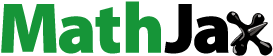
Abstract
Nanoparticles (NPs) have biological activities like antibacterial, antifungal, drug delivery, immunomodulation and antitumor activities. The aim of the current study was to investigate some of biomedical applications of silver NP synthesis using extracts from leaves of Eriobotrya japonica. Colour changes, UV–visible spectroscopy, SEM, zeta potential, dynamic light scattering, FTIR and XRD were used to confirm AgNPs formation. The UV–vis spectrum absorption band was observed at almost 430 nm. The SEM image shows quasi-spherical shape of AgNPs. The zeta potential demonstrated the negative surface charge of NPs. FTIR results showed the functional groups of AgNPs. Crystalline nature of AgNPs was confirmed by XRD pattern. MTT assay was used to study the anti-proliferative activity against MCF-7 and HeLa cells. Apoptosis was tested using a DNA-fragmentation test, and expression of P53. AgNPs inhibited the proliferation of MCF-7 and HeLa cells, and reduced inflammation. Treatment with AgNPs significantly decreased allergic disorder. AgNPs stimulated the phagocytosis process in BMDMs. The results suggested that AgNPs could be a promising therapy for future and preventing inflammation, reduce allergic disorders and prevent bacterial infection through the up-regulation of phagocytosis. Hence, future work such as developed and improved NPs as adjuvants, immune-modulating substances and nano-drug delivery system is needed.
Introduction
Medicinal plants have been used for centuries as remedies for human diseases [Citation1], and ancient medication was recently accepted as an alternate form of health care. As various infective agents are resistant to obtainable antibiotics, there is more incentive to use medicinal plants [Citation2]. Moreover, the increased use of plant extracts within the food, cosmetic and pharmaceutical industries suggests that research on medical plants is vital to discover active compounds [Citation3].
The loquat, Eriobotrya japonica, is a fruit tree that belongs to the Rosaceae family. This fruit tree is native to southeastern China and southern Japan, and it is also grown in other parts of the world, including the warmest climates. Previous studies showed that E. japonica extract contains many antioxidants and exhibits bioactivity capable of counteracting health issues, including inflammation, diabetes, cancer, bacterial and parasitic infections, pain and allergies. Bioactive compounds, such as phenolics and terpenoids, were isolated and characterized to improve our understanding of the chemical mechanisms underlying the biological activities of loquat extracts. However, poor solubility and bioavailability severely restrict their use [Citation4]. Therefore, scientists used a drug delivery system to improve the bioavailability of the drug and diminish toxicity related to the high doses that are typically required for an optimum response [Citation5].
Metallic nanoparticles (NPs) have received significant attention in the area of biomedical applications. Many chemical, physical and biological approaches exist for producing metallic NPs. Physical and chemical methods are time and energy consuming, expensive and not eco-friendly. For the biological approach, various enzymes, algae, microbes and plants are used. Silver NPs specifically have gained attention due to their unusual physiochemical. Recently, the green synthesis of NPs using plant extracts emerged as a promising methodology for the fabrication of metallic NPs because it involves a straightforward, fast, low-cost, environmentally friendly bioprocess, ease of scale-up, less biohazardous nature, and avoiding the hideous procedure of maintaining the cell lines [Citation6]. However, several factors influence the morphology and diameter of NPs and the characteristics of the active compounds extracted from plants. Thus, the green synthesis of NPs with specific morphology, size and stable distribution remains under development [Citation7].
Cancer remains a life-threatening disease that accounts for high mortality rates worldwide [Citation8]. Recently, announced data from Iraq’s national cancer registry indicate that over 31,500 cancer and tumour-related cases existed in Iraq in 2017‒2018. Cancer is considered one of the leading causes of mortality in the country, contributing to an estimated 11% of all deaths [Citation9]. The exact cause of cancer is unknown. Genetics is involved in 5–10% of cancers, and other contributing factors include poor diet, certain infections, lack of physical activity, obesity, the use of tobacco, and pollution, which can directly or indirectly influence the activity of crucial genes that can cause cancer development [Citation10].
When a person has cancer, inflammation, which is a non-specific immune response to infection, irritation or other injuries, begins with redness, fever, swelling, pain, and loss of function. The inflammation is triggered by innate immune receptors that recognize pathogens and damaged cells. The purpose of inflammation is to eliminate the initial cause of cell injury and remove necrotic cells and tissues damaged from the original injury and the inflammatory process to initiate tissue repair. Therefore, the inflammatory response is essential to remaining healthy and maintaining homeostasis. However, the inflammatory process does not always result in the successful resolution and repair of damaged tissue. Allergic disorders are a growing international pathological state for over 25% of populations in industrialized countries, and a significant worldwide increase has occurred in the prevalence of respiratory disease and rhinitis since 1960 [Citation11].
A hypersensitivity reaction, with objectively reproducible symptoms or signs, can be initiated by exposure to a defined stimulus at a dose that is tolerated by healthy subjects. The adaptive immune system can accidentally react against otherwise harmless antigens, which may have originated from the environment, such as from plant seeds, food or medication. Allergies refer to certain diseases in which the hypersensitivity reaction is triggered by environmental antigens initiating immunologic mechanisms, which cause tissue inflammation and organ dysfunction. Non-steroidal anti-inflammatory drugs (NSAIDs) have been used to treat various ailments for over a hundred years [Citation12]. As a class of medications, they possess anti-inflammatory, anti-allergy, analgesic and antipyretic activity and are widely used to treat chronic inflammatory states, such as inflammatory disease, skin disorders and respiratory illness. All NSAIDs are similar in terms of their anti-inflammatory efficaciousness. However, when combined, they cause unfavourable side effects, such as in the gastrointestinal tract, in increasingly more treated patients, and this often limits medical care. Green synthesis enables the creation of NPs from a reaction between a natural compound, which is used as a reducing agent, and metal salts. When plant extracts are used, they act as a reductant and a stabilizer agent for the system [Citation13]. Green synthesized of AgNPs using Acalypha indica Linn aqueous extract have been showed 40% cell inhibition of human breast cancer cells (MDA-MB-231) [Citation14]. Other study used AgNPs formed by Taxus baccata aqueous extract exhibits anti-proliferative activity against breast cancer cells MCF-7 cells (IC50 = 0.25 μg/mL) [Citation15]. Antitumor activity of both AgNPs and silver cations was expressed via oxidative stress as well as inflammation through production of ROS that cause DNA damage and mitochondrial dysfunction, releasing cytochrome c and resulting in mitochondrial related apoptosis and necrosis to cell proliferation and carcinogenesis [Citation16]. Ag NPs prepared by green synthesis using Lyngbya majuscula had anti-proliferative activity against leukemic cell lines (K562, MOLT3 and REH) [Citation17]. In this study, the silver NPs AgNPs were synthesis through a single-step silver ions reduction by E. japonica extract as a reducing agent and then investigate for first time their activity as an immune modulator agent as a new therapeutic approach against cancer cell lines. In particular, the study used in vitro and in vivo models to investigate the ability of synthesized AgNPs to reduce inflammation, induce phagocytosis, and act as anti-allergic agent.
