of their smaller size, large surface to volume ratio show much more promising activity. The green synthesis is simple, easy, cost-effective, rapid and ecofriendly [Citation7].
Some of the examples of gold nanoparticles (AuNPs) synthesized using different plant parts are Elettaria cardamomum seed [Citation8], Mimosa pudica flower [Citation9], Amomum villosum and Fructus amomi fruit [Citation10], Justicia adhatoda leaf [Citation11], etc. There are many application of AuNPs viz. drug delivery [Citation12], antimicrobial [Citation13], anti-cancer [Citation14], antioxidant and catalytic activities [Citation15], antitumor and anti-inflammatory activity [Citation16], hepatoprotective [Citation17], etc.
In this work, we report perhaps for the first time, synthesis of AuNP from Mangifera indica fruit waste, i.e. seed. Characterization was done using various spectroscopic techniques like UV-Vis, Zeta, XRD, TEM, SAED, FTIR. Further antimicrobial, antioxidant and anticancer activities were evaluated.
Material and methods
Plant material
The fruits of M. indica were purchased from Rajkot, Gujarat, India. The fruits were washed thoroughly with tap water and then peel and pulp was separated; the seeds were manually separated after breaking seed kernel, shade dried at room temperature and homogenized to fine powder and stored in air-tight bottles.
Preparation of seed extract
Mangifera indica seed extract was prepared by decoction extraction method. One gram dry powder was extracted with 100 ml of double-distilled water by boiling for 5 min. The extract was filtered through Whatman filter paper No. 1 and centrifuged at 10,000 rpm for 10 min. The filtrate was used for the synthesis of AuNPs.
Qualitative phytochemical analysis
Qualitative analysis for the detection of phytoconstituents in M. indica seed powder was carried out following the procedure described by Harborne [Citation18].
Synthesis of AuNPs
Aqueous solution of Gold (III) chloride trihydrate (HAuCl4) (1 mM) was prepared and used for the synthesis of AuNPs. About 6 ml of freshly prepared seed extract was added to 40 ml of 1 mM HAuCl4 solution at room temperature (25 °C ± 2 °C) and incubated in dark for 24 h. The nanoparticles solution was purified by repeated centrifugation at 10,000 rpm for 10 min followed by redispersion of the pellet of nanoparticles in acetone. After air drying, the purified nanoparticles were stored at 4 °C for analysis.
Characterization of the synthesized AuNPs
UV-visible spectroscopy
The formation of AuNPs was determined using a UV–Vis spectrophotometer (Shimadzu UV-1601) in 350–750 nm range operated at a resolution of 10 nm. Baseline correction of the spectrophotometer was performed using a blank reference. The reduction of the Au + ions in solution was monitored by periodic sampling of aqueous component and measuring the UV-Vis spectra of the solution.
Zeta potential determination
The Zeta potential of green synthesized AuNPs was evaluated using a Zetasizer Nano ZS (Malvern Instruments Inc.), which measures electrophoretic mobility of nanoparticles using phase analysis light scattering.
Fourier transform infrared spectroscopy (FTIR) analysis
Possible functional groups involved in the synthesis and stabilization of AuNPs was determined by FTIR spectroscopy. The FTIR spectrum was recorded in the range of 400–4000 cm−1 Nicolet IS10 (Thermo Scientific, Waltham, MA). Various modes of vibrations were identified and assigned to determine the different functional groups present in M. indica seed extract.
X-ray diffraction (XRD) analysis
XRD analysis was utilized for the structure determination of green synthesized AuNPs. The formation of AuNPs was determined by an X’Pert Pro X-ray diffractometer (PAN analytical BV) operated at a voltage of 40 kV and a current of 30 mA with Cu Ka radiation in θ–2θ configurations. The crystallite domain size was calculated from the width of the XRD peaks, assuming that they are free from non-uniform strains, using the Scherrer formula. D¼0.94 λ/β cos θ, where D is the average crystallite domain size perpendicular to the reflecting planes, λ is the X-ray wavelength, β is the full width at half maximum (FWHM), and θ is the diffraction angle.
Transmission electron microscopy (TEM) analysis
TEM analysis was done to visualize the shape and size of the green synthesized AuNPs. The sample was dispersed in double-distilled water. A drop of thin dispersion was placed on a “staining mat”. Carbon coated copper grid was inserted into the drop with the coated side upwards. After about 10 min, the grid was removed and air-dried, then screened in JEOL JEM 2100 Transmission Electron Microscope (JOEL Corp, Tokyo, Japan).
