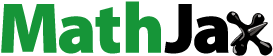
Abstract
PEG-interpenetrated dual-sensitive hydrogels that load nano lipid carrier (NLC) were researched and developed for topical drug administration. Natural antioxidant α-lipoic acid (ALA) was selected as our model drug. The α-lipoic acid (ALA) nano lipid carrier was successfully prepared by hot melt emulsification and ultrasonic dispersion method, and the physicochemical properties of the nano lipid carrier were investigated, including morphology, particle distribution, polydispersity coefficient, zeta potential and encapsulation efficiency. Carboxymethyl chitosan and poloxamer 407 contributed to pH- and temperature-sensitive properties in the hydrogel, respectively. Natural non-toxic cross-linking agent genipin reacted with carboxymethyl chitosan to form the hydrogel. Poly ethylene glycol (PEG), a polymer compound with good water solubility and biocompatibility, interpenetrated the hydrogel and influenced the mechanical strength and drug release behaviour. FI-IR test verified the successful synthesis of the hydrogel. The rheological parameters indicated that the mechanical strength of the hydrogel was positively correlated with the amount of PEG, and the in vitro dissolution profiles demonstrated that the increasement of PEG could accelerate the drug release rate. The compatibility of the drug delivery system was verified with cells and mice model. Topical delivery of ALA in solution, NLC and NLC-gel was investigated in-vitro.
Introduction
Alpha lipoic acid (ALA) is a naturally occurring organic sulphur compound that can be extracted from plants and animals [Citation1]. As a natural biological antioxidant, α-lipoic acid has received a lot of attention in the past few years [Citation2]. It has been verified to have active oxygen scavenging effect and anti-inflammatory properties [Citation3–5]. As an antioxidant, it can be effective in many fields, such as vitamin supplements for therapeutic drugs, health foods and anti-aging beauty products [Citation6–9]. Alpha-lipoic acid has good physicochemical properties for transdermal administration, but its hydrophobicity and extreme vulnerability to degradation by the sunlight limit its application [Citation10,Citation11].
Nano lipid carrier is a promising nanomedical drug delivery system that composes both solid and liquid lipids. It can increase the permeability of topical medications, promote closing effect and reduce adverse reactions [Citation12]. Avoiding the usage of organic solvents in the production process could ensuring good biocompatibility and bioavailability, controlled release effects, and potential for mass production [Citation13–16]. The nano lipid carrier can encapsulate hydrophilic/hydrophobic drugs with a good encapsulation efficiency. Due to the existence of the solid matrix, the nano lipid carrier can also immobilise the drug and prevent the aggregation of nanoparticles for a long time [Citation17]. However, because of the low-viscosity nature of nanoparticles, it will be quickly cleared by the skin's defense mechanisms when applied topically. In order to overcome this drawback, semi-solid system (e.g. hydrogel) with appropriate viscosity and mechanical strength was introduced as NLC carrier to prolong local residence time and extend the acting time of the drug [Citation18–21].
Hydrogel is an important biological material which was widely used in tissue engineering and pharmaceutical applications [Citation22–25]. Generally, hydrogel is composed of natural products and synthetic products, and its properties can be further modulated by physical interpenetration or chemical cross-linking [Citation26–29]. Natural polysaccharides such as chitosan and its derivatives have good biocompatibility and biodegradability, and are widely used in drug delivery and tissue engineering [Citation25]. Carboxymethyl chitosan (CMCS) is a kind of shell derivatives with the characteristics of chitosan. It has better solubility, moisturising properties and anionic properties than chitosan [Citation30]. Commercial temperature sensitive material Poloxamer 407 (P407) is capable to self-assemble to micella in water when the concentration exceeds the critical micella concentration (CMC) as well as the temperature surpass the lower critical solution temperature (LCST) [Citation31–33]. P407 and carboxymethyl chitosan were physically mixed to endow the pH- and temperature-sensitive properties in the hydrogel. Natural product genipin with good biocompatibility and low cytotoxicity was selected as cross-linker agent in our study [Citation34–36]. Synthetic polymers have an important effect on the reinforcement of natural polymers and have been proven to improve the mechanical properties and regulate the release behaviour of the system [Citation37]. Poly ethylene glycol (PEG) has been studied and extensively applied in the field of tissue engineering and medical delivery for the past few years, which possesses excellent physicochemical properties including superior hydrophilicity, lack of immunogenicity, high biocompatibility and resistance to protein adsorption [Citation38–41].
