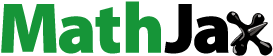
Abstract
The cytokine network of tumour microenvironment (TME) plays an important role in cancer growth and progression. The current work aims to provide a new strategy for cancer therapy based on the targeted regulation of cytokines in the TME. Here, heparin-coupled polyvinyl alcohol (PVA-H) microspheres have been developed as an adsorbent for selectively remove tumour-induced immunosuppressive cytokines, such as vascular endothelial growth factor (VEGF) and transforming growth factor-beta (TGF-β), but not tumour necrosis factor-alpha (TNF-α) which has an immune-stimulating effect and can inhibit tumour growth. The proliferation and apoptosis of breast cancer cells after perfusion were tested by cell viability assays, flow cytometry analysis and mRNA microarray assays. Results showed that the PVA-H microspheres efficiently absorbed the majority of VEGF (74.39%) and TGF-β (86.39%), but much less TNF-α (4.16%). The regulation of the cytokines had remarkable anti-proliferative and pro-apoptotic effects on breast cancer cells, which was further confirmed from the change of mRNA expression levels. Thus, targeting regulatory pathways within the TME by an affinity adsorbent that selectively depletes immunosuppressive cytokines is potentially a new and promising strategy for cancer therapy.
Introduction
The tumour microenvironment (TME) plays an essential role in the occurrence, development and metastasis of cancer [Citation1]. In the TME, plenty of cytokines account for tumour progression, recruitment of tumour-associated immune cells, and immunosuppressive effects. Therefore, regulation of these cytokines can reverse the immunosuppressive microenvironment and improve anti-tumour efficiency. These tumours are hypervascularized because of the overexpression of proangiogenic factors [Citation2], such as tumour-induced immunosuppressive cytokines (e.g. vascular endothelial growth factor (VEGF) and transforming growth factor-beta (TGF-β)), especially in breast cancer. VEGF is vital for the direct regulation of the cell survival and growth of breast cancer cell lines and blocking VEGF has direct and rapid antiangiogenic effects in the tumour [Citation3,Citation4]. TGF-β has a strongly pro-tumour effect at later stages of cancer [Citation5]. Nancy Dumont et al. [Citation5] had obtained the data relating to the cellular and molecular mechanisms by which TGF-β signalling can accelerate the natural history of tumour development. Whereas tumour necrosis factor-alpha (TNF-α) is one of the strongest anti-cancer factors that is frequently upregulated in various types of cancer, which can enhance an antitumor immune response in the TME. For clinical applications, TNF-α is safe and high efficient for the treatment of soft tissue sarcoma [Citation6]. In addition, other immunosuppressive cytokines (IL-4 and IL-10) and immune-stimulating cytokines (IL-1β and IL-6) can also regulate the proliferation and apoptosis of tumour cells [Citation7]. In response to TME, eliminating the excess tumour-promoting cytokines shown obvious advantages in overcoming tumour proliferation and hypervascularity [Citation8].
For instance, Christopher G Willett et al. [Citation9] used bevacizumab to cure rectal cancer patients and indicated that VEGF blockade had a direct and rapid antivascular effect in human tumours. Jianming Xu et al. [Citation2] developed a novel small-molecule inhibitor (Surufatinib) that targets proangiogenic factors, which could reduce tumour angiogenesis and enhance antitumor activity. However, the cost of these drugs treatment is high, and it also has clinically obvious pharmacodynamic limitations in respect of hypertension and proteinuria. Beyond conventional drug administration, hemoperfusion has been proved to be an effective and safe therapy to eliminate toxins in blood and is very successfully used in clinical. Yoshiki Yamamoto et al. [Citation10] reported extra-fine fibres for efficiently TGF-β removal and prolonged a longer survival time of the tumor-bearing rats through hemoperfusion. Therefore, it is of great clinical significance to develop a novel selectively adsorbent with higher adsorption capacity, aiming at simultaneous tumour-induced immunosuppressive cytokines (VEGF and TGF-β) cleansing, without a significant reduction of immune-stimulating cytokine (TNF-α) in a hemoperfusion system, to offer another effective technique for the regulation of TME in cancer treatment.