Antimicrobial activity
The antimicrobial activity was evaluated against B. cereus (BC)ATCC11778, B. subtilis (BS) ATCC6633, S. aureus (SA)ATCC29737, C. rubrum(CR)ATCC14898, E. coli (EC) NCIM2931, P. aeruginosa (PA) ATCC9027, K. neumoniae (KP) NCIM2719 and S. typhimurium (ST) ATCC23564, C. neoformans (CN) ATCC34664 C. albicans (CA)ATCC2091 and C. glabrata (CG)NCIM3438); three fungal clinical isolates (No.12, 15, 22). The bacteria and fungi were maintained on nutrient agar and MGYP medium (Hi-Media, India) respectively, at 4 °C and subcultured before use. The microorganisms studied are clinically important ones causing several infections, food-borne diseases, spoilages, skin infection and it is essential to overcome them through some active therapeutic agents.
Agar well diffusion assay
In vitro antimicrobial activity was determined by agar well diffusion assay [Citation19]. Five different concentrations (10, 20, 30, 40 and 50 mg/ml) of AuNPs were made in 100% DMSO; 100 μl of different concentrations of nanoparticles was added into the well. DMSO was used as a negative control. Antimicrobial activity was assayed by measuring the diameter of the zone of inhibition formed around the well in millimetres. The experiment was done in triplicate, and the average values were calculated for antimicrobial activity.
Antioxidant activity
Determination of 2, 2-diphenyl-1-picrylhydrazyl (DPPH) free radical scavenging assay
The DPPH free radical scavenging activity of AuNPs was measured by the modified method of McCune and Johns [Citation20]. The reaction mixture (3.0 ml) consisted of 1.0 ml DPPH in methanol (0.3 mM), 1.0 ml methanol and 1.0 ml of different concentrations of AuNPs diluted by methanol, was incubated for 10 min in dark, after which the absorbance was measured at 517 nm using UV-Vis Spectrophotometer (Shimadzu, Japan), against a blank sample. The percentage of inhibition was calculated using the following formula:
where B is the Absorbance of the control (DPPH plus methanol) and A is the absorbance of the sample (DPPH, methanol, plus sample).
Determination of superoxide anion free radical (O2−) scavenging assay
The antioxidant activity of AuNPs was measured by the method described by Robak and Gryglewski [Citation21]. Superoxide radicals were generated by oxidation of NADH and assayed by the reduction of NBT. The reaction mixture (3.0 ml) consisted of 1.0 ml of different concentrations of AuNPs diluted by distilled water, 0.5 ml Tris–HCl buffer (16 mM, pH 7), 0.5 ml nitroblue tetrazolium chloride (NBT) (0.3 mM), 0.5 ml NADH (0.936 mM) and 0.5 ml phenazine methosulphate (PMS) (0.12 mM). The superoxide anion radical generating reaction was started by the addition of PMS solution to the reaction mixture. The reaction mixture was incubated at 25 °C for 5 min and then the absorbance was measured at 560 nm using UV-Vis Spectrophotometer (Shimadzu, Japan), against a blank sample. Percentage of inhibition was calculated using the formula described above.
Determination of 2, 2′-Azino-bis-(3-ethyl) benzothiazoline-6-sulfonic acid (ABTS) radical cation scavenging assay
The ABTS radical cation scavenging activity of AuNPs was measured by the method described by Re et al. [Citation22]. ABTS radical cations were produced by the reaction of ABTS (7 mM) with potassium persulfate (2.45 mM) and incubated the mixture at room temperature in the dark for 16 h. The ABTS working solution obtained was further diluted with methanol to give an absorbance of 0.85 ± 0.20 at 734 nm. 1.0 ml of different concentrations of AuNPs diluted by methanol was added to 3.0 ml of ABTS working solution. The reaction mixture was incubated at room temperature for 4 min and then the absorbance was measured at 734 nm using a UV-Vis Spectrophotometer (Shimadzu, Japan) against a blank sample. Percentage of inhibition was calculated using the formula described above.
In vitro cytotoxic activity
Cancer cell viability of the AuNPs was evaluated by the MTT (3-[4,5-dimethylthiazol-2-yl]-2,5-diphenyltetrazolium bromide) assay [Citation23]. The cell lines uses were Human cervical cancer cell line (HeLa), Breast Cancer cell line (MCF-7) and Fibroblast normal cell line. The cell lines were obtained from National Repository of Animal Cell Culture, National Centre for Cell Sciences (NCCS), Pune, India.