In this study, a controlled-release hydrogel with dual sensitivity of pH and temperature based on carboxymethyl chitosan and P407 was synthesised, and PEG4000 was interpenetrated into the hydrogel to adjust its mechanical strength and release behaviour. For the first time, this drug delivery platform was employed to topical dermal application, and the influence of PEG on mechanical properties and drug release profile was investigate. FT-IR was used to identify the reaction between carboxymethyl chitosan and genipin. Gel physicochemical properties including apparent morphology, rheological parameters, swelling coefficients were subsequently tested. The nano lipid carrier loaded with α-lipoic acid was successfully prepared and identified by TEM (Transmission Electron Microscopy) and nanosizer. ALA-NLC was loaded into the hydrogel by swelling-loading method. The in-vitro dissolution tested were applied by dialysis membrane method. The compatibility of NLC-hydrogels was tested on human umbilical cord mesenchymal stem cells (hUCMSCs) and mice skin model. The results of in-vitro skin permeation study revealed relevance to in-vitro dissolution tests.
Materials and methods
Materials
The model drug alpha lipoic acid (ALA) was purchased from Shanghai Xianding Biotechnology Co., Ltd.(Shanghai, China);Poloxamer 407 was purchased from Beijing Solarbio Science & Technology Co., Ltd.(Beijing, China);Carboxymethyl chitosan (CMCS, Viscosity =100–200 mpa.s, deacetylation degree ≥95%) was purchased from Shanghai Macklin Biochemical Co., Ltd. (Shanghai, China); Polyethylene glycol 4000 (PEG 4000) was purchased from Shanghai Yuanye Bio-Technology Co., Ltd. (Shanghai, China); Genipin (GP) was purchased from Beijing Ouhe Technology Co. Ltd.(Beijing, China); Compritol 888 ATO, a mixture of mono-, di- and triglycerides of behenic acid, was kindly provided by Gattefosse (Paris, France); Soy lecithin was purchased from Taiwei Pharmaceutical Co., Ltd. (Shanghai, China). Medium chain triglyceride (MCT) was purchased from Shanghai Yuanye Bio-Technology Co., Ltd. (Shanghai, China); Cremophor EL was generously presented by BASF (Shanghai, China); The deionised water was purified by a MiliQ® System (Millipore, Schwalbach, Germany). The other reagents used in this study were analytical grade.
Preparation of alpha lipoic acid loaded nano lipid carried (ALA-NLC)
The NLC dispersion was prepared by melt-emulsification followed by ultrasound homogenisation method [Citation42]. The lipid phase, composed of MCT (75 mg), Compritol 888 ATO (125 mg) and the active compound α-lipoic acid (7 mg), was melted at 88 °C under magnetic stirring until a transparent oil phase appeared. Cremophor EL (220 mg) and soy lecithin (91 mg) were dispersed in 10 ml deionised water to form the aqueous phase under magnetic stirring at 40 °C. Then, the aqueous phase was heated to 88 °C and subsequently added to the melted oil phase drop by drop under magnetic stirring (400 rpm) at 88 °C. The resulting dispersed coarse emulsion was homogenised for 3 min (300 W) using a probe-type cell disruptor (SCIENTZ-IID, Scientz, Ningbo, China). Afterward, the prepared homogenous nanoemulstion was immediately cooled in ice bath, inducing lipid phase recrystallization to form ALA-loaded nanoparticles. The final product was kept in a 4 °C refrigerator.
Physicochemical characterisation of NLC
Particle size distribution and charge measurement
The mean particles size (Z-ave), polydispersity index (PDI) and zeta potential of the ALA-NLC were assessed via a Zetasizer Pro (Malvern Instruments Ltd., UK) at 25 °C. To produce the appropriate scattering intensity, samples were diluted thirty times by deionised water before the test.
Transmission electron microscopy
The morphology test was performed with a Hitachi H-7650 electronic transmission electron microscope (Tokyo, Japan). 5 μl of ALA-NLC diluted sample was placed on an amorphous carbon film coated copper grid, then stained with 2% phosphotungstic acid and incubated 30 min at room temperature. The images were taken under 80 kV working voltage.
Determination of encapsulation efficiency
Mini-column centrifugation method was applied to determinate the encapsulation efficiency of α-lipoic acid in the ALA-NLC sample. A disposable syringe barrel (2.5 ml) was applied as the outer shell of simple chromatography column with its tip covered tightly with filter paper, the proper amount of Sephadex G-50 (Solarbio, China) was filled in as chromatography stationary phase. The column was dehydrated by high-speed centrifugation at 2000 rpm for 30 s before adding sample. Afterward, 0.2 ml of ALA-NLC sample was filled into the mini-column, which was centrifuged at the same rotational speed for 2 min. For the complete collection of encapsulated drug, the eluate extraction process was performed triple times with 0.2 ml deionised water as eluent each time. All the eluates were gathered and the nano lipid carrier inside was destroyed by methanol to completely release the encapsulated drug. The encapsulated drug concentrations (Ce) analysis was performed on Agilent 1260 IntinityIIhigh performance liquid chromatography (HPLC) system (Agilent, USA) using a Diamonsil C18 column (250 mm × 4.6 mm, 5 μm, Dikma, China). The column temperature was set at 40 °C. The mobile phase was composed of methanol and 0.1% acetic acid (54:46, V/V), and flow rate was set at 1.0 ml/min. The detection was carried out at 332 nm wavelength. In addition, the same amount of prepared formulation was destroyed by methanol and tested by the above mentioned HPLC method to determinate total drug concentration (Ct). EE was calculated in the following equations:
In this equation, EE represents the encapsulation efficiency; Ce represents the encapsulated drug concentration while Ct represents total drug concentration in the formulation.