Polyvinyl alcohol (PVA) microspheres have been intensively investigated as an ideal carrier [Citation11], owing to their favourable mechanical properties, abundant macropore structure, and excellent biocompatibility, which are very important for hemoperfusion. Furthermore, a large number of hydroxyl groups on PVA can be easily functionalized by non-covalent and covalent chemical interactions. It is also widely reported that PVA beads represent a type of antifouling polymer, and have few adverse effects on blood components [Citation12]. Heparin is a highly sulphated linear polysaccharide belonging to the family of glycosaminoglycans and has been widely used as an anticoagulant agent. In the field of adsorbent-based hemoperfusion, heparin is always introduced to functionalize polymers in order to improve the blood compatibility of adsorbent, or used as a good ligand for the removal of certain toxins, including endotoxin and low-density lipoprotein (LDL) [Citation13]. However, the role of PVA-H in targeting regulation of TME using hemoperfusion column is not reported. To the best of our knowledge, VEGF contains some high-affinity binding sites for heparin, and therefore the heparin-functionalized adsorbent could be a good choice for the regulation of the TME through efficiently eliminating the excess VEGF [Citation14]. Moreover, heparin and TNF-α (pI = 5.6) molecules are negatively charged, while VEGF (pI = 8.5) and TGF-β (pI = 8.9) molecules are positively charged [Citation15]. Therefore, heparin would be expected to selectively bind with VEGF and TGF-β by electrostatic interaction, but for TNF-α, this binding ability should be weak.
In the present study, immobilized heparin onto PVA beads (PVA-H) were prepared, which was demonstrated that targeting regulation of cytokines in the TME by decreasing the levels of VEGF and TGF-β had anti-proliferative and pro-apoptotic effects on breast cancer cells (), offering a potential new strategy for breast cancer therapy.
Materials and methods
Immobilisation and characterisation of PVA and PVA-H microspheres
Polyvinyl acetate (PVA) microspheres were synthesized using a suspension polymerization method [Citation16] and the PVA-H microspheres were formed through the crosslinking between PVA beads and heparin (refer to Supplementary Information for details of synthetic strategy). Furthermore, the detailed characterization was provided in the Supplementary Information.
Adsorption experiments
For testing the adsorption performance of these PVA-H microspheres for VEGF, TGF-β, and TNF-α, human plasma was spiked with the recombinant human cytokine at a target concentration of 1000 pg/mL. Prior to use for adsorption experiments, the microspheres were allowed to swell fully in 0.9% saline solution. For brevity, details were provided in the Supplementary Information.
Regulation of cytokine network of TME by PVA-H microspheres
Cell culture
SHZ-88 rat breast cancer cells were obtained from the Cell Bank of Type Culture Collection of the Chinese Academy of Sciences, they cultured in complete RPMI 1640 media at 37 °C in an atmosphere of 5% CO2. Cell lines were subcultured for the subsequent experiment by adding 0.25% trypsin, 10% FBS, and fresh RPMI 1640 media. All of the cell culture materials were supplied by Biological Industries Israel Beit Haemek Ltd.
Cell viability assay
The cells were seeded into 96-well plates at a density of 3000 cells per well and cultured in their medium* (refer to Supplementary Information for details of the preparation of medium*) respectively. The cell viability was assayed with the CCK-8 Kit according to the manufacturer’s instructions. CCK-8 was detected at 0, 6, 12, 24, 48 and 72 h. Each experiment was done in sextuplicate.
Annexin V–FITC and PI assay
The cells were seeded into 6-well plates at a density of 9 × 104 cells per well and cultured in their medium* respectively. Cell apoptosis at 48 and 72 h were assessed by using the FITC Annexin V Apoptosis Detection Kit I (BD Pharmingen, USA) according to the manufacturer’s instructions, and the stained cells were analyzed by two-color flow cytometry.
RNA preparation and mRNA microarray experiments
Gene expression at 72 h was measured in mRNA microarray assays, which were performed by the Cnkingbio Biotechnology Corporation (Beijing, China). Total RNA was extracted from cells using the TRIzol reagent (Invitrogen, Carlsbad, Canada) and purified with an RNeasy Mini Kit (Qiagen, Hilden, Germany). RNA integrity and purity were investigated by absorption spectroscopy and electrophoresis. The hybrid chip was scanned using an Affymetrix Scanner 3000 7G laser confocal scanner (Affymetrix, USA), and then the bioinformatics analysis was performed.
Statistical analysis
All results are presented as mean ± SD, as noted in the text. Two-tailed non-paired t-test was used to compare the difference between two groups in GraphPad Prism (GraphPad Software Inc.), and statistical significance was displayed as “*” when p < .05, “**” when p < .01, “***” when p < .001, “****’ when p < .0001 and “ns” when p > .05.
Results and discussion
Characterisation
Scanning electron microscope (SEM) images () showed that both PVA and PVA-H possessed a well-formed spherical shape with a mean diameter of 100 μm and a highly porous structure. The results indicated that the functionalization of heparin by means of employing glutaraldehyde as a spacer arm did not have any significant impact on the structure of the microspheres, and clear macropores could still be seen on their rough external surfaces.