Results and discussion
Synthesis of AuNPs using M. indica seed extract
Synthesis of nanoparticles was visually seen by a colour change in reaction mixture indicating the formation of nanoparticles by M. indica seed extract. Green synthesis of AuNPs, involved the addition of M. indica seed extract to gold chloride trihydrate solution, the synthesis reaction started within few minutes and colour change occurred in the reaction mixture. The formation of AuNPs was preliminarily confirmed by a colour change from colourless to purple red colour solution within 5 min indicating the formation of AuNPs. As the incubation time increased, colour intensity also increased because of more synthesis of AuNPs and finally it turned to dark purple red colour at 24 h (). Such visual colour change indicated the formation of AuNPs which is due to surface plasmon resonance which is a collective excitation and oscillation of the surface electrons present in the surface conduction band of metal nanoparticles. Conversion of colourless reaction mixture to cherry red colour on addition of A. bettzickiana leaf extract indicated the formation of AuNPs [Citation24].
Characterisation of AuNPs
UV-Vis spectrophotometer analysis
Mangifera indica seed extract mediated synthesis of AuNPs was confirmed by UV-vis spectroscopic studies. The absorption spectrum of synthesized AuNPs was found to have a maximum absorption band in the range of 350–750 nm which is due to the surface plasmon resonance of AuNPs with a maximum absorption peak at 550 nm (). This is characteristic nature of noble metal gold and several other researchers showed an absorption peak at 545 and 560 [Citation25,Citation26].
Zeta potential analysis
The particle size distribution and Zeta potential graphs of AuNPs are given in . The Zeta potential of green synthesized AuNPs was −1.98 mV. The Zeta potential of AuNPs synthesized using P. scutellaria leaf extract was −19.6 mV [Citation27] and AuNPs synthesized using N. oleander stem bark extract was −16.3 mV [Citation28]. The charge of the Zeta potential indicates the stability of nanoparticles. Higher the negative value of Zeta potential more is the interparticle repulsion and more is the stability. In this study, the green synthesized particles showed moderate stability.
X-Ray diffraction analysis (XRD)
shows the diffraction pattern of fabricated AuNPs which confirmed the crystalline nature of synthesized AuNPs. XRD pattern showed four distinct diffraction peaks at 2Ø value viz. 38.30, 44.53, 64.73 and 77.70 corresponding to the crystal planes of (111), (200), (220) and (311), respectively (). All the diffraction peaks were indexed to face-centred cubic structure of metal nanoparticles. The observed data are in good agreement with the standard database of Joint Committee on Powder Diffraction Standards JPCDS file No: 04-0784. The results of the X-ray diffraction pattern confirmed the formation of nanoparticles with strong and narrow bands of Bragg peaks and this may be due to the stabilization of the nanoparticles by M. indica seed extract. Saraca asoca leaf extract mediated synthesized AuNPs showed similar diffraction peaks confirming the crystalline nature and cubic structure of synthesized AuNPs [Citation29].
Fourier transform infrared spectroscopy (FTIR)
FTIR spectra of AuNPs showed prominent peaks at 3549.14, 2885.60, 2175.78, 1705.13, 1612.54, 1535.39, 1450.52, 1334.78, 1180.47, 1010.73, 925.86, 871.85, 678.97, 640.39, 547.80, 516.94, 470.65 and 432.07 cm−1 (). The absorption band at 3549.14 cm−1 corresponds to O–H medium stretching of alcohol group. The absorption band at 2885.60 cm−1 corresponds to C–H medium stretching of alkane group. The absorption band at 2175.78 cm−1 corresponds to –C=C– medium stretching of alkyne group. The absorption band at 1705.13 cm−1 corresponds to C=O broad stretching of acid group. The absorption band at 1612.54 cm−1 corresponds to C=C medium stretching of non-conjugated diene group. The absorption band at 1535.39 cm−1 corresponds to N–O medium stretching of nitro group. The absorption band at 1450.52 cm−1 corresponds to C=C medium stretching of aromatic group. The absorption band at 1334.78 cm−1 corresponds to C–F medium stretching of alkyl halide group. The absorption band at 1180.47 cm−1 corresponds to C–N strong stretching of amine group. The absorption band at 1342.50 and 1180.47 cm−1 corresponds to C–N broad stretching of amine group. The absorption band at 1010.73 cm−1 corresponds to C–F broad stretching of alkyl halide group. The absorption band at 925.86 and 871.85 cm−1 corresponds to = C–H broad bending of alkene group. The absorption band at 678.97 cm−1 corresponds to = C–H medium bending of alkene group. The absorption band at 640.39 cm−1 corresponds to C–Cl medium stretching of alkyl halide group. The absorption band at 547.80 and 516.94 cm−1 corresponds to C–Br medium stretching alkyl halide group and corresponds to C–Br strong stretching alkyl halide group. The absorption band at 470.65 and 432.07 cm−1 corresponds to C = N weak stretching of nitrites group.