Thermo gravimetric analysis (TGA)
TGA analysis was performed by a TGA-50 thermal gravimetric analyser (Shimadzu, Japan). Appropriate amount of α-lipoic acid and soybean lecithin were filled in open aluminium pans, and the heating procedure was set from 40 °C to 240 °C with a heating rate of 10 °C/min. The purge gas was nitrogen with a flow rate of 40 ml/min.
Synthesis of PEG-interpenetrated dual-sensitive hydrogel
Genipin (GP) was used as the crosslink agent to trigger the synthesise reaction. The powdered P407 and CMCS were added and mixed thoroughly in 10 ml deionised water under magnetic stirring at 37 °C. Subsequently, the crosslink reaction was performed by adding crosslink agent solution contained 2 mg GP drop by drop into the mixed solution, and the crosslinking action lasted for 2 h to form a viscous solution. Then PEG was melted by heating at 60 °C and added according to gradient weight ratios into the solution respectively under magnetic stirring at 37 °C. The components of the synthesised PEG-interpenetrated dual-sensitive hydrogels are summarised in .
Table 1. Compositions of the formation of PEG-penetrated F127/CMCS hydrogel.
ALA-NLC was loaded into the PEG-interpenetrated dual-sensitive hydrogel by swelling-loading method [Citation31]. Briefly, the lyophilised hydrogel stents were immerged in ALA-NLC dispersion at room temperature for 24 h to reach swelling equilibrium. Then the expanded hydrogel was carefully taken out from ALA-NLC dispersion, excess water on the surface of the expanded hydrogel was carefully wiped out with paper towel.
Fourier transform infra-red spectroscopy (FTIR) characterisation of functional group
To validate the functional group variation and the synthesise reaction effect, CMCS/P407 hydrogel was assayed by FTIR method. The analysis was performed using KBr as blank background on an FTIR-850 spectrometer (Gangdong, China) at 25 °C with wavelength from 4000 to 500 cm−1.
Characterisation of PEG-interpenetrated CMCS/P407 hydrogel properties
Scanning electron microscopy (SEM)
Hydrogels were pre-treated by dialysis method to totally remove PEG and then lyophilised. The pore size and surface morphology of hydrogel lyophilised stent were detected by a Hitachi SU3500 electronic scanning electron microscope (Hitachi, Japan), at 15.0 kV.
Rheological characterisation
The prepared five PEG-penetrated CMCS/P407 gels were characterised by a MCR92 rotational rheometer (Anto Paar, Austria). The shear rate ranged from 0.1 s−1 to 600 s−1, temperature was consistent at 35 °C. The remaining parameters are default parameters.
Swelling properties
The lyophilised PEG-penetrated CMCS/P407 gel stents were weighed in dry state before immersed in PBS (phosphate buffer saline, pH 2.5 or 6.5) at two kinds of temperature (25 or 35 °C). To measure the weight variation, the expanded hydrogel was carefully taken out from PBS with surface dried with paper towel and weighted at preconcerted time intervals. Swelling ratio (SR) of the samples was calculated according to the following formula:
In this equation, Ws represents the weight of the swollen hydrogel while W0 represents the weight of the lyophilised hydrogel. All the measurements were performed in triplicate, data are exhibited as the mean ± standard deviation.
In vitro dialysis dissolution test
Dynamic dialysis method was used to perform in-vitro release experiments [Citation30]. Dialysis membrane of molecular weight cut-off between 8000–12,000 relative molecular mass was selected to contain ALA saline solution, ALA-NLC and various formulations of ALA-NLC loaded hydrogels and fixed onto stirring paddles in sequence. 1 ml ALA solution, ALA-NLC and different kinds of swelling hydrogels contained 1 ml ALA-NLC were added into the dialysis bags, respectively. The dissolution medium was 100 ml PBS of pH 6.5 that maintained sink conditions. The dissolution medium temperature was set at 35 ± 0.5 °C, while paddle speed was at 100 rpm. Periodically, 1 ml aliquots of samples were withdrawn and subsequently same amount of preheated dissolution medium was replenished. ALA concentration was analysed by HPLC method at 332 nm as mentioned above in subsection “Determination of encapsulation efficiency.”
