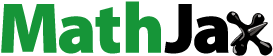
Abstract
Adhesion bands are pathological fibrous tissues that create in the middle of tissues and organs, often reasons of intestinal obstruction, and female infertility. Here, we explored the anti-adhesive and inflammatory capacities of PEG/silk and Ibuprofen-loaded PEG/Silk core-shell nanofibrous membranes, respectively. The ibuprofen-loaded Silk Fibroin-Poly ethylene Glycol (SF-PEG) core-shell membrane was fabricated by electrospinning and considered in terms of morphology, surface wettability, drug release, and degradation. To reveal the membrane capability for adhesion bands inhibition, the membrane was stitched among the abdominal partition and peritoneum and then evaluated using two scoring adhesion systems. According to results, the fibrous membrane hindered cell proliferation, and the scoring systems and pathology showed that in a rat model, Ibuprofen-loaded PEG/Silk core-shell membrane caused a lightening in post-operative adhesion bands and the low-grade inflammatory reaction in animal models. Collectively, we fabricated new ibuprofen-loaded PEG/SF membranes with anti-adhesion and anti-inflammation properties. Moreover, this core-shell electrospun fibrous membrane has not even now been used to prevent peritendinous adhesion generation.
Introduction
An adhesion (a band of wound tissue) attaches two portions of tissue jointly [Citation1] that routinely go along with tissue injury, pain, functional obstruction, foreign body reaction, infection, bleeding [Citation1], and the request for a second surgical mediation [Citation2,Citation3]. The most joint locations of adhesion are within the stomach, the pelvis, and the heart. Peritoneal adhesions are a serious difficulty that may consist of female reproductive organs, leading to infertility, chronic pelvic pains, and may progress after any kind of abdominal or pelvic surgery [Citation4]. Modified surgical techniques, novel biomaterials as barriers, and new rehabilitation protocols have been defined for adhesion prevention [Citation5].
Although numerous physical membranes have been used as hurdles to keep down adhesion formation, there are many problems in using these membranes for adhesion prevention in patients such as late-onset postoperative pain, organ adherence, and foreign body reactions [Citation2,Citation6–9], not removed from the place of location and demand to be detached laparoscopically, rapid degradation time (in less than one week) in vivo, [Citation10,Citation11] the inflammation reactions and the delayed trafficking of nutrients and wastes at the surgical place [Citation12].
To date, nanofibrous membranes (PCL, PLGA, PLLA- PEG tri-block copolymer, chitosan/alginate polymers) have been described for the anti-adhesion barrier [Citation13–15]. An electrospinning technique is a straightforward method for the creation of a nanofibrous membrane (membranes) from various materials and polymers [Citation16].
There are some great attractive properties of silk such as biocompatibility, appropriate controllable degradability, mechanical, and physical outstanding structures which signify a great biomaterial for the medical and pharmaceutical industry [Citation17]. The silk fibroin (SF) is accepted as a biomaterial for medical uses that is easily absorbable by the skin tissue [Citation18]. PEG is a famous studied biocompatible and amphiphilic polymer and known as a high structure [Citation19–21]. Some properties including low immune cell activation, low cellular intake, and negligible inflammation are among the key properties that are considered in medical uses [Citation22].
Severe inflammatory reaction to surgery is a hazard element for adhesion generation [Citation23]. In spite of the early capacity revealed by fence matters in medical utilization, ingestible membranes frequently degrade quickly, occasionally persuading a serious foreign-body reaction [Citation24]. Inflammatory cells and fibroblasts move to the location of the wound and novel arteries generate throughout the proceeding of adhesion generation [Citation25]. Ibuprofen picks out the inflammatory stage of healing to diminish adhesions, but Ibuprofen solely cannot wholly prevent adhesion generation by reason of their quick clearance and capacity for serious aftereffects once taken orally [Citation26].
Here, ibuprofen-loaded core/shell membranes produced by the coaxial electrospinning method were assessed for the capability to keep down adhesion generation and inflammation created by surgical procedure and material degradation. The electrospun ibuprofen-loaded PEG/Silk membranes were specified and assessed in vitro and in vivo for their capability to block tissue adhesion via coacting anti-adhesion and anti-inflammatory efficacies. We focussed on the fabrication of a new ibuprofen-loaded PEG/silk fibroin nanofibrous membrane with anti-adhesive and anti-inflammatory potential using coaxial electrospinning methods.
