Abstract
Melanoma is a deadly skin cancer. Surgery is effective for early stages but there may be remnant cells. Treatments of later stages are associated with severe side effects. Moreover, a dangerous type of melanoma cannot be detected early enough for surgery. There is an urgent need for treatment with less severe side effects. We use a novel system of artificial cell polymer–lipid membrane nanocarrier containing a biomolecular nano-system of enzyme–oxygen biotherapeutic. In this report we show (1) its effectiveness and mechanisms in inhibiting the growth of melanoma in a 3D culture collagen medium that is more similar to that in the animal. (2) This allows us to design and carry out animal studies to successfully show that this can inhibit the growth of melanoma in an animal model. This includes following the tumour sizes and body weights every 2 days for 30 days followed by histology of the sites of injection and vital organs. We also analyze the action of the different components of the nanocarrier–nano-biotherapeutic complex. In conclusion, the results show the safety and clinical feasibility of this approach in the animal model and encourages further study towards clinical use.
Introduction
Melanoma is a deadly skin cancer. The incidence of melanoma has increased rapidly in the recent years [Citation1,Citation2]. Much progress has been made in treatment strategies, like immunotherapy, chemotherapy and others [Citation3–5]. These are more suitable for advance stages since they can be associated with severe side effects [Citation5,Citation6]. Surgery is effective for early stages [Citation7] but there may be remnant cells. A dangerous type of melanoma cannot be detected early [Citation3,Citation8]. Treatment with less severe side effects is needed for those conditions that may not need the more severe immunotherapy and chemotherapy
There is renewed interest in metabolic approach that has less adverse effect [Citation9]. Melanoma needs tyrosine [Citation10,Citation11]. Extensive study by Meadows’ group shows that dietary depletion of tyrosine/phenylalanine suppresses the growth of melanoma [Citation10]. However, it is difficult to maintain the dietary depletion in human. We, therefore, look into another way to deplete tyrosine. Our soluble nano-biotherapeutic poly-[haemoglobin–tyrosinase] (PH-TYR), can lower the systemic tyrosine level, but it is removed quickly from the circulation and we need a carrier to increase the time of its function [Citation12]. We use one of the artificial cell nano-carriers [Citation6,Citation12–15] to prepare a novel biodegradable polymer–lipid nanocarrier containing this nano-biotherapeutic [Citation16,Citation17]. We have established the preparative procedure, characterization and analysis in 2D culture study [Citation16,Citation17]. We now report our 3D culture study followed by animal study that shows its effectiveness in inhibiting the growth of melanoma in an animal model ().
Figure 1. (a) Preparation: first by crosslinking haemoglobin and tyrosinase into a soluble nanobiotherapeutic complex of poly-[haemoglobin-tyrosinase] that functions as an oxygen therapeutic with enzyme activity. This is then bioencapsulated into a polymer–lipid artificial cell nanocarrier. (b) This is place in a 2D culture with B16F10 melanoma cell to study its effect. This is more suitable for sample collections to analyze the mechanism of action on the tumour cells. (c) 3D culture is more comparable to the in vivo condition that would allow us to analyze the in vivo action and dosage needed for animal study. (d) Local injection to C57BL/6 mice to study the inhibition of B16F10 melanoma growth. (e) Sites of action of injected nanoencapsulated PolyHb-tyrosinase.