Materials and methods
Cells and reagents
MCF-7 and HeLa cells were kindly provided by the Iraqi Centre for Cancer and Medical Genetic Research, Al-Mustansiriyah University, Baghdad, Iraq. RPMI-1640, foetal bovine serum, trypsin–EDTA, dimethyl sulphoxide (DMSO), 3-(4,5-dimethylthiazal-z-yl)-2,5diphenylterazolium (MTT), oval albumin, lipopolysaccharides (LPSs), adenosine triphosphate (ATP), Triton X-100, Giemsa stain, Eosin stain, xylene and paraffin were obtained from Sigma (St. Louis, MO). 3,3′,5,5′-Tetramethylbenzidine (TMB) microwell peroxidase substrate solution was obtained from KPL (Gaithersburg, MD), and stop solution was obtained from eBioscience (Hatfield, UK). However, all other chemicals and reagents were analytical grade level.
Extraction of plants
Eriobotrya japonica peels were collected from Al-Karada and Baghdad, Iraq during June and August 2018. The peels were dried and ground into powder. Then, 25 g of this powder was soaked in a flask containing 250 mL of deionized distilled water. Next, extraction was performed by incubating the flask on an orbital shaker at room temperature (RT) and agitating it at 100 rpm for 72 h. After extraction, the aqueous solution of the extract was filtered using Whatman filter paper no. 1. Then, the extract was concentrated under reduced pressure to obtain crude extract. Subsequently, the extract was maintained at 4 °C for further experiments [Citation18].
Synthesis of silver nanoparticles
The synthesis of AgNPs was performed using green synthesis following Sulaiman et al. [Citation19] with minor modification. Aliquots of 9 mL of 2 mM silver nitrate were added to 1 mL of E. japonica leaves extract and incubated for 8 h at RT. After incubation, the AgNO3 was bioreduced.
Characterization of silver nanoparticles
The synthesis of AgNPs was confirmed by UV–visible spectroscopic analysis using a Hitachi U-2910 spectrophotometer (Tokyo, Japan) via continuous scanning at 280–760 nm. Then, the morphology of the NPs was determined using a scanning electron microscopy (SEM; Shimadzu AA-7000, Tokyo, Japan) assay. The zeta potential of the NPs was studied using zeta sizer Nano-ZS90. We confirmed formation of AgNPs using DLS assay. This method relies on the light supply and the detector; 1 mL of distilled water was added into the cells, followed by the addition of 50 μL of the stock dispersions. The length of the NPs distribution was measured through the DLS method. Fourier transform infra-red (FTIR) was done to indicate the bonding of AgNPs through bridging linkage. The crystalline nature of the NPs was determined using an XRD-6000 X-ray diffractometer (Shimadzu, Tokyo, Japan) operated at a voltage and current of 40 kV and 30 mA, respectively, with Cu Kα radiation in 2θ configurations [Citation20–23].
Free radical scavenging activity
The assay of free-ranging scavenging activity was conducted using a manufactured kit (Gentaur, cat. no. ECAT-100, Kampenhout, Belgium). For each concentration of 10, 20, 30, 40, and 50 µg.mL−1 of synthesized AgNPs, 10 µL was transferred into different wells of a 96-well plate along with wells of 10 µL of the positive control and 10 µL of the sample blank. After that, 90 µL of the 50 µM substrate was added to wells to initiate catalase reactions. Then, the well plate was incubated for 30 min in a dark place at RT. After the incubation period, 100 µL of detection chemical reagent was added, and absorbance was measured at 570 nm. The amendment in colour was directly proportional to catalase activity within the sample.
3-(4,5-Dimethylthiazol-2-yl)-2,5-diphenyltetrazolium bromide assay
The cancer MCF-7 and HeLa cell lines were kept in RPMI-1640 in the presence of 10% foetal bovine serum, 100 units/mL of penicillin and 100 µg/mL of streptomycin. Cells were passaged in trypsin–EDTA and reseeded at 80% confluence twice a week while being incubated at 37 °C. A cytotoxicity assay of AgNPs against MCF-7 and HeLa cells was conducted following Alsaedi et al. [Citation24]. Cells were seeded at 5 × 104 cells mL−1 in wells of microtiter plates supplied with RPMI medium and allowed to adhere overnight. Dilutions of the AgNPs were prepared, added in triplicate and incubated for 24, 48 and 72 h. Thereafter, the 3-(4,5-dimethylthiazol-2-yl)-2,5-diphenyltetrazolium bromide (MTT) dye solution was added to the treated and untreated MCF-7 and HeLa cells. After each incubation period, the medium was aspirated, and a DMSO solution was applied to the samples. The absorbance for each well was determined at 492 nm on a microplate reader. Regression analysis was used to calculate the concentration of AgNPs required to inhibit 50% of cell growth (IC50) [Citation25].
Lactate dehydrogenase release assay
A lactate dehydrogenase (LDH) assay was done following the manufacturer’s instructions. Cells were incubated in media lacking Phenol red indicator. Then, cells were treated with AgNPs for 24, 48 and 72 h. Triton-X was used as a positive control. Samples were incubated at 37 °C for 2 h. After incubation time, transfer 75 μL aliquots from all test and control wells to a fresh 96-well flat. Then, 50 μL of the CytoTox 96® Reagent to each sample aliquot was added. The plate was covered with foil to protect from light and incubate for 30 min at RT. Added 50 μL of stop solution for I hour. Read the absorbance at 490 nm. Calculation the results using the equation
4′,6-Diamidino-2-phenylindole staining for the nucleus of cells
For the 4′,6-diamidino-2-phenylindole (DAPI) staining, MCF-7 and HeLa cells were subjected to 12-well plating, 24 h incubation with AgNPs at the IC50 and washing with phosphate-buffered saline (PBS) three times. Then, they were fixed with 1% glutaraldehyde for 30 min and washed with PBS before 30 min DAPI staining was performed, followed by another PBS washing. Finally, changes to nuclei were visualized using a fluorescence microscope [Citation26].