Materials and methods
Production of silk fibroin & fabrication of fibrous membrane
The slices of Bombyx mori SF was dissolved in boiling distilled water containing Na2CO3, washed, dried overnight and then, dissolved in another solvent (distilled water: ethanol: calcium chloride), keep warm in the oven (60 °C for 4 h), and located in cellulose membrane (Pore size: 14 000 Mw; Sigma) at 4 °C. In the end, the solutions were freeze‐dried (freeze dryer Alpha) for 4 days [Citation27]. For the fabrication of nanofibrous membrane, SF polymer (10% w/v) was dissolved in formic acid, PEG (MW: 20,000, 25 w/v %) and 20 mg ibuprofen (Aviscera Bioscience, USA) were dissolved in deionised water. The PEG and SF solutions were transferred to the coaxial needle (22 and 16 Gage) with a selectable syringe pump (24‐kV high voltage, 220‐mm distance, 0.1 ml/h flow rate, (2500 rpm) speed rotating drum, 35–37% of humidity and 24–26 °C temperature). Then, the electrospun fibrous membranes were floated in methanol (20 min) to produce a beta-sheet structure.
Surface properties of fibrous membrane
The fibrous membrane was coated with gold by a sputter machine and then scanned by a scanning electron microscope (Seron technology, AIS2100). The membranes were developed by sticking the fibres onto grids, dried in a vacuum before transmission electron microscopy (TEM) (EM10C, Zeiss) imaging.
FTIR and contact angle analysis
The chemical bond structure of the membrane was explored by attenuated total reflectance–Fourier transforms infra-red spectroscopy (ATR–FTIR). The contact angle measuring system in the sessile drop method was used to assess the hydrophilicity of the membranes (G10, KRUSS, Germany). The measurements were performed for each membrane (four times) and the average values were determined.
In vitro degradation of fibrous membrane
Nanofibrous membrane (15 mm × 15mm) was cut into rectangles shape, sterilised under UV irradiation for 2 h, placed in a plate containing PBS solution, and incubated at 37 °C (95% humidity and 5% CO2). The incubation medium was replaced by a fresh PBS solution every 2 weeks. The membranes (Six scaffolds of each degradation period) were washed, dried, and their weights were measured. Furthermore, an SEM image of the membrane throughout the 8 weeks of degradation was obtained. The degradation ratio of the membrane after divergent periods of degradation was determined. The degradation ratio as Degradation ratio = initial dry weight – dry weight after degradation/initial dry weight.
Porosity and mechanical properties of membrane
The porosity of SF/PEG membranes was measured by the liquid displacement method. The membrane was immersed in hexane for 5 min and removed. The porosity was obtained by [Citation28]: % ε = v1: the volume of hexane, v2: the volume of hexane while the membrane is immersed in hexane and v3: the volumes of hexane remaining after removing of the membrane. The tensile strength and elongation of the membranes (30 × 10mm, n = 5) were measured by a mechanical testing system (Model STM-20, SANTAM, Iran). The accuracy of the measurement is within ± 5%.
Drug release from the membrane
The fibrous membrane was punched (3 × 3 cm2), immersed in PBS medium (10 ml, pH = 7.4), and maintained in an incubator shaker (37 °C at 100 rpm). At programmed time frames, 500 µL of the PBS was collected and stored at −70 °C. The quantity of the released drug was sensed by ultraviolet-visible spectrophotometry.
Cell proliferation assay
MCF7 cell line (purchased from Pasture Institute Cell Bank, Iran) proliferation on the membrane (1.5 cm2) was assessed by MTT test. The membranes (After 3, 5, and 7 days of cell culturing) were washed with PBS and incubated (3 h at 37 °C) with MTT solution (5 mg/mL in DMEM). DMSO solvent was added, the suspension moved into a reader plate, and the absorbance peruses at 570 nm.
Adhesion formation evaluation
All work on rat animals was conducted with the formal approval of the animal care committees of the National Institute for Medical Research and Development (Ethics No: IR NIMAD REC. 1396. 008). After anaesthetic induction, the abdomen opened, the caecum disclosed and then softly abraded by dry gauze pads at all faces up to it loses its polish, and haemorrhagic areas become observable. Then one 1.5 cm section of fibrous membrane was placed to the abdominal partition sandwiched between the abdominal and peritoneum. After 1 wk, the rats (n = 6 for each group: untreated control group, the PEG/SF group, the Ibuprofen–loaded PEG/SF group) were euthanized, their abdominal chambers wide opened, and the post-surgical adhesion bands evaluated based on scores (created on two scales described by Zuhlke et al. and Duran et al. () [Citation29,Citation30]).