Hydrogel toxicity analysis on hUCMSCs
CCK-8 assay method was employed to assess the cell viability .The hUCMSCs were kindly supplied from Liaoning Medical Diagnosis and Treatment Centre (Shenyang, China). The hydrogels containing gradient PEG4000 were pre-treated respectively by culture media (volume ratio = 1:1) in a 37 °C incubator for 24 h, then the supernatants were collected and sterilised by filtration to get the hydrogel extractions as culture medium. Then the hUCMSCs were seeded into 96-well sterile plates with a seeding density of 1 × 104 cells per well. After 24 h of incubation in the 37 °C, 5% CO2 incubator, the above-mentioned supernatants were added to each well with the amount of 100 µl, respectively. hUCMSCs were incubated with the supernatants for another 24 h. Subsequently,10 µl CCK8 solution were added into each well to form a concentration of 10% and then incubated for 2 h under the same culture conditions. The absorbance values were measured at the wavelength of 450 nm via a microplate reader (SpectraMax M3, Molecular Devices, USA).
Results and discussion
Physicochemical characterisation of ALA-NLC systems
α-Lipoic acid is a promising drug candidate in the field of topical administration due to its strong antioxidant property and favourable physicochemical properties [Citation43]. In this research the α-lipoic acid (ALA) NLC was successfully prepared along with suitable carriers composed of both solid and liquid lipid materials. The dispersion produced by ultrasound homogenisation method revealed milk-like appearance, bluish after dilution.
The characteristics of nanoparticle distribution was mainly influenced by the aspects of particle size and polydispersity index, meanwhile, entrapment efficiency (EE%) was an important evaluation index of encapsulation efficiency. The measured parameters of the prepared ALA-NLC are listed in . ALA-NLC revealed a relatively low particle size, the small particle size of NLC along with the lipid inherent nature could promote the permeability of α-lipoic acid through skin epidermis [Citation12]. PDI value was inferior to 0.3, representing a relatively narrow size distribution. In terms of entrapment efficiency, the liquid lipid could interfere with ordered crystalline state and induce structure flaws in the core solid matrix, leading to more storage space and higher drug entrapment capacity. The particle size date was consistent with the subsequent TEM images as shown in , it was observed by transmission electron microscope that the particle morphology was spherical in shape. TGA thermogram of α-lipoic acid and soybean lecithin was revealed in , demonstrating both active ingredient and soybean lecithin remained stable at 88 °C. The experimental results verify the feasibility and validity of the melt-emulsification method.
Table 2. Physicochemical parameters of formulated ALA-NLC.
Characterisation of the CMCS/P407 hydrogel
The infra-red absorption spectrum detection results are revealed in . According to the spectrum, we could observe characteristic peaks of GP at 1680 and 1622 cm−1, triggered by the stretching vibration of C = O in carboxymethyl group and C = C in cycloolefin, respectively [Citation44,Citation45]. The stretching vibration of O–H contributed to the peaks between 3400 and 3200 cm−1 [Citation45]. In the CMCS spectrum, the typical bands around 3600 − 3200 cm −1 and peak at 1409 cm−1 were validated [Citation46]. In the P407 spectrum, the characteristic peaks were detected at 2890, 1467, 1344 and 1112 cm−1 because of the existence of –CH stretching, –CH2– bending, in-plane –OH bending and C–O stretching, respectively [Citation47]. In comparison to the spectrum of CMCS, the peaks in the spectrum of GP-CMCS/P407 hydrogel expressed similar in general after crosslinking. However, some differences indeed existed in detail such as smaller bands around 3600–3200 cm−1 and 1630 cm−1 corresponding to the dramatic decrease of –NH2 group in CMCS, which significantly chemically crosslinked by genipin. The results demonstrated the crosslinking reaction had taken place between amino groups of CMCS and carboxymethyl groups of genipin while no chemical reaction occurred to P407.
Morphologies of PEG-interpenetrated CMCS/P407 hydrogel
For hydrogel materials used in topical drug delivery system, the porous structure of the material has great influence on drug release profile, mainly from two aspects of pore size and interconnect structure. The porous structure with interconnect tunnels is feasible to uptake more water and improve drug release rate. presents the internal profile morphology of lyophilised PEG-interpenetrated hydrogels with none or gradient amounts of PEG 4000. As shown in the figure, none PEG-interpenetrated hydrogel possessed less porous networks and a more regular arrangement than other hydrogels contained PEG, no signs of porous on the network lateral wall was observed. Few small porous structures with the size of approximately 10 nm could be observed on the network lateral was in 0.5% PEG group, and the hydrogel matrix arrangement was similar to the none PEG group, implying such amount of PEG induced minor effect on the hydrogel inner structure. Meanwhile, various porous structure with the size of 20–40 nm generated on the network lateral wall of 1.5% and 2.0% PEG-interpenetrated hydrogel inner structure, which could be attributed to the porous forming effect of PEG. The basic structure of 2.0% PEG group matrix was looser and more porous than any other groups, indicating the porous inducing effect of PEG. Moreover, the interconnect structure of PEG-interpenetrated hydrogel was more sophisticated which could promote water absorption and drug release profile.