Figure 1. Characterization of PVA and PVA-H microspheres. (a) The shape (300×) and porous structure (10,000×) micrographs of PVA and PVA-H microspheres by SEM. (b) Bright field, fluorescent and merge photographs of PVA and PVA-H/FITC. For each panel, images from left to right show bright field, fluorescent photographs, and merge of two images, respectively. (c) The XPS wide spectrum of PVA-H and the fitted peak analysis of the XPS for N 1 s and S 2p of PVA-H.
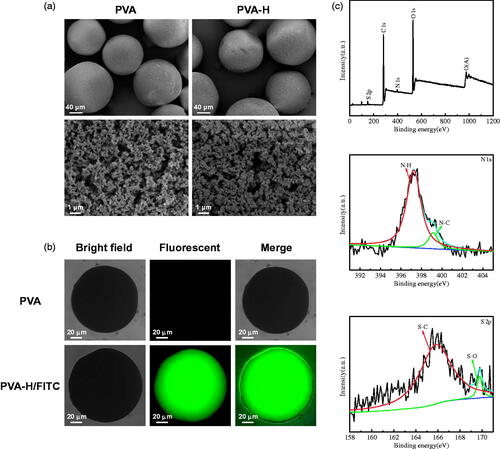
A fluorescence labelling strategy was also used to provide more direct evidence. These special PVA-H microspheres were prepared using fluorescein isothiocyanate (FITC)-labeled heparin (ThermoFisher, #H7482). shows the images of FITC-heparin immobilised PVA compared with unmodified PVA beads, an obvious fluorescence signal was detected on the PVA-H, while no signal was detected for the original PVA beads. Therefore, the presence of heparin on the PVA-H microspheres could be confirmed [Citation17].
The nature of the functional groups of the PVA and PVA-H microspheres was characterized by FTIR spectroscopy, as shown in Figure S1. After the functionalization of heparin, the PVA peak at 1124 cm−1 was shifted to 1091 cm−1, probably due to the overlapping of the heparin peak at 1020 cm−1 (sulphonic acid group). This indicates that heparin was present in the PVA-H microspheres [Citation18]. X-ray photoelectron spectroscopy (XPS) analyses of the obtained materials were also conducted to characterize the heparin component (SO32−) on the surface of the PVA-H microspheres. As shown in and Table S1, the surface sulphur content of the PVA-H microspheres was about 0.07 wt.%, and the sulphur element could be detected at 163.7 eV. For comparison, PVA microspheres had no sulphur elements as is shown in Figure S2. These results demonstrated that heparin was successfully immobilized on the PVA microspheres.
Assessment of adsorption characteristics
The PVA-H microspheres were prepared using different amounts of heparin. As shown in Figure S3, the optimal dosage of heparin was identified as 60 mg in order to achieve the best adsorption capacity of the PVA-H microspheres for VEGF (70.37%, 31.31 ng/g). Next, the adsorption characteristics of PVA-H for VEGF, TGF-β, and TNF-α in human plasma were compared, as shown in , Figure S4 and Table S2. The results of time-dependent adsorption kinetics () demonstrated that the uptake of VEGF by PVA-H was quite rapid at the very beginning and reached equilibrium within 90 min. The final VEGF adsorption rate of PVA-H reached 71.23%, with an adsorption capacity measured as 32.06 ng/g. In contrast, the comparable VEGF adsorption rate of PVA alone was only about 5%, which was clearly much lower than that of the PVA-H microspheres. Furthermore, the kinetic data were analyzed using two extensively used kinetic models (pseudo-first-order and pseudo-second-order models). The nonlinear form of the two models is expressed as equations:
(1)
(1)
(2)
(2)
where, k1 (min−1) is the first-order equilibrium rate constant, k2 (g/ng min) is the second-order equilibrium rate constant, Qe and Qt are the adsorption quantity (ng/g) at equilibrium and at time t, respectively. The relevant parameters (Qe, exp, Qe, calc, k), the correlation coefficients (R2), and non-linear fitting are shown in Table S2 and . It could be seen that R12 was higher than R22 value, which was manifested that the physical adsorption played an important role in the adsorption of VEGF by PVA-H (Table S2 and ) [Citation19]. In addition, as shown in , PVA-H also had an excellent adsorption capacity for TGF-β (86.39%, 30.28 ng/g) compared with PVA (62.85%, 20.58 ng/g), and was able to reach adsorption equilibrium quickly. From the data of TGF-β onto the two adsorbents (Table S2), pseudo-second-order had a higher R22, indicating the adsorption of TGF-β using PVA-H was dominated by the chemical adsorption [Citation20]. Even more noteworthy is the fact that over time, PVA-H barely adsorbed any TNF-α (less than 4.5%), a representative of the immune-stimulating cytokines, which is shown in . It is precisely because the heparin is a linear sulphated polysaccharide, molecules of which are negatively charged, which is similar to TNF-α (pI = 5.6). In contrast, VEGF (pI = 8.5) and TGF-β (pI = 8.9) molecules are positively charged. Therefore, PVA-H can selectively bind with VEGF and TGF-β but not TNF-α through electrostatic interactions between the sulphonic acid groups of heparin and the basic amino acids of cytokines. Taken together, the adsorption characteristics of PVA-H microspheres for cytokines had a certain selectivity, which was beneficial for the following regulation of the TME by removing immunosuppressive but not immunostimulatory cytokines.