Table 1. Adhesion and inflammation grade score.
Histological and inflammation evaluation
Afterward, the adhesion band formation analysis, the membranes (fibrous membrane) and the peritoneal tissues were removed, fixed (10% formalin), and immersed in paraffin. These segments were stained with Haematoxylin–Eosin (HE) and the score of inflammation was assessed via a semiquantitative scoring system () [Citation31].
Statistical analysis
Statistical software SPSS 10.0 was used to examine the documents by one-way Anova analysis of variance (p < .05). All data were stated as mean ± SD.
Results
Preparation and characterisation of electrospun fibrous PEG/SF
In coaxial electrospinning, the special nozzles (at the same time) with two diverse solutions were used (PEG and Ibuprofen as an internal layer and SF as the external layer). The keen borderlines in the TEM images designated the border between the core and shell layers. displays the dark core layer and the light-coloured shell layer in the fibre. The morphology and diameter frequency of PEG/SF fibrous membrane fabricated by coaxial electrospinning are shows in . The FTIR spectra of PEG, SF, and PEG/SF scaffold were publicised in . The peaks located at 1633 and 1553 cm‐1 of SF, and PEG -SF membrane, are related to Amide I and Amide II respectively. The signals located at 3440, 2885, and 1102 cm‐1 of PEG were assigned to the stretching vibration of -OH, C-H, and C-O-C. These peaks prove that PEG/SF fibrous membrane may be designed. The water contact angle for PEG-SF fibrous membrane was 112–126° ().
Degradation and weight loss assay of PEG/SF
The weight loss measurement was carried out for 60 days. The result showed that there was a difference with regards to their weight loss of fibrous membrane on different days of the period. At days 15 and 60, the weight loss of the PEG/SF membrane is 7.9%, 12.4%, and ibuprofen-loaded PEG/SF fibrous membrane is 10.6%, 12.8%, respectively ().
Figure 4. The molecular weight of electrospun fibrous scaffolds containing ibuprofen drug, after keeping warm in PBS medium.
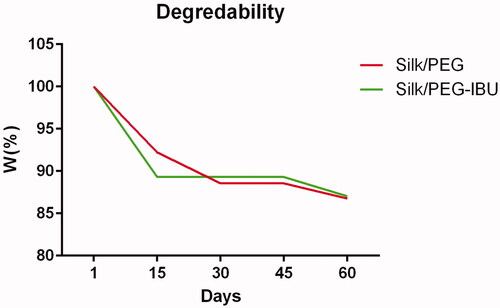
Furthermore, the morphology changes of the fibrous membrane (after degradation in PBS media) were examined using SEM imaging at different times. As shown in , the morphology changes of the PEG/SF and ibuprofen-loaded PEG/SF fibrous membranes after degradation in PBS media at several time points. After 15 days of degradation, no different structures with their unique except for minor swelling properties presented in all fibrous membranes. After 30 days of degradation, PEG/SF and ibuprofen-loaded PEG/SF fibrous membrane presented definite swelling, and the fibres start to join while still no morphology alterations were detected on the surface of nanofibers. After 60 days of degradation, some fibres start to look breakage and fracture, and almost the pores of the surface fibres were enveloped due to the swelling effect.
Porosity and tensile property of fibrous membrane
The porosity was studied by the liquid displacement method (). The data indicated that porosity is 78% for PEG/SF core-shell fibrous membrane. However, there was a notable difference (up to 20%; p < .05) between the porosity of the SF fibrous membrane (96%) with that of core-shell membrane. The membrane exhibited a tensile strength of 1.5 ± 0.2 Neoton (N) and elongation at a break of 20.80 ± 2.84% ().
Release profiles of ibuprofen from PEG/SF core-shell fibrous membrane
The release profile (cumulative pattern) of ibuprofen filled in the PEG/SF core-shell nanofibrous were quantified as the release portion of the whole ibuprofen burden inside the fibers over 14 days is shown in . About 42% of loaded ibuprofen was released within about 4 h and then 57% of total Ibuprofen has been released for 48 h. On the 10th day (240 h), and 16th day (384 h), almost 69%, and 70% of the whole amount of ibuprofen was delivered from PEG/SF fibrous membrane inside the surrounding medium, respectively.