![Figure 1. (a) Preparation: first by crosslinking haemoglobin and tyrosinase into a soluble nanobiotherapeutic complex of poly-[haemoglobin-tyrosinase] that functions as an oxygen therapeutic with enzyme activity. This is then bioencapsulated into a polymer–lipid artificial cell nanocarrier. (b) This is place in a 2D culture with B16F10 melanoma cell to study its effect. This is more suitable for sample collections to analyze the mechanism of action on the tumour cells. (c) 3D culture is more comparable to the in vivo condition that would allow us to analyze the in vivo action and dosage needed for animal study. (d) Local injection to C57BL/6 mice to study the inhibition of B16F10 melanoma growth. (e) Sites of action of injected nanoencapsulated PolyHb-tyrosinase.](/cms/asset/cd1c184c-4a7e-4fed-a89e-6f3c468bb044/ianb_a_1918134_f0001_c.jpg)
Methods and materials
Preparation of polyhaemoglobin and polyhaemoglobin–tyrosinase
This has been described in our earlier publication [Citation16,Citation17]. Briefly, stroma-free haemoglobin (SFHb) with a concentration of 7 g/dL Hb with or without tyrosinase was dissolved in a 0.1 mol/l sodium phosphate buffer (pH = 7.4). Lysine was added at a molar ratio of 7:1 lysine/haemoglobin. Glutaraldehyde as crosslinker was added at a molar ratio of 16:1 glutaraldehyde/haemoglobin. The process of crosslink continued for 24 h. After that, lysine was added again to stop the crosslink reaction at a molar ratio of 200:1 lysine/haemoglobin. The sample was dialyzed overnight with a dialysis membrane (molecular weight cut off = 12–14 kDa) and then passed through sterile 0.45 μm syringe filters to remove impurities. All operations were conducted at 4 °C and under nitrogen in order to prevent the formation of methaemoglobin and the degradation of enzyme.
Preparation of polylactide–lipid membrane nanocarriers containing PH-TYR
This was described in our earlier publication [Citation16,Citation17]. Briefly, 50 mg dl-PLA and 25 mg hydrogenated soybean phosphatidylcholine were dissolved in 4 ml acetone and 2 ml ethanol by sonication for 30 min. The resulting organic phase was added dropwise into 5 ml 7 g/dl of PH-TYR solution under moderate magnetic stirring. Then the suspension was stirred continually for 30 min. The organic solvent was removed using rotary evaporator under vacuum for 1 h. Then the nano-capsules were separated by centrifugation at 20,000 rpm for 20 min to separate the supernatant and sediment. Then the suspension was concentrated with filtration.
Characterizations
The nanoencapsulation efficiency of PH-TYR into the nanocarrier was analyzed. Transmission electron microscopy (TEM) was used to analyze the characteristics and diameter range of the nanocapsules. We followed the distribution by engineering fluorescence labelled Nanocarrier-PH-TYR, and the entry of nanocarrier-PH-TYR into the melanoma cells for the 72 h of observation by confocal imagining. In confocal imagining, the nanocarrier-PH-TYR were stained by coumarin-6 (green dye) and the nucleus of the melanoma cells were stained with H33342 (blue dye). We followed the entry of nanocarrier-PH-TYR into the melanoma cells for the 72 h of observation. MTT test was used to study the viability of melanoma cells. We studied the invasive migration of the B16F10 tumour cells using the scratch test [Citation17]. Crystal violet staining was used to analyze the colony counts. Apoptosis analysis by flow cytometry was used to analyze the apoptosis of melanoma cells treated with the Nanocarrier-PH-TYR. We also tracked ROS generation with CellROX reagent. Cell cycle was studied using expressions of FAK and α4 integrin and the expression of cyclin D1, cyclin D3and CDK2.
Tumour cells and 3D culture conditions
B16F10 murine melanoma cells (American Type Culture Collection, ATCC®, #CRL-6475) were cultured in standard Dulbecco’s modified Eagle’s medium (DMEM) supplemented with 10% foetal bovine serum at 37 °C and 5% CO2 humidified atmosphere. Cells were washed with 0.25% trypsin/EDTA for 1 min to be detached from the dish. Then cell suspensions were collected in a sterile 15 ml tube and centrifuged at 1500 rpm for 5 min. Cells were counted by trypan blue exclusion.
In vivo effect in C57BL6 mice
This study was approved by the McGill University animal research ethics committee (McGill University Animal Use Protocol – Research no. 3816). The in vivo effect of polylactide-nanocarriers containing PH-TYR on B16F10 murine melanoma was studied in C57BL6 mice. C57BL6 mice were purchased from Charles River Canada. Mice were kept with a 12 h light/dark schedule and fed conventional food and water ad lib. All animals were housed and cared for according to the animal care regulations of McGill University. All mice were acclimatized for at least 7 days prior to use in the experiment.
B16F10 murine melanoma cell suspensions with different test solutions were used with n = 6 mice for each of the following groups each compared to a control group of saline solution.
Polylactide–lipid membrane nanocarriers containing PH-TYR.
Polylactide–lipid nanocarriers with no contents.
Free PH-TYR in solution.
Polylactide–lipid nanocarriers containing only poly-haemoglobin.