Figure 4. SEM images of the lyophilised PEG-interpenetrated CMCS/P407 hydrogels. (A) 0% PEG; (B) 0.5% PEG; (C) 1.5%PEG; (D) 2.0% PEG.
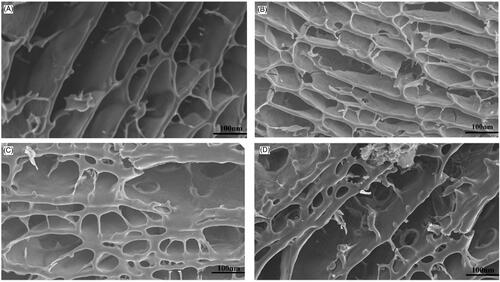
Rheological characterisation of PEG-interpenetrated CMCS/P407 hydrogel
Mechanical property is an important key factor of hydrogel for practical topical applications. Therefore, the viscosity of poly ethylene glycol hydrogel at swelling equilibrium was determined by rheological measurements. demonstrated the mechanical property of CMCS/P407 hydrogel was relatively poor, which often compromised its retention ability on dermal application. When the shear rate was below 100 (1/s), the shear stress of CMCS/P407 hydrogel obviously increased, indicating that the hydrogels interior had already crosslinked. However, when the shear rate exceeded 100 (1/s), the slope of shear stress curve of CMCS/P407 hydrogel dramatically decreased, indicating the pseudoplastic rheologic behaviour of the hydrogel. It can be observed from the figure that the shear stress of CMCS/P407 hydrogel was significantly improved along with the addition of PEG, especially at 2.0% PEG amount. The viscosity of CMCS/P407 hydrogel also escalated in accordance with the additional amount of PEG as shown in . In view of the viscosity had great influence on the hydrogel adhesivity, which is a key factor on the dermal application, the 2.0% PEG hydrogel with the highest viscosity in the tests was considered a suitable candidate for ALA-NLC carrier on dermal application.
Swelling properties of PEG-interpenetrated CMCS/P407 hydrogel
The swelling property of hydrogel is another key factor which plays an important role on drug loading and releasing efficiency in the hydrogel. Higher swelling ratio can obviously increase drug loading capacity and promote drug releasing behaviour. To investigate the hydrogel swelling attributes, the hydrogel water absorbency values were measured by gravimetric analysis method. The swelling capacity of CMCS/P407 hydrogel was tested in PBS solution under different pH (2.5 or 6.5) and temperature (25 or 35 °C), and the swelling ratio (SR) results were shown in . Data confirmed the pH-sensitive swelling attribute of CMCS/P407 hydrogel, which was attributed to the abundant carboxyl and hydroxyl groups in CMCS [Citation48]. In the aspect of pH value influence on swelling ratio, carboxyl and hydroxyl groups of CMCS remained in their neutral form (–COOH and –OH) at pH 2.5, which could form hydrogen bonds and hinder water absorption. In contrast to that, carboxyl groups of CMCS progressively ionised at pH 6.5. The increase of charge density facilitated water absorption performance by electrostatic repulsion force between ionising groups. On the other hand, it was evident that temperature had significant influence on the swelling behaviour of CMCS/P407 hydrogels. P407 is a kind of polymer with –PEO–PPO–PEO– structure. When the temperature reached 35 °C, the polymer P407 self-assembled into micelles with hydrophobic PPO blocks inside and hydrophilic PEO blocks outside. As a result, the hydrogel swelling ratio was the highest on the condition of 35 °C and pH 6.5 compared to the other conditions, demonstrating the hydrogel possessed both pH- and thermo-sensitive characteristics.
Figure 6. Swelling characteristic of the hydrogels CMCS/P407 hydrogel (a) in different test conditions (b) with gradient amount of PEG 4000 at pH 6.5, 35 °C (mean ± SD, n = 3).
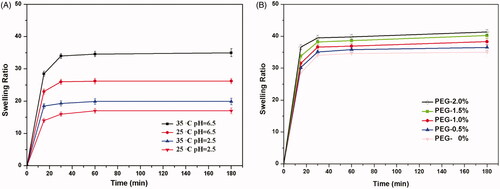
The swelling ratio results of PEG contained hydrogels were presented in , demonstrating the swelling properties could be modify by adding PEG, and 2.0% PEG hydrogel had the highest swelling property. The swelling ratio of the hydrogels gradually increased along with the increments of PEG amount. This phenomenon could be attributed to the pore-forming effect of PEG during the hydrogel immersed in PBS solution. When the hydrogel achieved diffusive equilibrium, PEG dissolved gradually into the solution and formed more pore and tunnel structure in the hydrogel, resulting in more water absorption.