In vitro cell adhesion and proliferation on fibrous membrane
MTT assay was used for investigating the cell viability on the fibrous membrane after 3, 5, and 7 days. The cell proliferation on tissue culture plastic surfaces (TCPS) was significantly higher than on membrane (SF, PEG/SF, and Ibuprofen-PEG/SF). The result presented that the cell viability in Ibu-PEG/SF was lower than in the PEG/SF after 7 days of culture ().
The grade of adhesion bands
According to Zuhlke et al. The adhesion score in the untreated group was 2.8 ± 1.4; in PEG-SF: 0.2 ± 0.4; in Ibuprofen-loaded PEG/SF: 0.16 ± 0.4. Notable divergences were recorded between the adhesion score in PEG/SF, and ibuprofen-loaded PEG/SF correlative to the untreated control sample (p < .05). There was no difference reduction between PEG/SF, and ibuprofen-loaded PEG/SF. Also, similar results were detected for the scores of adhesion bands according to Duran et al. scoring system. According to these analyses, it seems that in the rat that is given the fibrous membrane, adhesion bands were reduced and little abdominal adhesion bands were produced in the injured abdominal sites ().
Histological and inflammation evaluation
After 1 wk, the inflammation scores in every experimental group were assessed (). The mean ± SD of scores in the untreated group (control) was 1.5 ± 1.04; in the PEG/SF group, 2.4 ± 0.5; in the ibuprofen-loaded PEG/SF group, 1.8 ± 0.9. Although the inflammation grades in the membrane were higher than the untreated group (Control) but no notable divergences were seen between their groups. Compared with PEG/SF groups, the decrease of inflammation scores following the incorporation of ibuprofen-loaded PEG/SF fibrous membrane was detected. Histological study was done to more prospect the role of the core-shell fibrous membrane in the decrease of the inflammation score. However, the result showed that inflammatory cells such as neutrophils, lymphocytes, giant cells, and plasma cells were presented nearby the peritoneal superficial ().
Discussion
Adhesions cause functional obstruction, pain, and problems in operative surgery. In this study, ibuprofen-PEG/SF core-shell membrane was fabricated, and biomaterial, mechanical, surface properties of its, and the release outlines of ibuprofen drug were examined.
The loaded ibuprofen drug in the internal layer of fibres is constituted of PEG polymer. This polymer has biocompatible, has low toxicity, displays excessive fibre-forming capacity, and improved the stability of the drug and is a water-soluble matter that is used in a range of issues [Citation32,Citation33].
Now, we have created the core-shell structured PEG/SF fibrous membrane made via the coaxial electrospinning approach. The FTIR and TEM analysis established the construction of the PEG within the SF, and core-shell fibrous membrane.
In the current project, the PEG/SF core-shell fibrous membrane with good porosity and submicron pore size has been fabricated by electrospinning. Due to its high porosity, submicrometer pore size, and good permeability, this membrane allows the passage of nutrients and wastes [Citation25]. The porosity of the membrane is an important value to support growth, differentiation, and metabolite diffusion for cells and tissue [Citation34,Citation35]. However, there was a significant difference (up to 20%) between the porosity of the SF fibrous membrane (96%) with that of the PEG/SF core-shell membrane (78%). Our result seems to be supported by a previous report in which porosity was altered from 86.4% in poly-caprolactone (PCL) pure membrane to 77.9% in modified membranes [Citation34–36]. However, it seems that the extremely porous 3-dimensionally interconnected fibre structure in PEG/SF core/shell fibrous membrane was collapsed, adhered together, and decreased due to the incorporation of PEG polymer in the core of the membrane.
The best mechanical barrier for anti-adhesion functionality should be safe and stable in place without the need for sutures. The enhanced flexibility permits the anti-adhesion membranes to be more simply handled by surgeons [Citation37]. In this work, we fabricated a fibrous membrane from PEG/SF, and the incorporation of the PEG to the core part of the fibrous membrane increases the flexibility of SF and proper use and handling of the membranes.