Immunofluorescence microscopy assay
For immunofluorescence, MCF-7 and HeLa cells were seeded onto plastic Lab-tek four-well slides. Then, the cells were treated with prepared AgNPs at the IC50 for 24 h. The cells were processed with steps of washing with PBS three times, fixation with 4% PFA for 30 min at RT, permeabilization with 0.5% Triton X for 30 min at RT, and blocking with 10% normal goat serum for 60 min. Next, the cells were treated with 1 µg mL−1 of each of the primary antibodies of anti-p53 for 24 h at 4 °C and washed with PBS three times. Afterward, cells were treated with 1 mg/mL of the secondary antibodies Alexa Fluor 488-conjugated goat anti-rabbit IgG for 2 h at RT. After additional washing of the cells three times using PBS, the cells were mounted in Vectashield with DAPI. Finally, they were examined under a fluorescent microscope [Citation27]. AgNPs were examined for their apoptotic-inducing activity using fluorescence microscopy analysis for apoptotic protein P53. Cells were left untreated as a control group or treated with AgNPs for 48 h at the IC50. P53 staining was shown as green, and nuclei were indicated by blue. The tumour suppressor p53 gene and caspases enzymes are helpful in the regular monitoring of cells and preventing them from transformation into tumour cells. When the cell shows any form of malignancy, an activation process of the mechanisms responsible for DNA repair is initiated for the restoration of the altered DNA. The immunofluorescent technique was performed to confirm and test whether the AgNPs could cause the induction of p53.
Anti-inflammatory activity of silver nanoparticles: an in vitro model
Bone marrow-derived macrophages
Male C57/BL6 mice of 7–8 weeks of age were used as the source for the isolation of primary bone marrow-derived macrophages (BMDMs) following Sulaiman et al. [Citation28]. After isolation, the BMDM cells were treated with LPSs and ATP in the presence and absence of AgNPs to study their ability to reduce inflammation.
Anti-inflammatory activity of silver nanoparticles: an in vivo model
Male mice aged 7–9 weeks were randomly divided into three groups of six mice. All animals were kept under standard environmental conditions of ∼23−27 C and at a humidity of ∼55–60% room humidity and a ∼12 h light/dark cycle. The mice were housed in polypropylene cages with wood dust and given access to food and water ad libitum. The experimental procedures were approved by the Animal Ethics Committee at the Biotechnology Division, Applied Science Department, University of Technology, Baghdad, Iraq. The first group was injected intraperitoneally (IP) with 250 µL of standard saline, the second group was injected IP with 50 µg LPS per animal, and the third group was pre-treated with AgNPs at a concentration of 1 mg kg−1 animal weight then injected IP with 50 µg LPS per animal [Citation29]. After 8 h, the mice were sacrificed, blood was collected by cardiac puncture, and peritoneal fluid was taken from the abdominal cavity after 10 h for analysis of IL-1β and IL-6 secretion levels.
Anti-allergic activity of silver nanoparticles: in vivo model
For the anti-allergy assay, male mice were randomly divided into four groups. Asthma was induced by ovalbumin (OVA) sensitization. Then, the mice were subjected to the OVA challenge. First, the mice were hypersensitized using an IP injection of 1 mg kg−1 of OVA suspended in 1 mL of PBS for three days, and the negative control group received standard saline. Three days after the final injection, synthesized AgNPs were orally administered 60 min before each OVA challenge. The second group was left without treatment as the positive control, in the third group, the mice received 1 mg kg−1 dexamethasone, and the fourth group mice received 500 mg kg−1 AgNPs. Then, the mice were stirred by exposing them to OVA for 15 min, except for the negative control group, which was not disturbed [Citation30]. The mice were stirred every day for four weeks by aerosolizing OVA solution. Then, the animals were sacrificed, and blood samples were collected from the tail vein of all mice to obtain blood film, eosinophils count, immunoglobulin E (IgE) levels and IFN-γ level. For the histopathological study, the lungs were separated and washed with PBS, fixed with 10% formalin, embedded with paraffin, sectioned and stained with haematoxylin and eosin [Citation31]. A light microscope was used to examine a lung tissue sections from the different groups.
Enzyme-linked immunosorbent assay
The cytokine concentration of mouse IL-1beta (Abcam, cat. no. ab1977742, Burlingame, CA), IL-6 (Abcam, cat. no. ab46100, Burlingame, CA), IL-8 (Biosource, cat. no. MBS7606860, Camarillo, CA), IgE (Abcam, cat. no. ab157718, Burlingame, CA) and IFN-γ (ab 100690) were measured using an enzyme-linked immunosorbent assay (ELISA). Briefly, a 96-well plate was used with a coating of 50 μL of substrate to capture antibodies at a dilution of 1:10 PBS in deionized water. Then, the plate was sealed and incubated overnight at 4 °C. The wells were washed three times with a washing buffer, blocked with 125 µL (1×) assay buffer, and incubated overnight at 4 °C. After washing, the samples and 50 μL of the standard were added to a prime standard of 1000 pg mL−1. After incubation for 120 min at RT and washing the plate four times, an antibody detection agent was added at 50 µL per well, then placed on a microplate, shaken at 400 rpm, and incubated for 60 min at RT. After that, streptavidin–HRP was added for 30 min at RT in the dark. Then, 50 µL TMB microwell peroxidase substrate solution was added and incubated at RT in the dark for 15 min. A measure of 25 μL of stop solution was added, and the absorbance was read using an ELISA plate reader at 570 nm.
Preparation of blood films
A drop of blood was smeared on a clean slide and left to dry. Then, the smear was stained with Giemsa stain for 5 min, rinsed in a buffer for 10 min, washed with tap water, and the slides were left to dry. Eosinophil cells were counted in each sample using ×100 magnification [Citation32].
Phagocytic activity assay
Staphylococcus aureus was cultured at 37 °C in broth media until reaching the mid-log phase (OD 0.4–0.6). Following centrifugation at 3500×g, for 15 min, at 4 °C, sterile PBS was used to wash the pellets three times. The concentration of the resuspended bacteria was determined by measuring the optical density at 540 nm. The BMDMs were pre-treated with AgNPs at a concentration of 10 μg mL−1 for 1 h. Then, the cells were infected with S. aureus at M.O.I. 1:25 and incubated at 37 °C for 45 min. Next, the BMDMs were lysed using lysis buffer. Tenfold serial dilutions of the lysates were incubated on Luria-Bertani agar plates at 37 °C for 24 h, and the total number and number of extracellular bacteria killed were counted to determine the number of intracellular bacteria killed [Citation33].