Plant extracts contain different secondary metabolites which were responsible for synthesis, reducing and stabilising the AuNP formation. Barai et al. [Citation28] attributed the plant secondary metabolites such as polyphenols including flavonoids, steroids, etc for green synthesized AuNPs from stem bark extract of Nerium oleander [Citation30] synthesized AuNPs using Cannabis sativa which exhibited antibacterial, antifungal, anti-inflammatory and anticancer properties and the bioactive molecules like flavonoids, cannabinoids, terpenes and phenols present in the plant were responsible for reducing the metal salt to nanoparticles and stabilizing them. The qualitative phytochemical analysis of M. indica seed powder revealed the presence of phenols, flavonoids, tannins and coumarins while its polar solvent methanol extract and aqueous extract revealed the presence of phenols, flavonoids, tannins, cardiac glycosides, coumarins and anthocyanins ().
Table 1. Qualitative phytochemical analysis of M. indica seed powder and its solvent extracts.
Transmission electron microscopy analysis and selected area electron diffraction analysis (TEM and SAED)
Morphology, particle size and crystalline nature of AuNPs was characterized using TEM and SAED analysis. TEM images of synthesized AuNPs at different magnifications are given in . The size of the synthesized AuNPs was in the range 12–29 nm and average size was 19.45 nm. The AuNPs were predominantly round, triangle and irregular in shape. The SAED pattern of green synthesized AuNPs using M. indica seed extract is given in . The typical characteristic SAED pattern with bright circular rings characteristic to the indexing planes of (111), (200), (220) and (311) was found which indicated the highly crystalline nature of green synthesized AuNPs. AuNPs synthesized using leaf extract of C. sinensis and A. occidentale possessed average particle size of 23.33 and 10–30 nm, respectively [Citation31,Citation32].
Antimicrobial activity
The antibacterial activity of green synthesized AuNPs against Gram-positive, Gram-negative, fungi and fungal clinical isolates is given in . The antibacterial activity was more towards Gram-positive bacteria than Gram-negative bacteria. AuNPs could inhibit almost all the four Gram-positive bacteria at three higher concentrations (). BC was the most susceptible and CR the most resistant Gram-positive bacteria. AuNPs inhibited all the four Gram-negative bacteria at two higher concentrations (). PA was the most susceptible while EC and KP were the most resistant Gram-negative bacteria. All the three fungal strains were inhibited only at the highest concentration (); CG was the most resistant fungal strain. All the three clinical isolates were inhibited at two higher concentrations. On the whole, the antibacterial activity was more than antifugal activity and antibacterial activity towards Gram-positive bacteria was more than that against Gram-negative bacteria.
Figure 6. Antimicrobial activity of AuNPs against Gram-positive bacteria (A), Gram-negative bacteria (B), fungi (C) and clinical isolates (D).