The physical and chemical properties of the membrane, such as contact angles and hydrophobicity, are essential criteria for their demand as anti-adhesive membranes. The hydrophobicity of the anti-adhesive membranes takes part in a great character in preventing adhesion bands due to the avoidance of adsorption of cells on the membrane [Citation38]. In the present study, we fabricated membranes with hydrophobic surfaces, and the diminished quantity of cell attachment and proliferation are seen on PEG/SF membranes surfaces. An anti-adhesive membrane needs to own appropriate surface hydrophilicity. However, the diminished quantity of cell attachment is frequently noticed on membranes with a high hydrophobic nature [Citation14]. The cells more freely adhere on fairly hydrophilic surfaces than on hydrophobic [Citation39]. The contact angle of the PEG-SF core-shell fibrous membrane (112°–123°), indicates that the membrane surface was very hydrophobic. However, the electrospinning process increases surface hydrophobicity of fibrous membrane due to air entrapment between fibre interfaces throughout electrospinning [Citation40]. Furthermore, the methanol treatment of SF induced the formation of β-sheet and hydrophobic domains in polymer, therefore, impacting its capability to upkeep the cell adhesion [Citation18,Citation41]. Here, PEG/SF, fabricated by coaxial electrospinning, can more efficiently block cell and tissue adhesion and was consequently taken for this project. The avoid mechanical contact between tissues to reduce adhesion bands and to decrease the creation of a fibrin matrix between tissue and membrane is the main objective of using membrane around the damaged site [Citation42].
Biodegradation of membrane is a critical factor for using a peritoneal anti-adhesion barrier [Citation43]. The toughness of the semi-crystalline structure of silk fibres is reliant on their β-sheet composition and the association between the content of (random coil and a-helix) and (B-sheets), and the rate of degradation has been reported [Citation44]. The structural changes of silk fibre can also be induced by heat treatment, mechanical deformation, or organic solvents. In this work, methanol has been used to prompt a change from silk I (a-helix; random coil) to silk II (more B-sheets) [Citation45–47]. In the present study, the morphology changes and breaking of the PEG/SF and ibuprofen-loaded PEG/SF fibrous membrane after degradation in PBS media were documented. PBS solutions match with those of the human body due to having the same osmolarity and ion concentrations [Citation43].
The fibrous membrane has a good capability for applying as physical membranes, and drug delivery systems. A varied range of antibiotics, anti-inflammatory and fibrinolytic drugs have been exerted as drug delivery methods that decrease adhesions and hinder inflammation [Citation48–51]. In the current project, we examined the capacity of the PEG/SF fibrous membranes with ibuprofen fabricated via coaxial electrospinning to diminish the inflammatory reaction after surgery. Inflammation is a procedure mostly detected during the early phases of adhesion band creation. To the anti-adhesive exclusivity, a perfect physical fence must to have the least capacity to prompt inflammatory reaction throughout its in vivo entertainment. The low inflammation was identified in the adhesive tissues in animal rats cured with PEG/SF membranes membrane. Overall, we have revealed that the PEG/SF fibrous membranes prevent the adhesion bands compared with uncured animals. It is also indicative that the location of PEG/SF fibrous membranes between the peritoneum and abdominal partition caused a decline in the inflammation reaction. According to our results, the PEG/SF nanofibrous membranes can be appealed as an anti-adhesive substrate for falling postsurgical adhesion bands. However, due to the benefits of the electrospinning method such as straightforwardness, low cost, and informal handling for the fabrication of fibrous membrane, it appears that this method is useful for the marketable creation of the membranes for application as anti-adhesive mediators [Citation14].