In-vitro dialysis dissolution test
The in-vitro dissolution profiles of α-lipoic acid are illustrated in . The ALA solution revealed a burst release profile and completely released in 3 h due to the drug dispersed as free form in the solution. The ALA-NLC gel revealed a biphasic drug release behaviour: a burst release pattern in the initial phase and afterward a sustained release pattern. The burst release profile could be attributed to both the free form drug and the encapsulated drug released from the ALA-NLC. The biphasic release pattern is conductive to deliver topical antioxidant drug, as it promotes the rapid approach of effective drug concentration soon after application and then maintains effective drug concentration in a sustained manner to prolong drug efficacy. In addition, the release profiles revealed PEG could accelerate ALA release rate from the hydrogels, and the release rate of 2.0% PEG gel was obviously faster than the other hydrogels. ALA-NLC released more than 85% within 6 h and the cumulative amount was 94.37% at 12 h, while that from ALA-NLC gel was 74.48% at 12 h. The cumulative release amount of PEG hydrogels was 78.13% and 89.59%, corresponding to 0.5% and 2.0% PEG hydrogel respectively. The results demonstrated the hydrogel carrier could prolong the release of α-lipoic acid release compared to ALA-NLC itself, due to the barrier effect generated by complex 3 D spatial network structure in the hydrogels. Meanwhile, the released behaviour could be precisely modified by adding different amount of PEG as pore-forming agent.
Hydrogel toxicity on hUCMSCs
The biocompatibility of hydrogel was a vital factor in topical administration. CCK8 assay was applied to determine the biocompatibility of the prepared hydrogels. HUCMSCs were selected and supplied by Shenyang Kangwei Medical Laboratory co., Ltd. shows the CCK8 cell viability test results of the hydrogels. Control group was performed with hUCMSCs cultured directly in blank wells (non-treated). The cellular viability result of ALA-NLC was similar to control (non-treated) cells with ALA-NLC, demonstrating the ALA-NLC preparation revealed non-toxic to hUCMSCs. All the hydrogels performed over 85% cell viability after 24 h incubation as shown in the figure. The slight decrease in cell viability may be caused by the viscosity increment of the supernatant.
Conclusions
In this study, a series of PEG-interpenetrated CMCS/P407 hydrogel was prepared with genipin as cross-linker and different proportion of PEG4000, and the effect of PEG on rheological properties and drug release behaviour were investigated. The results have indicated that the PEG-interpenetrated CMCS/P407 hydrogel possessed both pH and thermo sensitive and fortified mechanical strength. ALA-NLC formulation with high encapsulation efficiency was prepared and successfully loaded into the hydrogel. Drug release behaviour could be modified by simply adjusted PEG amount, indicating the PEG-interpenetrated CMCS/P407 hydrogel is an effective drug delivery system for topical drug administration. CCK8 assay proved the formulation and hydrogels were biocompatible. Erythema and edoema did not occur during irritation tests on mouse skin model. In vitro permeant results showed good consistence with the in vitro dissolution test results (Supplement material). In conclusion, the prepared hydrogel revealed a good property for controlled release of alpha lipoic acid, and also possess a promising potential of water insoluble drug loading capacity for topical drug administration.
Authors’ contributions
Conceptualisation, Q.L (Qijun Li). and Y.Y (Yibin Yu); methodology and experiments, Q.L (Qijun Li), S.G (Shiqiang Gong) and W.Y (Weifan Yao); validation, C.L (Chao Liu) and R.W (Renjun Wang); writing—original draft preparation, Q.L (Qijun Li); writing—review and editing M.W (Minjie Wei) and Q.L (Qijun Li); supervision, H.P (Hao Pan) and M.W (Minjie Wei); All authors have read and agreed to the published version of the manuscript.
Supplemental Material
Download MS Word (993.1 KB)Acknowledgments
We appreciate the funding from Liaoning Province Scientific Research Foundation, grant number 20180530017.
Disclosure statement
No potential conflict of interest was reported by the author(s).
Additional information
Funding
References
- Houng WL, Lin CA, Shen JL, et al. Dihydrolipoic acid induces cytotoxicity in mouse blastocysts through apoptosis processes. Int J Mol Sci. 2012; 13(3):3988–4002.
- Brown SE, Ross MF, Sanjuan-Pla A, et al. Targeting lipoic acid to mitochondria: synthesis and characterization of a triphenylphosphonium-conjugated alpha-lipoyl derivative. Free Radic Biol Med. 2007;42(12):1766–1780.
- Salinthone S, Schillace RV, Tsang C, et al. Lipoic acid stimulates cAMP production via G protein-coupled receptor-dependent and -independent mechanisms. J Nutr Biochem. 2011;22(7):681–690.
- Wang J, Xia Q. Alpha-lipoic acid-loaded nanostructured lipid carrier: sustained release and biocompatibility to HaCaT cells in vitro. Drug Deliv. 2014;21(5):328–341.