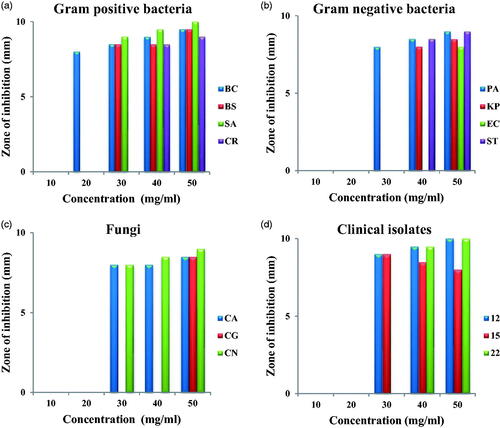
This differential activity is attributed to the different cell wall make-up of Gram-positive and Gram-negative bacteria. The cell wall of Gram-positive bacteria is made up of thick peptidoglycan layer while that of Gram-negative bacteria is thin and made up of lipopolysaccharides which is impermeable to lipophilic substances. Though the exact mechanism of antibacterial action is not very well understood it is suggested that nanoparticles induce morphological changes in cell wall membrane structure thereby disrupt the membrane permeability, disrupt the respiratory function ultimately leading to cell death. They induce the formation of irregular pits on the cellwall; interact with proteins forming complexes with S, N, P and oxygen atoms and cause irreversible damages in the cell wall. Free radicals like OH, SO, HOCL, H2O2, NO, RCOO are also formed which interact with bimolecules like lipids, proteins, enzymes and DNA. Some of the damages caused in DNA are mutations, additions, deletions, single-breaks, double-strand breaks and cross-linking with proteins [Citation33]. The antimicrobial activity of green synthesized Azima tetracantha leaf mediated AuNPs was evaluated against A. liquefaciens, E. fecalis, M. luteus, S. typhimurium and fungal C. albicans, Cryptococcus sp, M. canis, T. rubrum pathogens by Agar well diffusion method. The AuNPs showed maximum activity against S. typhimurium and minimum activity against M. luteus amongst bacterial pathogens while among fungi they were effective against T. rubrum with minimal smaller effect in Cryptococcus sp. A clear concentration effect was found [Citation34]. Nagalingam et al. [Citation24] reported efficient antibacterial activity of AuNPs synthesised using A. bettzickiana leaf extract against B. subtilis, S. aureus, S. typhi, P. aeroginosa, M. luteus, and E. aerogenes by agar well diffusion method. They have attributed the observed antibacterial activity as synergistic effect of the combination of AuNPs and extracts; since chemically synthesised AuNPs did not show antibacterial activity against similar microorganisms [Citation35]. AuNPs synthesised using T. arjuna leaf extract also showed good antibacterial activity against S. aureus, P. aeruginosa and S. typhimurium [Citation36].
Antioxidant activity
The DPPH, SO and ABTS free radical scavenging activity of green synthesized AuNPs at different concentrations is given in . All showed dose-dependent antioxidant activity i.e. as the concentration of nanoparticles increased, % inhibition also increased. In the concentration range of 80–480 µg/ml, an inhibition of 35–96% was envisaged for green synthesized AuNPs and IC50 value was 256 µg/ml for DPPH activity while IC50 value was 555 µg/ml for SO and 62 µg/ml for ABTS. For SO, the % inhibition was 24–78% in the concentration range of 160–960 µg/ml; for ABTS the % inhibition was 22–96% in the concentration range of 20–120 µg/ml. Dose-dependent DPPH antioxidant activity is reported by PV-AuNPs with IC50 value of 165.0 μg/mL [Citation37]. Similar results of AuNPs synthesized using roots of Angelica pubescens was reported by Markus et al. [Citation38]. They have attributed the antioxidant activity to the secondary metabolites like flavonoids, sesquiterpenes, and phenolic compounds present in A. pubescens. ABTS radical scavenging ability of AuNPS synthesized using Gnidia glauca leaf and stem extract is reported by Ghosh et al. [Citation39]. Bakur et al. [Citation40.] also reported dose-dependent ABTS radical scavenging activity of AuNPs. Citrullus lanatus rind mediated synthesized AUNPs showed 29.42% inhibition at 100 μg/ml concentration [Citation25].
In vitro cytotoxic effect
The cytotoxic effect of green synthesied AuNPs was evaluated by MTT assay against human cervical cancer cell line (HeLa), human breast cancer cell line (MCF-7) and human normal cell line. shows the percent viability of HeLa, MCF-7 and normal fibroblast cells exposed to different concentrations (2, 5, 10, 25, 50, 100, 150, 200, 250 and 400 µg/ml) of AuNPs. The % viability of HeLa cells, breast cells and normal cells were in the range of 98–67%, 97–58% and 98–60% when the concentration of synthesized AuNPs was in the range of 2–400 µg/ml, respectively. At low concentration of AuNPs, the % cell viability of HeLa cells, breast cells and normal cells was quite high but as concentration on AuNPs increased, the % cell viability decreased. The standard anticancer drug Mitomycin C was used as positive control which showed 17, 19 and 19% cell viability for HeLa cells, breast cells and normal cells respectively at 400 µg/ml. The synthesized AuNPs showed decreased % cell viability against fibroblast normal cells which indicates that AuNPs showed less cytotoxic effect to normal cells. At 400 µg/ml concentration AuNPs treated HeLa cells showed 67% cell viability; and breast cancer cells showed 58% cell viabilities while normal cells showed 60% cell viability respectively.