Each suspension was inoculated subcutaneously into the lateral flank of C57BL6 mice. Two B16F10 cell loads/test suspension volume were tested. (1) Study using cell load of 0.5 × 105 B16F10 melanoma cells/0.15 ml test suspension and (2) study using cell load of 1.0 × 105 B16F10 melanoma cells/0.15 ml test suspension.
The primary tumour size and the body weight were followed every 2 days. The tumour volume was calculated as V= (A*B2)/2. where V is the volume (mm3); A is the longest diameter (mm); B is the shortest diameter (mm). At the endpoint, the tumour tissues were collected for histological analysis.
Analysis of melanoma sites
Tissues of melanoma sites were collected and sent to the university histology division. Tissues sections were stained with haematoxylin and eosin. The expression profiles of Melan-A were analyzed by immunohistochemistry (IHC) methods. The histological analysis was analyzed independently by the animal pathologist of the university histology division.
Data analysis
Statistical analysis was performed using Student’s t-test or one-way ANOVA within analysis of variance and was considered to be significant at p < 0.05.
Result and discussion
Characterization and 2D culture
We have reported the following in earlier study [Citation16,Citation17]. The nanoencapsulation efficiency of PH-TYR into the nanocarrier is 75.4%. TEM image shows spherical and freely dispersed nanocarrier–PH-TYR with a size distribution ranging from 88 to 267 nm, and 64% are in the range of 120 to 200 nm. We have prepared these using both PEG-dl-PLA-lipid and dl-PLA-lipid membranes and use dl-PLA-lipid for the 2D study. We follow the distribution by engineering fluorescence labelled nanocarrier–PH-TYR. In confocal imagining, the nanocarrier–PH-TYR are stained by coumarin-6 (green dye) and the nucleus of the melanoma cells are stained with H33342 (blue dye). Nanocarrier–PH-TYR enters the melanoma cells within 1 h and remains inside the cytoplasm for the 72 h of observation. Within 24 h, the tyrosine level significantly decreases to 1% compare to that in the untreated control. MTT test shows that nanocarrier–PH-TYR significantly decreases the viability of melanoma cells in their proliferation phase at 24 h, and more than 80% in 48 h. We then study the invasive migration of the B16F10 tumour cells. In the control group, B16F10 tumour cells migrated into the scratch area and after 48 h, the scratch area is decreased to 1/3 of that at 0 h time. Nanocarrier–PH-TYR impaired the migration ability with lack of decrease in the scratched area. Crystal Violet staining shows that nanocarrier–PH-TYR significantly decreases the colony counts. Apoptosis analysis by flow cytometry shows apoptosis of melanoma cells treated with the nanocarrier–PH-TYR. Nanocarrier–PH-TYR impairs cell cycle resulting in 25% of G0/G1 arrest. Tracking ROS generation with CellROX reagent shows that intracellular ROS generation significantly increases after nanocarrier–PH-TYR treatment for 24 h and 48 h when compared with the untreated control. Nanocarrier–PH-TYR inhibits expressions of FAK and α4 integrin. It also downregulates the expression of cyclin D1, cyclin D3 and CDK2.
3D cell culture study
2D culture allows for the analysis of acute phase (2 days) of mechanism of action. However, B16F10 cells have fast growth speed in 2D culture and observation period is short. Second, it is not easy to change medium without removing the nanocarriers. 3D culture is an ideal approach to solve these problems. Tumour cells grow slower with the growth speed closer to that in the body. Moreover, nanocarriers trapped in the 3D gel allow one to change and refresh the culture medium. 3D culture allows us to prolong the observation period on B16F10 melanoma cells viability to 30 days instead of only 2 days using 2D culture. We use collagen that is a natural matrix component similar to body tissue. Another advantage is that at the completion of the experiments, collagenase can be used to digest the gels and to release the cells for detailed analysis. 3D culture can also help us to analyze a suitable dosage for in vivo study. Thus, the information from the 3D culture can be a better guide for in vivo studies. The following detailed 3D culture study showed that nanocarrier–PH-TYR (1) lowered the tyrosine level in the B16F10 cells to 1% of the control, (2) decreased viable B16F10 cells to less than 10% of the control, (3) inhibits migration (metastasis) significantly (p < 0.005) compared to the control and (4) arrests cell cycle of B16F10 melanoma cells in G0/G1, and the expression of cyclin D1, cyclin D3and CDK2 were also decreased.