Figure 2. The adsorption characteristics of PVA-H for VEGF, TGF-β and TNF-α in human plasma. (a) Non-linear fitting for the adsorption kinetic data of VEGF onto the two adsorbents in human plasma (T = 37 °C, CVEGF = 1335.21 ± 3.92 pg/mL). (b) Effects of temperature on adsorption of VEGF (t = 2 h, CVEGF = 1152.32 ± 4.23 pg/mL). (c) Non-linear fitting for the adsorption kinetic data of TGF-β onto the two adsorbents in human plasma (T = 37 °C, CTGF-β = 1048 ± 1.24 pg/mL). (d) Effects of temperature on adsorption of TGF-β (t = 2 h, CTGF-β = 1121 ± 2.34 pg/mL). (e) Non-linear fitting for the adsorption kinetic data of TNF-α onto the two adsorbents in human plasma (T = 37 °C, CTNF-α = 1069 ± 3.24 pg/mL). (f) Effects of temperature on adsorption of TNF-α (t = 2 h, CTNF-α = 968 ± 4.25 pg/mL). The plasma to adsorbent ratio was 20 and all values are expressed as mean ± SD (n = 3). ns: not significant, **p < .01, ***p < .001, ****p < .0001.
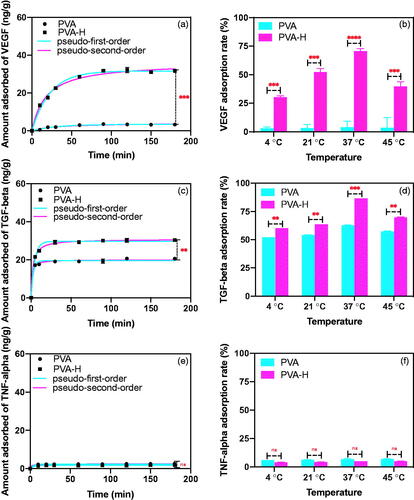
The effect of temperature on cytokine adsorption is shown in . The degree of cytokines adsorption on PVA-H significantly increased with the increase of temperature, probably due to the conformational transition leading to the increased surface hydrophobicity of cytokines at higher temperatures [Citation19,Citation21]. However, adsorption is an exothermic process, such that after a certain temperature was reached, the binding affinity decreased with increasing temperature.
Regulation of cytokine network of TME by PVA-H microspheres
Before this experiment, we had established that cultures of SHZ-88 cells were minimally affected by exposure to a cell culture medium that had been perfused through the PVA-H microspheres (Figure S6). Experiments to determine the effects of PVA-H microspheres on the regulation of the TME could then be performed (). During the attachment of breast cancer cells for 12 h, abundant VEGF and TGF-β were produced and secreted into the culture medium, whereas the amount of TNF-α was negligible (less than 5 pg/mL). Meanwhile, the expression of IL-1β, IL-4, IL-6, and IL-10 in the cell culture supernatants were measured using a premixed multi-analyte kit (R&D Systems), whereas the concentrations were too low to detect (data not shown). In addition, VEGF and TGF-β are generally regarded as two of the most representative immunosuppressive cytokines during the growth and metastasis of tumor, and their overexpression makes an inactive effect for the cure of cancer, while TNF-α is a typical proinflammatory cytokine. Therefore, VEGF, TGF-β, and TNF-α were chosen to assess the perfusion capacity.
Figure 3. Targeting regulation of the TME in SHZ-88 cells by PVA-H microspheres. (a) Schematic illustration of PVA-H microspheres dynamic targeting experiment. (b) Adsorption rate of different cytokines in the culture supernatants after perfusion through PVA and PVA-H microspheres (T = 37 °C, CVEGF = 490.83 ± 5.32 pg/mL, CTGF-β = 345.92 ± 6.32 pg/mL, CTNF-α = 4.12 ± 1.24 pg/mL the plasma to adsorbent ratio was 20). (c) CCK-8 experiments showed that perfusion through PVA-H microspheres attenuated the proliferative capacity of SHZ-88 cells. All values are expressed as mean ± SD (n = 6). ns: not significant, **p < .01, ***p < .001, ****p < .0001.