Phagocytosis of pHrodo Green S. aureus bioparticles by phagocytic cells
Phagocytic cells were first plated in four-well slides to determine the phagocytosis of pHrodo Green S. aureus BioParticles by phagocytic cells. The BMDM cells were pre-treated with AgNPs at a concentration of 10 µg/mL. pHrodo Green S. aureus BioParticles were mixed in 1× PBS at a pH of 7.2. After 24 h, the pHrodo particles were vortexed, and 50 µL of these particles was added to the BMDM cells. Phagocytosis was allowed for 3 h, and then the cells were fixed and co-stained with DAPI stain. Next, the cells were imaged using a Zeiss confocal microscope (Oberkochen, Germany). A blue colour represented the nuclei of the cells, and green dots represented the S. aureus pHrodo particles in the phagocytic cells.
Statistical analysis
The data were analysed using analysis of variance, and GraphPad Prism 5 (GraphPad Software, La Jolla, CA, USA) was used to statically process the data, which were shown as the mean ± SEM of the three replicates per experiment [Citation34].
Results and discussion
Characterization of silver nanoparticles
The E. japonica extract was yellow, and it changed to a dark brown after the addition of silver nitrate. The reduction of Ag+ into NPs during exposure to extract is visualized in , and the peak of synthesized AgNPs was observed at 430 nm (). The SEM image showed a relatively spherical shaped NP formed with a diameter in the range of 15–37 nm (). The zeta potential values indicated the surface charge of NPs which appeared to be negative charge for AgNPs. Additionally, it gives us an idea about the stability of NPs as in . DLS assay was done to determine of the Brownian motion of the spherical dispersed particles and to relate this to the particles' hydrodynamic length within the dispersed solution through dynamic variations of the scattered light intensity of this scattered light can be mathematically computed to relate the hydrodynamic length of the particle. As shown in , the diameter range of AgNPs was almost within 17–35 nm. The FTIR spectrum of leaves extract peaks appeared at 3402.54, 2891.39, 2314.66, 1741.78, 1687.77, 1616.40, 1518.03, 1383.01, 1068.60, 976.01, 906.57, 794.70, 758.05, 696.33, 630.74, 567.09, 513.08, and 449.43 cm−1, respectively. The band at 3402.54 cm−1 indicated phenolic OH. The bands at 2891.39 and 2314.66 cm−1 are for CH plane bends to alkenes. The bands at 1741.78, 1687.77, and 1616.40 cm−1 correspond to N–H bends to 1° amines. The band at 513.08 cm−1 corresponds to C–N to amines. The band at 1383.01 cm−1 corresponds to C–X to fluoride. The band at 1068.60 cm−1 corresponding to C–O. The bands at 976.01, 906.57, 794.70, 758.05, and 696.33 cm−1 corresponding to alkenes. The bands at 630.74, 567.09, 513.08, and 449.43 cm−1 corresponding to C–X to chloride as in . The XRD pattern evidently shows that the AgNPs formed by the reduction of Ag ions by E. Japonica extract are crystalline in nature ().
Figure 1. Formation of silver nanoparticles (AgNPs). (A) Colour changes. (1) Leaf extract. (2) Silver nitrate solution. (3) Silver nanoparticles at a concentration of 1 mM. (4) Silver nanoparticles at a concentration of 2.5 mM. (5) Silver nanoparticles at a concentration of 5 mM. (B) UV-spectroscopy of AgNPs.
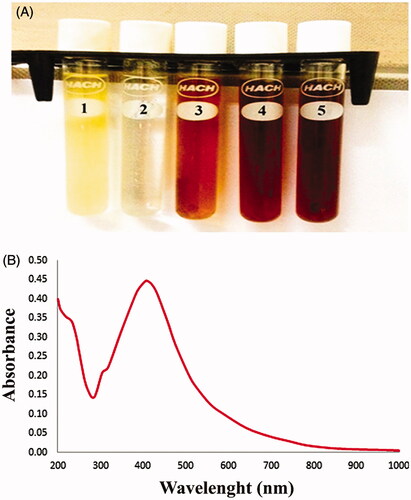
Silver nanoparticles reduce catalase activity
When the colorimetric method was used to determine H2O2 scavenging activity, AgNPs were found to be able to scavenge free radicals in a concentration-dependent manner, and a change in optical density occurred at 570 nm (). The value was represented as mean ± SEM. The optical density of positive control is 0.5860 ± 0.03550. Cells were treated with AgNPs at different concentration (10, 20, 30, 40 and 50 µg/mL). The optical density of different concentrations was 0.2516 ± 0.03068, 0.3150 ± 0.01137, 0.6284 ± 0.03415, 0.7654 ± 0.01615 and 0.8454 ± 0.01883, respectively. Enzymatic antioxidants play a crucial role in cellular defence against reactive oxygen species (ROS). Thus, the AgNPs showed high scavenging activities on hydroxyl radicals.
Figure 3. Antioxidant activity of silver nanoparticles (AgNPs). (A) AgNPs decrease catalase enzyme activity. (B) AgNPs augmented lactate dehydrogenase (LDH) release. Data are represented as mean ± SEM of three independent experiment. Asterisks indicate statistical different from untreated cells. *p≤.05; **p≤.01; ***p≤.001; ****p≤.001.
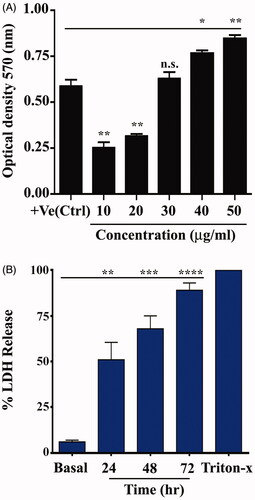
Effect of silver nanoparticles on the release of lactate dehydrogenase
The conversion of lactate to pyruvate in cells depends on the LDH enzyme, and this process is significant in energy production in cells. When this enzyme is released to culture media that contains treated cells with substances, it indicates the loss of cell membrane integrity [Citation35]. Cell viability was determined by an LDH, which determined the cytotoxicity effect of AgNPs in treated cells. Lactate dehydrogenase is released to the cytoplasm after cells damage, which causes the conversion of tetrazolium salt to formazan. Formazan production measured at 490 nm is relative to the number and amount of damaged, injured or dying cells [Citation36]. The cells were untreated (basal) as negative control, while the cells were treated with AgNPs at different time for 24, 48 and 72 h. Triton-X was used as a positive control. The percentage of LDH for basal cells was 6.000 ± 0.5774, while the percentage at different time was 51.00 ± 5.508, 68.00 ± 4.041 and 89.00 ± 2.309, respectively. The results showed the ability of AgNPs to penetrate treated cells, stimulate vesicle formation and enter vesicles (). Small AgNPs can easily enter cells and other biological systems and cause substantial cellular damage, leading to LDH release. However, NPs of 100–200 nm can also be taken up by cells and provoke toxic reactions, including DNA damage and genetic alternation. Nanoparticle toxicity could be related to the ability of NPs to disrupt the antioxidant system and induce oxidative stress [Citation14,Citation37]. Free radicals, such as ROS, mediate membrane damage, including mitochondrial and plasma membranes, which damage cellular components, such as lipids, fatty acids, proteins and nucleic acids, ultimately leading to cell death and loss of function of the electronic chain. The cytotoxicity effect of AgNPs on treated MCF-7 and HeLa cells in our study could be related to oxidative stress-mediated cellular damage. Similarly, the time dependent manner of the increase in LDH release caused by AgNPs could be related to the leakage of cellular enzymes and LDH via damaged plasma membranes [Citation38]. Recent study [Citation39] demonstrated that the AgNPs prepared by eco-friendly method using root extracts of Beta vulgaris L. had cytotoxic activity against human hepatic cancer cells cancer (HUH-7) using LDH assay.