- İnanan BE, Kanyılmaz M. Effect of alpha-lipoic acid on oxidative stress, viability and motility in the common carp (Cyprinus carpio) spermatozoa after short-term storage and cryopreservation. Cryobiology. 2020;94:73–79.
- Zhou Z, Liu C, Wan X, et al. Development of a w/o emulsion using ionic liquid strategy for transdermal delivery of anti-aging component α-lipoic acid: mechanism of different ionic liquids on skin retention and efficacy evaluation. Eur J Pharm Sci. 2020;141:105042.
- Eser Faki H, Tras B, Uney K. Alpha lipoic acid and vitamin E improve atorvastatin-induced mitochondrial dysfunctions in rats. Mitochondrion. 2020;52:83–88.
- Haidar MK, Timur SS, Kazanci A, et al. Composite nanofibers incorporating alpha lipoic acid and atorvastatin provide neuroprotection after peripheral nerve injury in rats. Eur J Pharm Biopharm. 2020;153:1–13.
- Duvvuri LS, Katiyar S, Kumar A, et al. Delivery aspects of antioxidants in diabetes management. Expert Opin Drug Deliv. 2015;12(5):827–844.
- Wang J, Tang J, Zhou X, et al. Physicochemical characterization, identification and improved photo-stability of α-lipoic acid-loaded nanostructured lipid carrier. Drug Dev Ind Pharm. 2014;40(2):201–210.
- Ruktanonchai U, Bejrapha P, Sakulkhu U, et al. Physicochemical characteristics, cytotoxicity, and antioxidant activity of three lipid nanoparticulate formulations of alpha-lipoic acid. AAPS PharmSciTech. 2009;10(1):227–234.
- Niamprem P, Srinivas SP, Tiyaboonchai W. Optimization of indomethacin loaded nanostructured lipid carriers. Asian J Pharm Sci . 2016;11(1):174–175.
- Battaglia L, Serpe L, Foglietta F, et al. Application of lipid nanoparticles to ocular drug delivery. Expert Opin Drug Deliv. 2016;13(12):1743–1757.
- Beloqui A, Solinís MÁ, Rodríguez-Gascón A, et al. Nanostructured lipid carriers: promising drug delivery systems for future clinics. Nanomedicine. 2016;12(1):143–161.
- Qi R, Li Y-z, Chen C, et al. G5-PEG PAMAM dendrimer incorporating nanostructured lipid carriers enhance oral bioavailability and plasma lipid-lowering effect of probucol. J Control Release. 2015;210:160–168.
- Sun M, Nie S, Pan X, et al. Quercetin-nanostructured lipid carriers: characteristics and anti-breast cancer activities in vitro. Colloids Surf B Biointerfaces. 2014;113:15–24.
- Fathi M, Mozafari MR, Mohebbi M. Nanoencapsulation of food ingredients using lipid based delivery systems. Trends Food Sci Technol. 2012;23(1):13–27.
- Yu Y, Feng R, Yu S, et al. Nanostructured lipid carrier-based pH and temperature dual-responsive hydrogel composed of carboxymethyl chitosan and poloxamer for drug delivery. Int J Biol Macromol. 2018;114:462–469.
- Senna JP, Barradas TN, Cardoso S, et al. Dual alginate-lipid nanocarriers as oral delivery systems for amphotericin B. Colloids Surf B Biointerfaces. 2018;166:187–194.
- Yu Y, Xu S, Yu S, et al. A hybrid genipin-cross-linked hydrogel/nanostructured lipid carrier for ocular drug delivery: Cellular, ex vivo, and in vivo evaluation. ACS Biomater Sci Eng. 2020;6(3):1543–1552.
- Tan G, Yu S, Li J, et al. Development and characterization of nanostructured lipid carriers based chitosan thermosensitive hydrogel for delivery of dexamethasone. Int J Biol Macromol. 2017;103:941–947.
- Colinet I, Dulong V, Mocanu G, et al. New amphiphilic and pH-sensitive hydrogel for controlled release of a model poorly water-soluble drug. Eur J Pharm Biopharm. 2009;73(3):345–350.
- Qiu Y, Park K. Environment-sensitive hydrogels for drug delivery. Adv Drug Deliv Rev. 2001;53(3):321–339.
- Bajpai AK, Shukla SK, Bhanu S, et al. Responsive polymers in controlled drug delivery. Prog Polym Sci . 2008;33(11):1088–1118.
- Ito T, Takami T, Uchida Y, et al. Chitosan gel sheet containing drug carriers with controllable drug-release properties. Colloids Surf B Biointerfaces. 2018;163:257–265.
- Finosh GT, Jayabalan M. Hybrid amphiphilic bimodal hydrogels having mechanical and biological recognition characteristics for cardiac tissue engineering. RSC Adv. 2015;5(48):38183–38201.