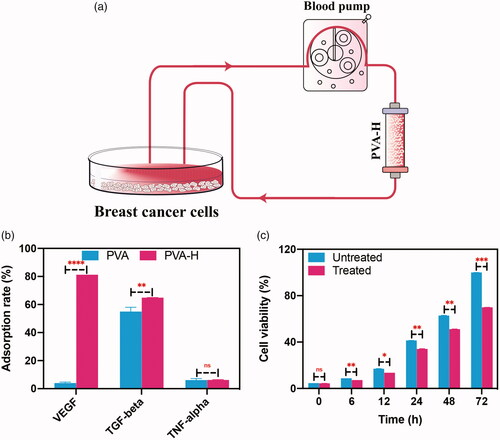
After perfusing these supernatants through the PVA-H microsphere column, as shown in , the concentration of VEGF therein sharply decreased from 490 to 92 pg/mL, and the concentration of TGF-β also decreased significantly from 345 to 121 pg/mL. Then, HUVECs were cultured in the conditioned medium with or without PVA-H perfusion for tube formation assay. The results demonstrated the perfusion using PVA-H microspheres can restrain the tube formation (Figure S7). Next, the effect of perfusion on the viability of SHZ-88 cells were assessed using the Cell Count Kit-8 (CCK-8) assay. As shown in , the cell viability of the treated group was lower than that of the control group at all different time points (0, 6, 12, 24, 48 and 72 h). This model of regulation in the TME indicates that the cell proliferation of SHZ-88 was dramatically inhibited when these cytokines were removed. Moreover, Figure S8 showed the proliferation of rat breast cancer cells was promoted by adding VEGF and TGF-β back into the treated medium at 24 and 48 h (add back group), which demonstrated that the VEGF and TGF-β played a vital role in the proliferation of SHZ-88 cells.
Flow cytometry analysis with Annexin V and propidium iodide was also performed to measure apoptosis of the SHZ-88 breast cancer cells. In , the flow histogram quadrants Q1, Q2, Q3, and Q4 indicate necrotic cells, late-stage apoptotic cells, early-stage apoptotic cells, and normal cells, respectively. The proportions of apoptotic cells in the treated group at 48 and 72 h reached as much as 47.69 and 44.6%, respectively. For the untreated group, the proportions of apoptotic cells at 48 and 72 h were only 15.17 and 22.74%, respectively. Altogether, it is concluded that the cell apoptosis of SHZ-88 was promoted in this model of regulating the TME by depleting anti-inflammatory cytokines.
Figure 4. Annexin V–FITC and PI assay. Effect of perfusion through PVA-H microspheres on cell apoptosis. All values are expressed as mean ± SD (n = 3).
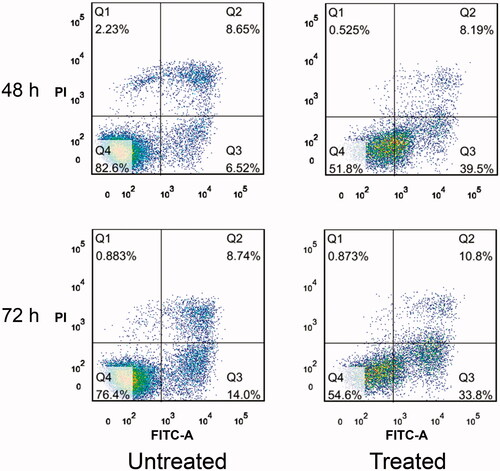
Therefore, these results demonstrated that the regulation of the TME using adsorbent-based hemoperfusion in this model had marked anti-proliferative and pro-apoptotic effects on breast cancer cells due to the removal of excess immunosuppressive cytokines including VEGF and TGF-β. We thus envisioned our hemoperfusion adsorbent as an ideal candidate for cancer treatment.
In order to evaluate the effect of perfusion at the level of gene expression of SHZ-88 cells, mRNA microarray assays were performed after 72 h exposure. As shown in Figure S9, we identified 954 significantly differentially expressed genes by setting the absolute fold change threshold to >1.2 and the p-value at <.05. It can be seen from the cluster gram that the samples from the two groups are clearly separated, which indicates a robust response to PVA-H perfusion treatment. Among these 954 differentially expressed genes, 420 genes were downregulated and 534 genes were upregulated. It is worth noting that in Figure S10, we had further chosen to focus on genes involved in proliferation and apoptosis. , Tables S5 and S6 showed the genes related to positive and negative regulation of proliferative and apoptotic. Genes positively regulating proliferating were down-regulated (light blue), while those negatively regulating proliferation were up-regulated (red). Genes positively regulating apoptosis were up-regulated (red), while those negatively regulating apoptosis were down-regulated (light blue). Thus, as for the functionally significantly related genes involved in the proliferative and apoptotic functions (), on the basis of related research, not only the down-regulated genes, such as gene Timp3, Ppargc1a, Lep and Nck2, but also the up-regulated genes, such as gene Blm, Rag1, Lifr and Ptk2b, would promote apoptosis of breast cancer cells [Citation22–31]. More importantly, both the down-regulated genes, such as gene Mmp12, Cxcl1, Lep and Wnt10b, and the up-regulated genes, such as gene Gpc3, Miip, Ovol1 and Fhl1, would inhibit the proliferation of breast cancer cells [Citation27,Citation28,Citation32–41]. Taken together, these results imply that most genes involved in proliferation and apoptosis were changed in such a manner that they exhibited a positive tendency to inhibit proliferation and promote apoptosis of the breast cancer cells after treatment by the perfusion through PVA-H microspheres.