Anti-proliferative activity of silver nanoparticles
We found highly significant cytotoxic activity of AgNPs against human cancer line MCF-7 and HeLa cells, suggesting that AgNPs can suppress cell growth in the MCF-7 cell line and HeLa cells (). The treatment of cancer cells with AgNPs for 72 h caused a significant decrease in the ability of cancer cells to proliferate, demonstrating the cytotoxic effects of AgNPs. AgNPs showed more cytotoxic effects on MCF-7 cells than HeLa cells. The AgNPs produced cytotoxicity in time dependent manner. The maximum cytotoxic level of AgNPs in MCF-7 cells was almost 75% after 72 h with IC50=18.05 and whereas it almost 65% for HeLa cells for same time with IC50=21.69. Overall, the results suggest a selective inhibitory effect of the AgNPs on MCF-7 and HeLa cells. Al-Sheddi et al. [Citation40] prepared silver NPs by a single-step reduction of silver ions using Nepeta deflersiana plant. Their study investigated anticancer potential of AgNPs against human cervical cancer (HeLa) cells. The results demonstrated that prepared AgNPs induces cytotoxicity in HeLa cells as a concentration-dependent manner. AgNPs were also found increases ROS which lead to induce oxidative stress. The increase in the intracellular ROS generation was found ultimately to trigger the development of mitochondrial membrane damage and cell cycle alterations. Also the results demonstrated that AgNPs have the ability of inducing apoptosis and necrosis cell death of HeLA cells through SubG1 cell cycle arrest. Additionally, after treating the MCF-7 and HeLa cells with AgNPs, the shape of the treated cancer cells showed evident cellular damage, morphological changes, plodding and inhibition of the binding of the treated cancer cells, and these changes were not observed in the untreated AgNPs cells.
Effect of silver nanoparticles on apoptosis and the regulation of the P53 protein
Apoptosis is a critical cellular homeostatic regulation mechanism for controlling the population size of various cell types [Citation41]. We hypothesized that the induction of apoptosis by AgNPs would be correlated with anti-proliferative activity against cancer cells. When the cancer cells were treated with AgNPs at the IC50 for 48 h and the characteristics of the apoptosis process, including nuclear morphological changes and DNA-fragmentation in cancer cell lines, were analysed using DAPI staining, the control cells showed healthy nuclei morphology (). demonstrates the induction of p53 in the cell lines following treatment with AgNPs at the IC50. The fluorescence of p53 was very low in the control cells, while in the treated MCF-7 and HeLa cells, p53 signalling was very evident, suggesting that the AgNPs are able to induce apoptosis via the p53 pathway. A previous study [Citation39] reported that the AgNPs prepared by green synthesis method induce apoptosis in human liver cancer cells initiated by the activation of caspase-3, the concentration of AgNPs was 20 and 40 µg/mL the caspase-3 activity was measured by ELISA and immunoblotting assays. Activation of caspase-3 was accompanied by the down-regulation of Bcl2 and the up-regulation of Bax and p53 proteins. All these events led to the appearance of the morphological signs of apoptosis, which were observed in the cancer cells after exposure to AgNPs.
Silver nanoparticles as anti-inflammatory agents
Inflammation is a process of defensive biological reactions to harmful agents, injury or infection that leads to pain, redness, swelling and heat in the body. The inflammatory response is a complex phenomenon, which can sometimes be helped by the use of known anti-inflammatory agents [Citation42]. In this study, AgNPs were used as an anti-inflammation compound, which altered the activation of inflammasome in vitro and in vivo. These results showed IL-1b secretion in supernatant culture in vitro when BMDM cells were treated with LPS 500 µg/mL + 5 mM of ATP compared with the untreated basal cells. However, BMDM cells pre-treated with AgNPs showed significantly lower IL-1b levels and IL-6 levels than cells treated with LPS and ATP (). Meanwhile, the results of the in vivo model on IL-1β levels and IL-6 confirmed the ability of AgNPs to reduce IL-1β and IL-6 levels (), respectively. The prepared AgNPs inhibited the secretion of proinflammatory cytokines, such as IL-6 and TNF-α, by inhibiting the production of LPS-induced cytokines and inhibiting signalling pathways for mitogen-activated protein kinases and nuclear factor kappa-light-chain-enhancer of activated B cell pathways, which induced LPS-activation of macrophages [Citation43]. Overall, we found that AgNPs were synthesized using E. japonica leaf extract, and they were effective against acute inflammation induced by LPS and ATP by significantly suppressing proinflammatory cytokines, such as IL-1β.
Figure 6. Silver nanoparticles inhibit the inflammation process. (A) Level of IL-1beta in vitro assay. Asterisks inside the column indicate statistical differences from the basal. Asterisks outside the column indicate statistical differences from lactate dehydrogenase (LPS)+adenosine triphosphate (ATP) treated cells. (B) Level of IL-1beta in vivo assay. Asterisks inside the column indicate statistical differences from basal. While, asterisks outside the column indicate statistical different from LPS treated cells. Results are represented as the mean ± SEM of three independent results. **p≤.01 and ***p≤.001.