Electrospun fibrous membranes are operative for tissue parting and drug delivery due to their great superficial area and high porosity [Citation52,Citation53]. The porous structure permits the path of nutrients from external the tissue to stimulate inherent healing [Citation25]. In the present work, bilayer nanofibrous membranes with an ibuprofen-loaded PEG inner membrane layer and an SF outer membrane layer have been investigated as an anti-inflammatory reaction. The electrospun fibrous membrane allows the constant release of ibuprofen from the PEG layer after the dispersion of it’s from the SF layer (about 30% and 50% within 4 h and 48 h, respectively). It is anticipated that the residual ibuprofen will release in a sustained time. In our study, the initial burst release of ibuprofen (42%, and 57% during the first 2 days) was continued by a sustained release phase (69% and 70%) in the following 14 days of incubation. Ibuprofen can decrease the inflammatory reaction to trauma, keep away from the emigration of inflammatory cells, and decrease vessel enlargement in the hurt region, so decreasing tissue adhesion [Citation24,Citation54]. After ibuprofen is incorporated into PEG/SF nanofibers, ibuprofen can be firmly delivered to reduce inflammation, therefore decreasing tissue inflammation. In this study, histology and inflammation score systems data confirmed that the inflammatory cell infiltration and inflammation score was diminished by the ibuprofen-loaded PEG/SF membrane. Also, peritoneal adhesions were diminished by the PEG/SF and ibuprofen-loaded PEG/SF fibrous membrane. Former projects have shown that ibuprofen-filled alginate hydrogel [Citation24,Citation55], hyaluronic acid gel [Citation56], and solvent-casting PELA film [Citation24] are used as physical fences and drug delivery methods diminish adhesions and hinder inflammation. Though, the fast degradation capacity of these materials limits their use in preventing adhesions. The current work focal points on the anti-adhesion capacities of ibuprofen-loaded PEG/SF membranes. Our result confirmed that the fabricated fibrous scaffold not only takes actions as a physical fence to inhibit peritoneal adhesions but also hinders inflammation.
Conclusion
In the present study, we fabricated new ibuprofen-loaded PEG/SF membranes with anti-adhesion and anti-inflammation properties. Moreover, this core-shell electrospun fibrous membrane has not even now been used to prevent peritendinous adhesion creation.
Disclosure statement
There is no conflict of interest in this study.
Additional information
Funding
References
- Cheong YC, Laird SM, Li TC, et al. Peritoneal healing and adhesion formation/reformation. Hum Reprod Update. 2001;7(6):556–566.
- diZerega GS, Campeau JD. Peritoneal repair and post-surgical adhesion formation. Hum Reprod Update. 2001;7(6):547–555.
- Taras JS, Lamb MJ. Treatment of flexor tendon injuries: surgeons' perspective. J Hand Ther. 1999;12(2):141–148.
- Kamel RM. Prevention of postoperative peritoneal adhesions. Eur J Obstet Gynecol Reprod Biol. 2010;150(2):111–118.
- James R, Kesturu G, Balian G, et al. Tendon: biology, biomechanics, repair, growth factors, and evolving treatment options. J Hand Surg Am. 2008;33(1):102–112.
- Pagidas K, Tulandi T. Effects of ringer's lactate, interceed(TC7) and Gore-Tex surgical membrane on postsurgical adhesion formation. Fertil Steril. 1992;57(1):199–201.
- March CM, Boyers S, Franklin R, et al. Prevention of adhesion formation/reformation with the Gore-Tex surgical membrane. Prog Clin Biol Res. 1993;381:253–259.
- Rice VM, Shanti A, Moghissi KS, et al. A comparative evaluation of poloxamer 407 and oxidized regenerated cellulose (interceed [TC7]) to reduce postoperative adhesion formation in the rat uterine horn model. Fertil Steril. 1993;59(4):901–906.
- diZerega GS, Verco SJ, Young P, et al. A randomized, controlled pilot study of the safety and efficacy of 4% icodextrin solution in the reduction of adhesions following laparoscopic gynaecological surgery. Hum Reprod. 2002;17(4):1031–1038.
- Drollette CM, Badawy SZ. Pathophysiology of pelvic adhesions. Modern trends in preventing infertility. J Reprod Med. 1992;37(2):107–121. discussion 21–2.
- Becker JM, Dayton MT, Fazio VW, et al. Prevention of postoperative abdominal adhesions by a sodium hyaluronate-based bioresorbable membrane: a prospective, randomized, double-blind multicenter study. J Am Coll Surg. 1996;183:297–306.
- Chen SH, Chen CH, Fong YT, et al. Prevention of peritendinous adhesions with electrospun chitosan-grafted polycaprolactone nanofibrous membranes. Acta Biomater. 2014;10(12):4971–4982.
- Yang DJ, Chen F, Xiong ZC, et al. Tissue anti-adhesion potential of biodegradable PELA electrospun membranes. Acta Biomater. 2009;5(7):2467–2474.
- Dinarvand P, Hashemi SM, Seyedjafari E, et al. Function of poly (lactic-co-glycolic acid) nanofiber in reduction of adhesion bands. J Surg Res. 2012;172(1):e1–e9.
- Zong X, Li S, Chen E, et al. Prevention of postsurgery-induced abdominal adhesions by electrospun bioabsorbable nanofibrous poly(lactide-co-glycolide)-based membranes. Ann Surg. 2004;240(5):910–915.