Figure 5. Mechanism of the anti-proliferative and pro-apoptotic effects on breast cancer cells. SHZ-88 cells were cultured for 12 h and then exposed to supernatants treated or not treated by perfusion through PVA-H microsphere columns. (n = 4). (a) Genes involved in positive and negative regulation from the genes involved in proliferation and apoptosis. (Left: Genes associated with positive and negative regulation of cell proliferation; Right: Genes involved in positive and negative regulation of apoptosis). (b) Functionally significantly related genes involved in the proliferative and apoptotic functions (Left: proliferation-related genes; Right: apoptosis-related genes).
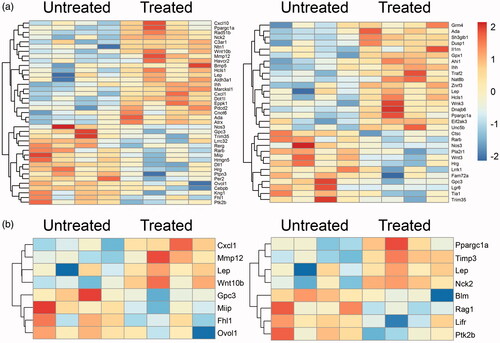
To gain a better understanding of how the perfusion through PVA-H microsphere columns affects the tumour, Kyoto Encyclopaedia of Genes and Genomes (KEGG) pathway enrichment analysis was performed as shown in . Notably, the TGF-beta signalling pathway was clearly enriched in those pathways that were down-regulated after treatment, which was consistent with the previous results. Next, gene ontology (GO) enrichment analysis showed that the antitumor activities had been up-regulated, such as the negative regulation of the vascular endothelial growth factor signalling pathway, positive regulation of cytokine production involved in inflammatory response, regulation of macrophage chemotaxis, regulation of chromatin silencing, long-chain fatty acid import, and others (). In contrast, the pro-tumour activities had been down-regulated, for instance, the inactivation of MAPK activity, negative regulation of Ras protein signal transduction, DNA integration, fructose transport, and others. KEGG pathway and GO enrichment analysis were combined to demonstrate that both VEGF and TGF-β were down-regulated at the gene level. It was concluded that this study provided a facile strategy to fabricate a selectively affinity adsorbent by hemoperfusion for cancer therapy.
Blood compatibility
Haemolysis experiments, blood routine tests, platelet adhesion and coagulation assays were conducted to evaluate the blood compatibility of the PVA-H microspheres (refer to Supplementary Information). For the haemolysis assay, the mean optical density (OD) values of the negative and positive controls were 0.0191 ± 0.0051 and 0.8915 ± 0.0047, respectively, with both these values conforming to the ISO 19003-4:2002(E) standard. As shown in and Table S3, the haemolysis ratio for PVA-H microspheres was about 3.22 ± 0.24% (<5%), indicating that the functionalization of heparin on the PVA microspheres exhibited little risk of facilitating haemolysis during application. The influence of PVA-H microspheres on blood components after 60 min of direct contact is also shown in Table S3. It was found that PVA-H microspheres had negligible negative effects on the majority of blood cells including white blood cells (WBC) and red blood cells (RBC), as compared with the normal group. A little platelet (PLT) adsorption was observed for PVA and PVA-H microspheres, which was still in an acceptable range for clinical uses (<20%) (). Therefore, these results demonstrated that PVA-H microspheres possessed an excellent blood compatibility profile and had a high potential to be applied in hemoperfusion for cancer treatment.
Conclusions
In summary, heparin was successfully immobilized onto PVA microspheres as an adsorbent for selectively removing tumour-induced immunosuppression cytokines (VEGF and TGF-β) but to retain the anti-tumour cytokines (TNF-α) in the TME by perfusion. The proliferation of the rat breast cancer cells was inhibited, and apoptosis was promoted. mRNA microarray assays indicated that differentially expressed genes in treated cells were involved in inhibiting proliferation and promoting apoptosis of the breast cancer cells. Furthermore, KEGG pathway and GO enrichment analysis were combined to demonstrate that both VEGF and TGF-beta were down-regulated at the gene level. Besides, the PVA-H microspheres had excellent blood compatibility (a negligible haemolytic activity and platelet adhesion) for hemoperfusion.