- Finosh GT, Jayabalan M, Vandana S, et al. Hybrid alginate-polyester bimodal network hydrogel for tissue engineering-Influence of structured water on long-term cellular growth. Colloids Surf B Biointerfaces. 2015;135:855–864.
- Thankam FG, Muthu J. Influence of physical and mechanical properties of amphiphilic biosynthetic hydrogels on long-term cell viability. J Mech Behav Biomed Mater. 2014;35:111–122.
- Sarker B, Papageorgiou DG, Silva R, et al. Fabrication of alginate-gelatin crosslinked hydrogel microcapsules and evaluation of the microstructure and physico-chemical properties. J Mater Chem B. 2014;2(11):1470–1482.
- Li J, Liu D, Tan G, et al. A comparative study on the efficiency of chitosan-N-acetylcysteine, chitosan oligosaccharides or carboxymethyl chitosan surface modified nanostructured lipid carrier for ophthalmic delivery of curcumin. Carbohydr Polym. 2016;146:435–444.
- Huh HW, Zhao L, Kim SY. Biomineralized biomimetic organic/inorganic hybrid hydrogels based on hyaluronic acid and poloxamer. Carbohydr Polym. 2015;126:130–140.
- Dewan M, Bhowmick B, Sarkar G, et al. Effect of methyl cellulose on gelation behavior and drug release from poloxamer based ophthalmic formulations. Int J Biol Macromol. 2015;72:706–710.
- Arranja A, Schroder AP, Schmutz M, et al. Cytotoxicity and internalization of Pluronic micelles stabilized by core cross-linking. J Control Release. 2014;196:87–95.
- Eslahi N, Simchi A, Mehrjoo M, et al. Hybrid cross-linked hydrogels based on fibrous protein/block copolymers and layered silicate nanoparticles: tunable thermosensitivity, biodegradability and mechanical durability. RSC Adv. 2016;6(67):62944–62957.
- Lewandowska-Łańcucka J, Gilarska A, Buła A, et al. Genipin crosslinked bioactive collagen/chitosan/hyaluronic acid injectable hydrogels structurally amended via covalent attachment of surface-modified silica particles. Int J Biol Macromol. 2019;136:1196–1208.
- Wu T, Liao W, Wang W, et al. Genipin-crosslinked carboxymethyl chitosan nanogel for lung-targeted delivery of isoniazid and rifampin. Carbohydr Polym. 2018;197:403–413.
- Dragan ES. Design and applications of interpenetrating polymer network hydrogels. A review. Chem Eng J. 2014;243:572–590.
- Liu S, Cao H, Guo R, et al. Effects of the proportion of two different cross-linkers on the material and biological properties of enzymatically degradable PEG hydrogels. Polym Degrad Stab . 2020;172:109067.
- Bakaic E, Smeets NMB, Hoare T. Injectable hydrogels based on poly(ethylene glycol) and derivatives as functional biomaterials. RSC Adv. 2015;5(45):35469–35486.
- Galarraga JH, Burdick JA. Moving hydrogels to the fourth dimension. Nat Mater. 2019;18(9):914–915.
- Broguiere N, Husch A, Palazzolo G, et al. Macroporous hydrogels derived from aqueous dynamic phase separation. Biomaterials. 2019;200:56–65.
- Yu Y, Feng R, Li J, et al. A hybrid genipin-crosslinked dual-sensitive hydrogel/nanostructured lipid carrier ocular drug delivery platform. Asian J Pharm Sci. 2019;14(4):423–434.
- Lasoń E, Sikora E, Miastkowska M, et al. NLC delivery systems for alpha lipoic acid: physicochemical characteristics and release study. Colloids Surf A . 2017;532:57–62.
- Yang L-q, Lan Y-q, Guo H, et al. Ophthalmic drug-loaded N,O-carboxymethyl chitosan hydrogels: synthesis, in vitro and in vivo evaluation. Acta Pharmacol Sin. 2010;31(12):1625–1634.
- Zhao J, Yan Y, Shang Y, et al. Thermosensitive elastin-derived polypeptide hydrogels crosslinked by genipin. Int J Polym Mater Polym Biomater. 2017;66(8):369–377.
- Wu S, Butt H-J. Near-infrared photochemistry at interfaces based on upconverting nanoparticles. Phys Chem Chem Phys. 2017;19(35):23585–23596.
- Bujňáková Z, Dutková E, Baláž M, et al. Stability studies of As4S4 nanosuspension prepared by wet milling in Poloxamer 407. Int J Pharm. 2015;478(1):187–192.
- Chen S-C, Wu Y-C, Mi F-L, et al. A novel pH-sensitive hydrogel composed of N,O-carboxymethyl chitosan and alginate cross-linked by genipin for protein drug delivery. J Control Release. 2004;96(2):285–300.