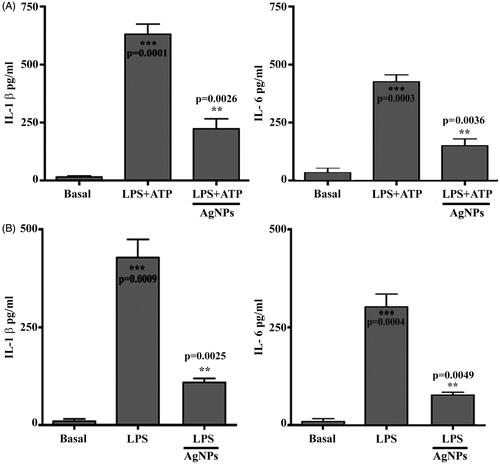
Anti-allergy activity of silver nanoparticles
Allergic reactions are the hypersensitized response of the immune system of allergic individuals to a substance. Once the antigen invades the body, it causes the body’s immune system to develop a hypersensitive reaction, evoking IgE [Citation44]. Asthma is a common chronic disease associated with a complex interaction of bronchial hyper-responsiveness, underlying inflammation and airflow interference [Citation45]. We found that OVA significantly increased eosinophil numbers and IgE, IFN-γ levels. The eosinophil count is significantly increased more than 22% in OVA challenged group. While, the count is decreased to less than 10% in group that pre-treated with dexamethasone for 60 min and in group that treated with AgNPs. The results demonstrated that the IgE, and IFN-γ levels significantly decreased when the results compared with the OVA challenged group. Meanwhile, oral administration of AgNPs significantly decreased eosinophil counts, IgE levels and IFN-γ levels as shown in ). The mechanism-dependent-T-helper cell (Th2), which can produce several proinflammatory cytokines after activation in asthma, such as IL-4, IL-5, IL-9, and IL-13, causes an increase in eosinophil, increased production of specific IgE, and the development of airway hyper-responsiveness. Therefore, decreasing allergic airway inflammation by treating mice with an antioxidant compound protects against increasing Th2 cytokines in mice [Citation46]. The mechanism-dependent Ca influx suggested that the anti-allergic drug inhibited calcium influxes by blocking calcium channels in rat tracheal smooth muscle. When the lung tissue sections of the control group were examined, we observed a healthy wall of a bronchiole with its lining epithelial tissue lying on a skinny layer of swish muscle fibres, and a respiratory organ tissue section of the OVA group showed increased eosinophils in the cellular infiltration. However, the lung tissue section of the DEX group revealed decreases in cellular infiltration, and the section of AgNPs showed decreases in cellular infiltration ().
Figure 7. Anti-allergic activity of silver nanoparticles (AgNPs). (A) AgNPs reduce percentage of eosinophil count. (B) AgNPs reduce IgE level. (C) AgNPs reduce IFN-γ level. Asterisks inside the column indicate statistical differences from the basal. While, asterisks outside the column indicate statistical differences from dexamethasone and AgNPs treated animals. Data are represented as mean ± SEM of three independent experiment. *p≤.05, **p≤.01 and ***p≤.001. (D) Photomicrographs of sections of the lung tissue were showed as: (1) a normal control group. (2) Asthmatic animal group. (3) Dexamethasone treated group. (4) AgNPs treated group. Magnification power ×40.
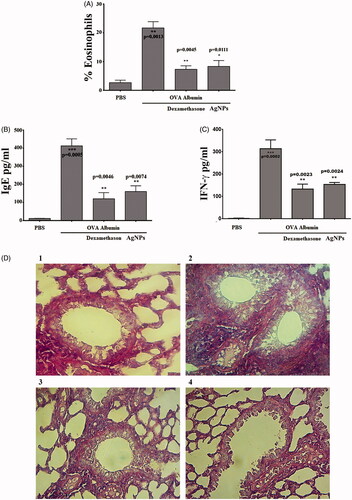
Silver nanoparticles induce phagocytic cell activity
Macrophages are cellular elements of the immune system that are characterized by plasticity and heterogeneity. In innate and adaptive immunity, phagocytic cells exhibit the ability to control the initiation and resolution phases because of their effective abilities to present antigens, secrete cytokines and engulf foreign bodies, such as bacterial cells. The function of these phagocytic cells is critically dependent on their polarization and reprogramming states. The possibility exists for M0 macrophages to polarize into an M1 macrophage, with proinflammatory functions, or M2 macrophages, with anti-inflammatory functions, in response to various micro-milieu conditions. However, the reversal of the phenotype can also lead to the reprogramming of already-polarized cells [Citation47]. We investigated the ability of AgNPs to induce phagocytosis at concentration of 25 μg mL−1. Our results showed the ability of AgNPs to enhance the activity of phagocytic cells. We measured the numbers of viable internalized S. aureus in phagocytic cells following infection, with and without treatment with AgNPs, to gauge the activity of phagocytosis and intracellular killing. Phagocytes pre-treated with AgNPs showed significant increases in their intracellular killing activity of the ingested, live S. aureus (). The percentage of intracellular killing was more than 40%, while this percentage is raised up to 60% in the presence of AgNPs. Thus, AgNP-treated BMDMs cells showed an enhanced antimicrobial response to Gram + ve bacterial strains. The role of FcgRIIa expression on the surface of phagocytic cells in the intracellular killing of S. aureus has already been studied [Citation48]. In addition, we investigated the phagocytic activity of BMDMs by measuring the uptake of a tagged S. aureus that only showed a fluorescent signal after moving to an acidic compartment of the lysosome. We compared the phagocytosis of pHrodo S. aureus BioParticles following the pre-treatment of phagocytic cells with AgNPs at a concentration of 10 µg mL−1. We found that the BMDMs that were not treated with AgNPs exhibited less phagocytosis than the BMDMs cells pre-treated with AgNPs (). We measured the ability of BMDMs to secrete IL-8 before and after being treated with AgNPs to study the possible mechanism by which AgNPs enhance the phagocytic activity of cells. We found that the BMDMs cells could produce cytokines, such as IL-8, after treatment with AgNPs (). Additionally, the percentage of intracellular killing activity increased after BMDMs were pre-treated with AgNPs. An ELISA assay was conducted using mouse cytokine IL-8 assay kits to estimate the level of IL-8 in BMDM supernatants after pre-treatment with AgNPs for 24 h in the presence and absence of Nystatin at a concentration of 50 µg mL−1 as an inhibitor for IL-8. After that, the optical densities of samples were read at 450 nm. Overall, AgNPs augmented BMDMs and phagocytosis activity due to IL-8 production. The increase in phagocytic activity is likely a consequence of the AgNPs containing chemical substances, which acted as immune stimulators and enhancers, leading to increases in the activity of BMDMs to engulf foreign bodies, such as bacterial strains [Citation49]. A study by Fraser et al. [Citation50] verified effect of silver NP on circulating mature, CD16bright/CD62Lbright and immature, CD16dim/CD62Lbright neutrophils. In their study, silver NPs triggered CD62L shedding and activation of mature circulating neutrophils. Nevertheless, CD62L shedding and activation was not observed in immature neutrophil population. Instead, silver NPs dramatically increased CD16 expression in the immature neutrophils. As CD16 is a marker of neutrophil maturation, this suggests silver NPs could trigger maturation of circulating CD16dim/CD62Lbright neutrophils.