Compared to antibody-based drugs, hemoperfusion is a safer and more economical therapy, which has been widely used in the treatment of acute drug poisoning, liver failure and other difficult miscellaneous diseases. Therefore, hemoperfusion with PVA-H microspheres targeting the regulation of TME may be a useful and cost-effective approach for cancer treatment.
Supplementary data related to this article can be found on the publisher’s website.
Supplemental Material
Download MS Word (13.6 MB)Disclosure statement
No potential conflict of interest was reported by the author(s).
Data availability statement
The authors confirm that the data supporting the findings of this study are available within the article and its supplementary materials.
Additional information
Funding
References
- Hoshino A, Costa-Silva B, Shen T-L, et al. Tumour exosome integrins determine organotropic metastasis. Nature. 2015;527(7578):329–335.
- Xu J, Shen L, Zhou Z, et al. Surufatinib in advanced extrapancreatic neuroendocrine tumours (SANET-ep): a randomised, double-blind. Placebo-Controlled, Phase 3 Study, the Lancet Oncology. 2020;21(11):1500–1512.
- Valtola R, Salven P, Heikkilä P, et al. VEGFR-3 and its ligand VEGF-C are associated with angiogenesis in breast cancer. Am J Pathol. 1999;154(5):1381–1390.
- Weigand M, Hantel P, Kreienberg R, et al. Autocrine vascular endothelial growth factor signalling in breast cancer. Evidence from cell lines and primary breast cancer cultures in vitro. Angiogenesis. 2005;8(3):197–204.
- Dumont N, Arteaga CL. Transforming growth factor-beta and breast cancer: tumor promoting effects of transforming growth factor-beta. Breast Cancer Res. 2000;2(2):125–132.
- Victor F, Gottlieb A. TNF-alpha and apoptosis: implications for the pathogenesis and treatment of psoriasis. J Drugs Dermatol. 2002;1(3):264–275.
- Sheu B-C, Chang W-C, Cheng C-Y, et al. Cytokine regulation networks in the cancer microenvironment. Front Biosci. 2008;13(13):6255–6268.
- Ferrara N, Hillan KJ, Gerber H-P, et al. Discovery and development of bevacizumab, an anti-VEGF antibody for treating cancer. Nat Rev Drug Discov. 2004;3(5):391–400.
- Willett CG, Boucher Y, Tomaso ED, et al. Direct evidence that the VEGF-specific antibody bevacizumab has antivascular effects in human rectal cancer. Nat Med. 2004;10(2):145–147.
- Yamamoto Y, Ueda Y, Itoh T, et al. A novel immunotherapeutic modality with direct hemoperfusion targeting transforming growth factor-beta prolongs the survival of tumor-bearing rats. Oncol Rep. 2006;16(6):1277–1284.
- Yu YT, Zhu H, Wang S. Amphiphilic polyvinyl alcohol adsorbent for the removal of low-density lipoprotein, artificial cells. Artif Cells Nanomed Biotechnol. 2015;43(2):117–123.
- Wang W, Xie H, Sun L, et al. Macroporous poly(vinyl alcohol) microspheres bearing phosphate groups as a new adsorbent for low-density lipoprotein apheresis . Biomed Mater. 2009;4(6):065007.
- Ma K-W, Ma L, Cai S-X, et al. Preparation of heparin-immobilized PVA and its adsorption for low-density lipoprotein from hyperlipemia plasma. J Mater Sci Mater Med. 2008;19(10):3255–3261.
- Zhao W, McCallum SA, Xiao Z, et al. Binding affinities of vascular endothelial growth factor (VEGF) for heparin-derived oligosaccharides. Biosci Rep. 2012;32(1):71–81.
- Impellitteri NA, Toepke MW, Levengood SKL, et al. Specific VEGF sequestering and release using peptide-functionalized hydrogel microspheres. Biomaterials. 2012;33(12):3475–3484.
- Chen J, Han W, Su R, et al. Non-ionic macroporous polystyrene adsorbents for removal of serum toxins in liver failure by hemoperfusion. Artif Cells Nanomed Biotechnol. 2017;45(1):174–183.
- Zong W, Chen J, Han W, et al. Preparation of PVA/amino multi-walled carbon nanotubes nanocomposite microspheres for endotoxin adsorption. Artif Cells Nanomed Biotechnol. 2018;46(1):185–191.
- Wei H, Han L, Tang Y, et al. Highly flexible heparin-modified chitosan/graphene oxide hybrid hydrogel as a super bilirubin adsorbent with excellent hemocompatibility. J Mater Chem B. 2015;3(8):1646–1654.