- Min BM, Lee G, Kim SH, et al. Electrospinning of silk fibroin nanofibers and its effect on the adhesion and spreading of normal human keratinocytes and fibroblasts in vitro. Biomaterials. 2004;25(7–8):1289–1297.
- Vollrath F, Knight DP. Liquid crystalline spinning of spider silk. Nature. 2001;410(6828):541–548.
- Melke J, Midha S, Ghosh S, et al. Silk fibroin as biomaterial for bone tissue engineering. Acta Biomater. 2016;31:1–16.
- Harris JM, Chess RB. Effect of pegylation on pharmaceuticals. Nat Rev Drug Discov. 2003;2(3):214–221.
- Pasut G, Veronese FM. State of the art in PEGylation: the great versatility achieved after forty years of research. J Controlled Release. 2012;161(2):461–472.
- Xiao X-F, Jiang X-Q, Zhou L-J. Surface modification of poly ethylene glycol to resist nonspecific adsorption of proteins. Chin J Anal Chem. 2013;3:445–453.
- D'souza AA, Shegokar R. Polyethylene glycol (PEG): a versatile polymer for pharmaceutical applications. Expert Opin Drug Deliv. 2016;13(9):1257–1275.
- Lee JH, Kim KO, Ju YM. Polyethylene oxide additive-entrapped polyvinyl chloride as a new blood bag material. J Biomed Mater Res. 1999;48(3):328–334.
- Lee JH, Go AK, Oh SH, et al. Tissue anti-adhesion potential of ibuprofen-loaded PLLA-PEG diblock copolymer films. Biomaterials. 2005;26(6):671–678.
- Bhavsar D, Shettko D, Tenenhaus M. Encircling the tendon repair site with collagen-GAG reduces the formation of postoperative tendon adhesions in a chicken flexor tendon model. J Surg Res. 2010;159(2):765–771.
- Tan V, Nourbakhsh A, Capo J, et al. Effects of nonsteroidal anti-inflammatory drugs on flexor tendon adhesion. J Hand Surg Am. 2010;35(6):941–947.
- Rahmani A, Nadri S, Kazemi HS, et al. Conductive electrospun scaffolds with electrical stimulation for neural differentiation of conjunctiva mesenchymal stem cells. Artif Organs. 2019;43(8):780–790.
- Li JJ, Gil ES, Hayden RS, et al. Multiple silk coatings on biphasic calcium phosphate scaffolds: effect on physical and mechanical properties and in vitro osteogenic response of human mesenchymal stem cells. Biomacromolecules. 2013;14(7):2179–2188.
- Zuhlke HV, Lorenz EM, Straub EM, et al. Pathophysiology and classification of adhesions. Langenbecks Archiv Fur Chirurgie Supplement II, Verhandlungen Der Deutschen Gesellschaft Fur Chirurgie Deutsche Gesellschaft Fur Chirurgie Kongress. 1990;1:1009–1016.
- Duran B, Ak D, Cetin A, et al. Reduction of postoperative adhesions by N,O-carboxymethylchitosan and spermine NONOate in rats. Exp Anim. 2003;52(4):267–272.
- Hooker GD, Taylor BM, Driman DK. Prevention of adhesion formation with use of sodium hyaluronate-based bioresorbable membrane in a rat model of ventral hernia repair with polypropylene mesh–a randomized, controlled study. Surgery. 1999;125(2):211–216.
- Janse van Rensburg A, Davies NH, Oosthuysen A, et al. Improved vascularization of porous scaffolds through growth factor delivery from heparinized polyethylene glycol hydrogels. Acta Biomater. 2017;49:89–100.
- Wang W. Instability, stabilization, and formulation of liquid protein pharmaceuticals. Int J Pharm. 1999;185(2):129–188.
- Yin H-M, Qian J, Zhang J, et al. Engineering porous poly (lactic acid) scaffolds with high mechanical performance via a solid state extrusion/porogen leaching approach. Polymers. 2016;8(6):213.
- Karageorgiou V, Kaplan D. Porosity of 3D biomaterial scaffolds and osteogenesis. Biomaterials. 2005;26(27):5474–5491.
- Jing X, Mi H-Y, Cordie T, et al. Fabrication of porous poly (ε-caprolactone) scaffolds containing chitosan nanofibers by combining extrusion foaming, leaching, and freeze-drying methods. Ind Eng Chem Res. 2014;53(46):17909–17918.