Figure 8. Silver nanoparticles (AgNPs) enhanced phagocytosis. (A) AgNPs increase intracellular killing index in macrophage cells. (B) AgNPs augment bone marrow-derived macrophages in phagocytosis of pHrodo S. aureus BioParticles. (C) AgNPs increase IL-8 concentration in phagocytic cells with commercial Ag nanoparticles used as a positive control. Data are represented as mean ± SEM of three independent experiments. Asterisks indicate statistical different in the presence and absence of AgNPs. **p≤.01 and ***p≤.001.
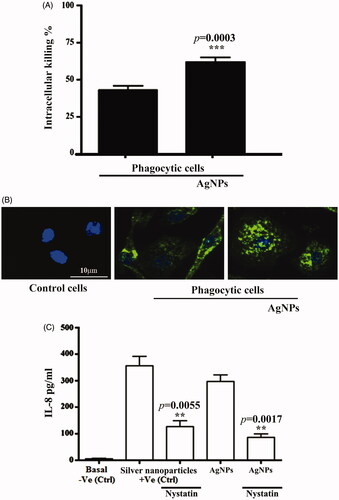
Conclusions
Eriobotrya japonica is a herbal medicine that contains numerous natural components, such as phenols, alkaloids and flavonoids, and has various pharmacological properties. In this study, AgNPs were synthesized using green synthesis. We hypothesized that the AgNPs will increase the potential activity of E. japonica leaves extract. To test our hypothesis, we tested the role AgNPs in some biomedical applications. We found that AgNPs inhibited the proliferation of cancer cell lines and induced apoptosis. Silver NPs decreased the IL-1beta and IL-6 levels in both the in vivo and in vitro models. Meanwhile, AgNP administration significantly decreased the eosinophil count, IgE level, IFN-γ level and the differential cell count under microscopic examination compared with the OVA challenged group. Furthermore, we found significantly more phagocytosis in the presence of AgNPs than in non-treated cells due to increasing IL-8 levels. The results confirmed that AgNPs could be used as a medicinal drug for treating different types of cancers and promoting immune system functions in the future. The biosynthesized of AgNPs using ecofriendly methods may be used for many biomedical applications. Nevertheless, further studies such as assessment of AgNPs toxicity in vivo and in vivo as well as study that details the possible mechanisms how these NPs killed cancer cells and how they modulate immune system are needed.
Acknowledgements
The authors extend their appreciation to the University of Technology and Researchers Support & Services Unit for their technical support.
Disclosure statement
No potential conflict of interest was reported by the author(s).
Additional information
Funding
References
- Eddouks M, Chattopadhyay D, De Feo V, et al. Medicinal plants in the prevention and treatment of chronic diseases 2013. Evid Based Complement Alternat Med. 2014;2014:180981.
- Gupta PD, Birdi TJ. Development of botanicals to combat antibiotic resistance. J Ayurveda Integr Med. 2017;8(4):266–275.
- Ekor M. The growing use of herbal medicines: issues relating to adverse reactions and challenges in monitoring safety. Front Pharmacol. 2014;4:177.
- Li H, Li Y, Ao H, et al. Folate-targeting annonaceous acetogenins nanosuspensions: significantly enhanced antitumor efficacy in HeLa tumor-bearing mice. Drug Deliv. 2018;25(1):880–887.
- Wen H, Jung H, Li X. Drug delivery approaches in addressing clinical pharmacology-related issues: opportunities and challenges. AAPS J. 2015;17(6):1327–1340.
- Khan M, Khan M, Adil SF, et al. Green synthesis of silver nanoparticles mediated by Pulicaria glutinosa extract. Int J Nanomed. 2013;8:1507–1516.
- Carmona ER, Benito N, Plaza T, et al. Green synthesis of silver nanoparticles by using leaf extracts from the endemic Buddleja globosa hope. Green Chem Lett Rev. 2017;10(4):250–256.
- Al Ansari RF, AL Gebori AM, Sulaiman GM. Serum levels of zinc, copper, selenium and glutathione peroxidase in the different groups of colorectal cancer patients. Caspian J Intern Med. 2020; 11(4):384-390.
- GBD 2015 Risk Factors Collaborators. Global, regional, and national comparative risk assessment of 79 behavioural, environmental and occupational, and metabolic risks or clusters of risks, 1990–2015: a systematic analysis for the Global Burden of Disease Study 2015. Lancet. 2016;388:1659–1724.
- Anand P, Kunnumakara AB, Sundaram C, et al. Cancer is a preventable disease that requires major lifestyle changes. Pharm Res. 2008;25(9):2097–2116.
- Thomsen SF. Epidemiology and natural history of atopic diseases. Eur Clin Respir J. 2015;2(1):24642.
- Warrington R, Watson W, Kim HL, et al. An introduction to immunology and immunopathology. Allergy Asthma Clin Immunol. 2011;7:S1.
- Singh J, Dutta T, Kim KH, et al. 'Green' synthesis of metals and their oxide nanoparticles: applications for environmental remediation. J Nanobiotechnol. 2018;16(1):84.
- McShan D, Ray PC, Yu H. Molecular toxicity mechanism of nanosilver. J Food Drug Anal. 2014;22(1):116–127.
- Kajani AA, Bordbar A-K, Esfahani SHZ, et al. Green synthesis of anisotropic silver nanoparticles with potent anticancer activity using Taxus baccata extract. RSC Adv. 2014;4(106):61394–61403.
- Datkhile KD, Durgawale PP, Patil MN. Biogenic silver nanoparticles are equally cytotoxic as chemically synthesized silver nanoparticles. Biomed Pharmacol J. 2017;10:337–344.
- Roychoudhury P, Gopal PK, Paul S, et al. Cyanobacteria assisted biosynthesis of silver nanoparticles—a potential antileukemic agent. J Appl Phycol. 2016;28(6):3387–3394.
- Melo IR, Pimentel MF, Lopes CE, et al. Application of fractional factorial design to levan production by Zymomonas mobilis. Braz J Microbiol. 2007;38(1):45–51.
- Sulaiman GM, Mohammed WH, Marzoog TR, et al. Green synthesis, antimicrobial and cytotoxic effects of silver nanoparticles using Eucalyptus chapmaniana leaves extract. Asian Pac J Trop Biomed. 2013;3(1):58–63.
- Sulaiman GM, Ali EH, Jabbar II, et al. Synthesis, characterization, antibacterial and cytotoxic effects of silver nanoparticles. Dig J Nanomater Biostruct. 2014;9:787–796.
- Jabir MS, Nayef UM, Kadhim WKA. Polyethylene glycol-functionalized magnetic (Fe3O4) nanoparticles: a novel DNA-mediated antibacterial agent. Nano BioMed Eng. 2019;11(1):18–27.