- Cheng G, Chai Y, Chen J, et al. Polystyrene-divinylbenzene based nano-CaCO3 composites for the efficient removal of human tumor necrosis factor-α. Chem Commun. 2017;53(55):7744–7747.
- Li Q, Zhao W, Guo H, et al. Metal-organic framework traps with record-high bilirubin removal capacity for hemoperfusion therapy. ACS Appl Mater Interfaces. 2020;12(23):25546–25556.
- Song M, Winchester J, Albright RL, et al. Cytokine removal with a novel adsorbent polymer. Blood Purif. 2004;22(5):428–434.
- Johnson RW, Finger EC, Olcina MM, et al. Induction of LIFR confers a dormancy phenotype in breast cancer cells disseminated to the bone marrow. Nat Cell Biol. 2016;18(10):1078–1089.
- Coffelt SB, Kersten K, Doornebal CW, et al. IL-17-producing γδ T cells and neutrophils conspire to promote breast cancer metastasis. Nature. 2015;522(7556):345–348.
- Mombaerts P, Iacomini J, Johnson RS, et al. RAG-1-deficient mice have no mature B and T lymphocytes. Cell. 1992;68(5):869–877.
- Sokolenko AP, Iyevleva AG, Preobrazhenskaya EV, et al. High prevalence and breast cancer predisposing role of the BLM c.1642 C > T (Q548X) mutation in Russia. Int J Cancer. 2012;130(12):2867–2873.
- Labelle-Côté M, Dusseault J, Ismaïl S, et al. Nck2 promotes human melanoma cell proliferation, migration and invasion in vitro and primary melanoma-derived tumor growth in vivo. BMC Cancer. 2011;11(1):443.
- Cleveland RJ, Gammon MD, Long C-M, et al. Common genetic variations in the LEP and LEPR genes, obesity and breast cancer incidence and survival. Breast Cancer Res Treat. 2010;120(3):745–752.
- Gonzalez RR, Cherfils S, Escobar M, et al. Leptin signaling promotes the growth of mammary tumors and increases the expression of vascular endothelial growth factor (VEGF) and its receptor type two (VEGF-R2). J Biol Chem. 2006;281(36):26320–26328.
- Uría JA, Ferrando AA, Velasco G, et al. Structure and expression in breast tumors of human TIMP-3, a new member of the metalloproteinase inhibitor family. Cancer Res. 1994;54(8):2091–2094.
- Burwinkel B, Schmutzhard J, Tchatchou S, et al. Associations of genetic variants in the estrogen receptor coactivators PPARGC1A, PPARGC1B and EP300 with familial breast cancer. Carcinogenesis. 2006;27(11):2201–2208.
- Beck TN, Nicolas E, Kopp MC, et al. Adaptors for disorders of the brain? The cancer signaling proteins NEDD9, CASS4, and PTK2B in Alzheimer’s disease. Oncoscience. 2014;1(7):486–503.
- Hughes S, Agbaje O, Bowen RL, et al. Matrix metalloproteinase single-nucleotide polymorphisms and haplotypes predict breast cancer progression. Clin Cancer Res. 2007;13(22):6673–6680.
- Acharyya S, Oskarsson T, Vanharanta S, et al. A CXCL1 paracrine network links cancer chemoresistance and metastasis. Cell. 2012;150(1):165–178.
- Brennan KR, Brown AMC. Wnt proteins in mammary development and cancer. J Mammary Gland Biol Neoplasia. 2004;9(2):119–131.
- Bui TD, Rankin J, Smith K, et al. A novel human Wnt gene, WNT10B, maps to 12q13 and is expressed in human breast carcinomas. Oncogene. 1997;14(10):1249–1253.
- Hamamoto R, Silva FP, Tsuge M, et al. Enhanced SMYD3 expression is essential for the growth of breast cancer cells. Cancer Sci. 2006;97(2):113–118.
- Xiang Y-Y, Ladeda V, Filmus J. Glypican-3 expression is silenced in human breast cancer. Oncogene. 2001;20(50):7408–7412.
- Colander D, Holmes J. Gender and graduate economics education in the US. Feminist Econ. 2007;13(2):93–116.
- Dong C, Wu Y, Wang Y, et al. Interaction with Suv39H1 is critical for Snail-mediated E-cadherin repression in breast cancer. Oncogene. 2013;32(11):1351–1362.
- Ding L, Niu C, Zheng Y, et al. FHL1 interacts with oestrogen receptors and regulates breast cancer cell growth. J Cell Mol Med. 2011;15(1):72–85.
- Li X, Jia Z, Shen Y, et al. Coordinate suppression of Sdpr and Fhl1 expression in tumors of the breast, kidney, and prostate. Cancer Sci. 2008;99(7):1326–1333.