Figure 8. In vitro cytotoxicity of AuNPs against HeLa cancer cell line, MCF-7 cell line and normal fibroblast cell line.
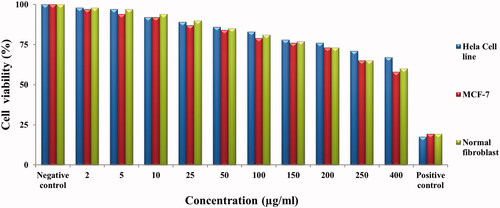
When cancer cells are treated with nanoparticles, the cell morphological characteristics is changed or altered. Rajan et al. [Citation41] reported morphological changes in the cells when treated with different concentration of AuNPs. Chandrasekaran et al. [Citation42] reported that breast cancer cells treated with sliver nanoparticles showed morphological changes such as, cell clumping, cell rupture, inhibition of cell growth and loss of membrane stability. Nanoparticles also induce apoptosis of cell via several apoptotic mechanisms-like generation of ROS, activation caspase-3 cascade, change apoptotic protein expression, cell cycle arrest which resulted in cell growth reduction, chromatin condensation, membrane blebbing and nuclear fragmentation [Citation43]. AuNPs synthesized using Moringa oleifera showed caspase-mediated apoptosis in A549 cells by down regulation of Bcl 2 and activation of caspase-9 and caspase-3/7 [Citation44]. AuNPs synthesized from Panax notoginseng downregulated the expression of Bcl-2, Bcl-xl and upregulated the expression of Bax, caspase-8, caspase-9 and caspase-3 in PANC-1 cells [Citation45]. However, the cytotoxic effect of metal nanoparticles depends on size, shape, type of cells, capping agents and are also dose and time-dependent.
Conclusion
We report a simple, easy, quick one-step green synthesis of AuNPs which is reproducible and ecofriendly which does not require any other reducing agent. The biomolecules in the aqueous seed extract of M. indica act as reducing and stabilizing agent. AuNPs showed characteristic peak at 550 nm. The green synthesized AuNPs were predominantly round, triangle and irregular in shape and average size was 19.45 nm. Antimicrobial activity was done against a number of pathogenic microorganisms and green symthesized AuNPs exhibited antimicrobial activity more towards Gram-positive bacteria. They also showed dose-dependent DPPH, ABTS and SO antioxidant activities and exhibited potential cytotoxicity on HeLa cancer cell line, MCF-7 cell line and Normal fibroblast cell line. The results suggest that biowaste (seed) can be effectively used for green synthesis of AuNPs that can be used as a natural antimicrobial, antioxidant and anticancer agent. This is a good example of best from waste. M. indica mediated synthesized AuNPs could be employed as a source for the exploration of novel therapeutic agents in biomedical field to control various human diseases.
Acknowledgements
The authors thank Department of Biosciences (UGC-CAS) for providing excellent research facilities.
Disclosure statement
No potential conflict of interest was reported by the author(s).
Data availability statement
The authors confirm that the data supporting the findings of this study are available within the article.
References
- Chanda S, Amrutiya N, Rakholiya K. Evaluation of antioxidant properties of some Indian vegetable and fruit peels by decoction extraction method. Am J Food Technol. 2013;8(3):173–182.
- Yemis GP, Bach S, Delaquis P. Antibacterial activity of polyphenol-rich pomegranate peel extract against Cronobacter sakazakii. Int J Food Prop. 2019;22(1):985–993.
- Basri HH, Talib RA, Sukor R, et al. Effect of synthesis temperature on the size of ZnO nanoparticles derived from pineapple peel extract and antibacterial activity of ZnO – Starch nanocomposite films. Nanomaterials. 2020;10(6):1061.
- Donga S, Chanda S. Best from waste: therapeutic potential of plant waste (seeds, peels, flowers). Int J Curr Microbiol Appl Sci. 2020;9(08).
- Parvez GMM. Pharmacological activities of mango (Mangifera indica): a review. J Pharmacogn Phytochem. 2016;5(3):1–7.
- Pande J, Chanda S. Screening of anticancer properties of some medicinal plants – review. Int J Curr Microbiol Appl Sci. 2020;9(3):1348–1362.
- Mohanpuria P, Rana NK, Yadav SK. Biosynthesis of nanoparticles: technological concepts and future applications. J Nanopart Res. 2008;10(3):507–517.