- Khashan KS, Jabir MS, Abdulameer FA. Carbon nanoparticles prepared by laser ablation in liquid environment. Surf Rev Lett. 2019;26(10):1950078.
- Kadhim WKA, Nayef UM, Jabir MS. Polyethylene glycol-functionalized magnetic (Fe3O4) nanoparticles: a good method for a successful antibacterial therapeutic agent via damage DNA molecule. Surf Rev Lett. 2019;26(10):1950079.
- Alsaedi IIJ, Taqi ZJ, Abdul Hussien AMA, et al. Graphene nanoparticles induces apoptosis in MCF-7 cells through mitochondrial damage and NF-kB pathway. Mater Res Express. 2019;6(9):95413.
- Albukhaty S, Naderi-Manesh H, Tiraihi T, et al. Poly-l-lysine-coated superparamagnetic nanoparticles: a novel method for the transfection of pro-BDNF into neural stem cells. Artif Cells Nanomed Biotechnol. 2018;46(Suppl. 3):S125–S132.
- Kadhem HA, Ibraheem SA, Jabir MS, et al. Zinc oxide nanoparticles induces apoptosis in human breast cancer cells via caspase-8 and P53 pathway. Nano BioMed Eng. 2019;11(1):35–43.
- Jabir MS, Nayef UM, Abdulkadhim WK, et al. Supermagnetic Fe3O4-PEG nanoparticles combined with NIR laser and alternating magnetic field as potent anti-cancer agent against human ovarian cancer cells. Mater Res Express. 2019;6(11):115412.
- Sulaiman GM, Waheeb HM, Jabir MS, et al. Hesperidin loaded on gold nanoparticles as a drug delivery system for a successful biocompatible, anti-cancer, anti-inflammatory and phagocytosis inducer model. Sci Rep. 2020;10(1):9362.
- Chen T, Yan J, Li Y. Genotoxicity of titanium dioxide nanoparticles. J Food Drug Anal. 2014;22(1):95–104.
- Chen S, You H, Mao L, et al. Dibutyl phthalate induced oxidative stress does not lead to a significant adjuvant effect on a mouse asthma model. Toxicol Res. 2015;4(2):260–269.
- Ali IH, Jabir MS, Al-Shmgani HS, et al. Pathological and immunological study on infection with Escherichia coli in male BALB/c mice. J Phys Conf Ser. 2018;1003:12009.
- Sulaiman GM, Jabir MS, Hameed AH. Nanoscale modification of chrysin for improved of therapeutic efficiency and cytotoxicity. Artif Cells Nanomed Biotechnol. 2018;46(Suppl. 1):708–720.
- Taqi ZJ, Abdul-Wahed HE, Al-Saadi HK, et al. Potential activity of silver nanoparticles synthesized by Iraqi propolis on phagocytosis. AIP Conf Proc. 2020;2213:20104.
- Al-Salman HNK, Ali ET, Jabir M, et al. 2-Benzhydrylsulfinyl-N-hydroxyacetamide-Na extracted from fig as a novel cytotoxic and apoptosis inducer in SKOV-3 and AMJ-13 cell lines via P53 and caspase-8 pathway. Eur Food Res Technol. 2020;246(8):1591–1608.
- Gaspar P, Al-Bayati FAY, Andrew PW, et al. Lactate dehydrogenase is the key enzyme for pneumococcal pyruvate metabolism and pneumococcal survival in blood. Infect Immun. 2014;82(12):5099–5109.
- Vijayakumar S, Ganesan S. In vitro cytotoxicity assay on gold nanoparticles with different stabilizing agents. J Nanomater. 2012;2012:1–9.
- Khashan KS, Sulaiman GM, Hussain SA, et al. Synthesis, characterization and evaluation of anti-bacterial, anti-parasitic and anti-cancer activities of aluminum-doped zinc oxide nanoparticles. J Inorg Organomet Polym. 2020;30(9):3677–3693.
- Bhattacharyya A, Chattopadhyay R, Mitra S, et al. Oxidative stress: an essential factor in the pathogenesis of gastrointestinal mucosal diseases. Physiol Rev. 2014;94(2):329–354.
- Bin-Jumah M, Monera AA, Albasher G, et al. Effects of green silver nanoparticles on apoptosis and oxidative stress in normal and cancerous human hepatic cells in vitro. Int J Nanomedicine. 2020;15:1537–1548.
- Al-Sheddi ES, Farshori NN, Al-Oqail MM, et al. Anticancer potential of green synthesized silver nanoparticles using extract of Nepeta deflersiana against human cervical cancer cells (HeLA). Bioinorg Chem Appl. 2018;2018:9390784.
- Gupta S, Kass GE, Szegezdi E, et al. The mitochondrial death pathway: a promising therapeutic target in diseases. J Cell Mol Med. 2009;13(6):1004–1033.
- Lodish H, Berk A, Zipursky SL, et al. DNA damage and repair and their role in carcinogenesis. In Molecular cell biology, 4th edn. W.H. Freeman, New York; 2000.
- Vasto S, Candore G, Balistreri CR, et al. Inflammatory networks in ageing, age-related diseases and longevity. Mech Ageing Dev. 2007;128(1):83–91.
- Zar PPK, Morishita A, Hashimoto F, et al. Anti-inflammatory effects and molecular mechanisms of loquat (Eriobotrya japonica) tea. J Funct Foods. 2014;6:523–533.
- Becker Y. The changes in the T helper 1 (Th1) and T helper 2 (Th2) cytokine balance during HIV-1 infection are indicative of an allergic response to viral proteins that may be reversed by Th2 cytokine inhibitors and immune response modifiers–a review and hypothesis. Virus Genes. 2004;28(1):5–18.
- Noutsios GT, Floros J. Childhood asthma: causes, risks, and protective factors: a role of innate immunity. Swiss Med Wkly. 2014;144:w14036.
- Steinke JW, Borish L. Th2 cytokines and asthma. Interleukin-4: its role in the pathogenesis of asthma, and targeting it for asthma treatment with interleukin-4 receptor antagonists. Respir Res. 2001;2(2):66–70.
- Oczypok EA, Oury TD, Chu CT. It's a cell-eat-cell world: autophagy and phagocytosis. Am J Pathol. 2013;182(3):612–622.
- Waheeb HM, Sulaiman GM, Jabir MS. Effect of hesperidin conjugated with golden nanoparticles on phagocytic activity: in vitro study. AIP Conf Proc. 2020;2213:20217.
- Fraser JA, Kemp S, Young L, et al. Silver nanoparticles promote the emergence of heterogeneic human neutrophil sub-populations. Sci Rep. 2018;8(1):1–14.