- Liu S, Hu C, Li F, et al. Prevention of peritendinous adhesions with electrospun ibuprofen-loaded poly(L-lactic acid)-polyethylene glycol fibrous membranes. Tissue Eng Part A. 2013;19(3–4):529–537.
- Zhu Y, Gao C, Liu X, et al. Surface modification of polycaprolactone membrane via aminolysis and biomacromolecule immobilization for promoting cytocompatibility of human endothelial cells. Biomacromolecules. 2002;3(6):1312–1319.
- Wang Y, Ji Y, Zhao Y, et al. Effects of surface functional groups on proliferation and biofunction of schwann cells. J Biomater Appl. 2016;30(10):1494–1504.
- Zhu X, Cui W, Li X, et al. Electrospun fibrous mats with high porosity as potential scaffolds for skin tissue engineering. Biomacromolecules. 2008;9(7):1795–1801.
- Kundu SC, Kundu B, Talukdar S, et al. Invited review nonmulberry silk biopolymers. Biopolymers. 2012;97(6):455–467.
- Yeo Y, Kohane DS. Polymers in the prevention of peritoneal adhesions. Eur J Pharm Biopharm. 2008;68(1):57–66.
- Yeo A, Rai B, Sju E, et al. The degradation profile of novel, bioresorbable PCL-TCP scaffolds: an in vitro and in vivo study. J Biomed Mater Res A. 2008;84:208–218.
- Sagnella A, Pistone A, Bonetti S, et al. Effect of different fabrication methods on the chemo-physical properties of silk fibroin films and on their interaction with neural cells. RSC Adv. 2016;6(11):9304–9314.
- Drummy LF, Phillips DM, Stone MO, et al. Thermally induced α-Helix to β-Sheet transition in regenerated silk fibers and films. Biomacromolecules. 2005;6(6):3328–3333.
- Qi Y, Wang H, Wei K, et al. A review of structure construction of silk fibroin biomaterials from single structures to multi-level structures. IJMS. 2017;18(3):237.
- Tsukada M, Gotoh Y, Nagura M, et al. Structural changes of silk fibroin membranes induced by immersion in methanol aqueous solutions. J Polym Sci B Polym Phys. 1994;32(5):961–968.
- Jackson EK. Intraperitoneal administration of adenosine inhibits formation of abdominal adhesions. Dis Colon Rectum. 2004;47(8):1390–1396.
- Wiseman DM, Gottlick-Iarkowski L, Kamp L. Effect of different barriers of oxidized regenerated cellulose (ORC) on cecal and sidewall adhesions in the presence and absence of bleeding. J Invest Surg. 1999;12:141–146.
- Kusunoki M, Ikeuchi H, Yanagi H, et al. Bioresorbable hyaluronate-carboxymethylcellulose membrane (seprafilm) in surgery for rectal carcinoma: a prospective randomized clinical trial. Surg Today. 2005;35(11):940–945.
- Hellebrekers BW, Trimbos-Kemper GC, van Blitterswijk CA, et al. Effects of five different barrier materials on postsurgical adhesion formation in the rat. Hum Reprod. 2000;15(6):1358–1363.
- Zeng J, Xu X, Chen X, et al. Biodegradable electrospun fibers for drug delivery. J Controlled Release. 2003;92(3):227–231.
- Verreck G, Chun I, Rosenblatt J, et al. Incorporation of drugs in an amorphous state into electrospun nanofibers composed of a water-insoluble, nonbiodegradable polymer. J Controlled Release. 2003;92(3):349–360.
- Strick MJ, Filan SL, Hile M, et al. Adhesion formation after flexor tendon repair: a histologic and biomechanical comparison of 2- and 4-strand repairs in a chicken model. J Hand Surg Am. 2004;29(1):15–21.
- Oh SH, Kim JK, Song KS, et al. Prevention of postsurgical tissue adhesion by anti-inflammatory drug-loaded pluronic mixtures with sol-gel transition behavior. J Biomed Mater Res A. 2005;72(3):306–316.
- Miller JA, Ferguson RL, Powers DL, et al. Efficacy of hyaluronic acid/nonsteroidal anti-inflammatory drug systems in preventing postsurgical tendon adhesions. J Biomed Mater Res. 1997;38(1):25–33.