- Rajan A, Rajan AR, Philip D. Elettaria cardamomum seed mediated rapid synthesis of gold nanoparticles and its biological activities. Open Nano. 2017;2:1–8.
- Mapala K, Pattabi M. Mimosa pudica flower extract mediated green synthesis of gold nanoparticles. Nano World J. 2017;3(2):44–50.
- Soshnikova V, Kim YJ, Singh P, et al. Cardamom fruits as a green resource for facile synthesis of gold and silver nanoparticles and their biological applications. Artif Cells Nanomed Biotechnol. 2018;46(1):108–117.
- Latha D, Prabu P, Arulvasu C, et al. Enhanced cytotoxic effect on human lung carcinoma cell line (A549) by gold nanoparticles synthesized from Justicia adhatoda leaf extract. Asian Pac J Trop Biomed. 2018;8(11):540–574.
- Mukherjee S, Sau S, Madhuri D, et al. Green synthesis and characterization of monodispersed gold nanoparticles: toxicity study, delivery of doxorubicin and its bio-distribution in mouse model. J Biomed Nanotechnol. 2016;12(1):165–181.
- Milanezi FG, Meireles LM, de Christo Scherer MM, et al. Antioxidant, antimicrobial and cytotoxic activities of gold nanoparticles capped with quercetin. Saudi Pharm J. 2019;27(7):968–974.
- Vijayan R, Joseph S, Mathew B. Anticancer, antimicrobial, antioxidant, and catalytic activities of green-synthesized silver and gold nanoparticles using Bauhinia purpurea leaf extract . Bioprocess Biosyst Eng. 2019;42(2):305–319.
- Chahardoli A, Karimi N, Sadeghi F, et al. Green approach for synthesis of gold nanoparticles from Nigella arvensis leaf extract and evaluation of their antibacterial, antioxidant, cytotoxicity and catalytic activities. Artif Cells Nanomed Biotechnol. 2018;46(3):579–588.
- Ahn EY, Hwang SJ, Choi MJ, et al. Upcycling of jellyfish (Nemopilema nomurai) sea wastes as highly valuable reducing agents for green synthesis of gold nanoparticles and their antitumor and anti-inflammatory activity. Artif Cells Nanomed Biotechnol. 2018a;46(sup2):1127–1136.
- Mitra M, Bandyopadhyay A, Datta G, et al. Protective role of green synthesized gold nanoparticles using Terminalia arjuna against acetaminophen induced hematological alterations in male wistar rats. J Nanomed Nanotechnol. 2019;10(2).
- Harborne AJ. Phytochemical methods a guide to modern techniques of plant analysis. Springer Science and Business Media; 1998.
- Perez C, Paul M, Bazerque P. An antibiotic assay by the agar well diffusion method. Acta Biol Med Exp. 1990;15:113–115.
- Mc Cune LM, Johns T. Antioxidant activity in medicinal plants associated with the symptoms of diabetes mellitus used by the indigenous peoples of the North American boreal forest. J Ethnopharmacol. 2002;82(2-3):197–205.
- Robak J, Gryglewski RJ. Flavonoids are scavenger of superoxide anions. Biochem Pharmacol. 1988;37(5):837–841.
- Re R, Pellegrini N, Proteggente A, et al. Antioxidant activity applying an improved ABTS radical cation decolourisation assay. Free Radic Biol Med. 1999;26(9-10):1231–1237.
- Labieniec M, Gabryelak T. Effects of tannins on Chinese hamster cell line B14. Mutat Res Genet Toxicol Environ Mutagen. 2003;539(1–2):127–135.
- Nagalingam M, Kalpana VN, Rajeswari D, et al. Biosynthesis, characterization, and evaluation of bioactivities of leaf extract-mediated biocompatible gold nanoparticles from Alternanthera bettzickiana. Biotechnol Rep . 2018;19:e00268.
- Patra JK, Baek KH. Novel green synthesis of gold nanoparticles using Citrullus lanatus rind and investigation of proteasome inhibitory activity, antibacterial, and antioxidant potential. Int J Nanomed. 2015;10:7253–7264.
- Könen-Adıgüzel S, Adıgüzel AO, Ay H, et al. Genotoxic, cytotoxic, antimicrobial and antioxidant properties of gold nanoparticles synthesized by Nocardia sp. GTS18 using response surface methodology. Mater Res Express. 2018;5(11):115402.
- Yulizar Y, Utari T, Ariyanta HA, et al. Green method for synthesis of gold nanoparticles using Polyscias scutellaria leaf extract under UV light and their catalytic activity to reduce methylene blue. J Nanomater. 2017;2017:1–6.
- Barai AC, Paul K, Dey A, et al. Green synthesis of Nerium oleander-conjugated gold nanoparticles and study of its in vitro anticancer activity on MCF-7 cell lines and catalytic activity. Nano Converg. 2018;5(1):1–9.
- Patra N, Kar D, Pal A, et al. Antibacterial, anticancer, anti-diabetic and catalytic activity of bio-conjugated metal nanoparticles. Adv Nat Sci Nanosci Nanotechnol. 2018;9(3):035001.
- Singh P, Pandit S, Garnaes J, et al. Green synthesis of gold and silver nanoparticles from Cannabis sativa (industrial hemp) and their capacity for biofilm inhibition. Int J Nanomed. 2018;13:3571–3591.
- Shabanzadeh P, Yusof R, Shameli K, et al. Computational modeling of biosynthesized gold nanoparticles in black Camellia sinensis leaf extract. J Nanomater. 2019;2019:1–11.
- Sunderam V, Thiyagarajan D, Lawrence AV, et al. In-vitro antimicrobial and anticancer properties of green synthesized gold nanoparticles using Anacardium occidentale leaves extract. Saudi J Biol Sci. 2019;26(3):455–459.
- Gahlawat G, Choudhury AR. A review on the biosynthesis of metal and metal salt nanoparticles by microbes. RSC Adv. 2019;9(23):12944–12967.
- Hariharan A, Begum TNB, Ilyas MHM, et al. Synthesis of plant mediated gold nanoparticles using Azima tetracantha Lam. leaves extract and evaluation of their antimicrobial activities. Phcogj. 2016;8(5):507–512.
- Mishra A, Tripathy SK, Yun SI. Bio-synthesis of gold and silver nanoparticles from Candida guilliermondii and their antimicrobial effect against pathogenic bacteria. J Nanosci Nanotechnol. 2011;11(1):243–248.
- Dudhane AA, Waghmode SR, Dama LB, et al. Synthesis and characterization of gold nanoparticles using plant extract of Terminalia arjuna with antibacterial activity. Int J Nanosci Nanotechnol. 2019;15(2):75–82.
- Ahn EY, Lee YJ, Park J, et al. Antioxidant potential of Artemisia capillaris, Portulaca oleracea, and Prunella vulgaris extracts for biofabrication of gold nanoparticles and cytotoxicity assessment. Nanoscale Res Lett. 2018b;13(1):348.
- Markus J, Wang D, Kim YJ, et al. Biosynthesis, characterization, and bioactivities evaluation of silver and gold nanoparticles mediated by the roots of Chinese herbal Angelica pubescens Maxim. Nanoscale Res Lett. 2017;12(1):46.
- Ghosh S, Patil S, Chopade NB, et al. Gnidia glauca leaf and stem extract mediated synthesis of gold nanocatalysts with free radical scavenging potential. J Nanomed Nanotechnol. 2016.
- Bakur A, Niu Y, Kuang H, et al. Synthesis of gold nanoparticles derived from mannosylerythritol lipid and evaluation of their bioactivities. AMB Express. 2019;9(1):62.
- Rajan A, Vilas V, Philip D. Studies on catalytic, antioxidant, antibacterial and anticancer activities of biogenic gold nanoparticles. J Mol Liquid. 2015;212:331–339.
- Chandrasekaran R, Gnanasekar S, Seetharaman P, et al. Formulation of Carica papaya latex-functionalized silver nanoparticles for its improved antibacterial and anticancer applications. J Mol Liquid. 2016;219:232–238.
- Allen RT, Hunter WJ, Agrawal DK. Morphological and biochemical characterization and analysis of apoptosis. J Pharmacol Toxicol Method. 1997;37(4):215–228.
- Tiloke C, Phulukdaree A, Anand K, et al. Moringa oleifera gold nanoparticles modulate oncogenes, tumor suppressor genes, and caspase-9 splice variants in A549 cells. J Cell Biochem. 2016;117(10):2302–2314.
- Wang L, Xu J, Yan Y, et al. Synthesis of gold nanoparticles from leaf Panax notoginseng and its anticancer activity in pancreatic cancer PANC-1 cell lines. Artif Cells Nanomed Biotechnol. 2019;47(1):1216–1223.