Tyrosine removal
Polylactide nanocarrier containing PH-TYR (Ncap-PH-TYR) lowered the B16F10 cells tyrosine level to 1% compared to the control (). Only polylactide nanocarriers with the component of tyrosinase (Ncap-PH-TYR) could lower tyrosine, since the vehicle (Ncap) or polylactide nanocarrier containing polyhaemoglobin without tyrosinase (Ncap-PH) were not able to lower the tyrosine level.
Figure 2. The effects of different components on B16F10 melanoma cell tyrosine levels in 3 D cell culture at 0 h, 24 h and 48 h. Ctr: control with saline. Ncap: empty polylactide nanocarrier. Ncap-PH: polylactide nanocarrier containing polyhemoglobin. Ncap-PH-TYR200 and Ncap-PH-TYR400 polylactide nanocarrier containing poly-[haemoglobin-tyrosinase] with 200 units or 400 units of tyrosinase. Results are expressed as mean + s.e.m. Single asterisk indicates p < 0.05. Double asterisk indicates p < 0.005 compared with control.
![Figure 2. The effects of different components on B16F10 melanoma cell tyrosine levels in 3 D cell culture at 0 h, 24 h and 48 h. Ctr: control with saline. Ncap: empty polylactide nanocarrier. Ncap-PH: polylactide nanocarrier containing polyhemoglobin. Ncap-PH-TYR200 and Ncap-PH-TYR400 polylactide nanocarrier containing poly-[haemoglobin-tyrosinase] with 200 units or 400 units of tyrosinase. Results are expressed as mean + s.e.m. Single asterisk indicates p < 0.05. Double asterisk indicates p < 0.005 compared with control.](/cms/asset/f94df8d4-d3fb-426f-8d18-ad8c32e86cd5/ianb_a_1918134_f0002_b.jpg)
Effect on growth of melanoma cells
In the test groups (Ncap-PH-TYR200 and Ncap-PH-TYR400) the viable cell numbers decreased to less than 10% of the control (). The carrier itself (Ncap), did not inhibit the growth. The nanocarrier containing only polyhaemoglobin (Ncap-PH) only had a small effect on the tumour growth. In earlier 2D culture study, Ncap-PH increased the ROS, reactive oxygen radicals, that has some effects on the viability of the melanoma cells. However, both in the 2D culture study and in the present 3D study, the lowering of tyrosine by the Ncap-PH-TYR was more effective
Figure 3. The effects of different components on viable B16F10 melanoma cell count in 3 D cell culture at 24 h. Ctr: control with saline. Ncap: empty polylactide nanocarrier. Ncap-PH: polylactide nanocarrier containing polyhaemoglobin. Ncap-PH-TYR200 and Ncap-PH-TYR400: polylactide nanocarrier containing poly-[haemoglobin-tyrosinase] with 200 units or 400 units of tyrosinase. Results are expressed as mean + s.e.m. Single asterisk indicates p < 0.05. Double asterisk indicates p < 0.005 compared with control.
![Figure 3. The effects of different components on viable B16F10 melanoma cell count in 3 D cell culture at 24 h. Ctr: control with saline. Ncap: empty polylactide nanocarrier. Ncap-PH: polylactide nanocarrier containing polyhaemoglobin. Ncap-PH-TYR200 and Ncap-PH-TYR400: polylactide nanocarrier containing poly-[haemoglobin-tyrosinase] with 200 units or 400 units of tyrosinase. Results are expressed as mean + s.e.m. Single asterisk indicates p < 0.05. Double asterisk indicates p < 0.005 compared with control.](/cms/asset/563ca859-a606-4c7b-9ab8-3e310a36498f/ianb_a_1918134_f0003_b.jpg)
Effects on B16F10 melanoma cell cycle
A majority of B16F10 melanoma cells were arrested in G0/G1 when treated with Ncap-PH and Ncap-PH-TYR50. It was time dependent (). In the cell cycle of B16F10 melanoma cells, the cyclin D and their attendant CDKs are key regulators of G1 progression, which is an important checkpoint of cell cycle. Our results showed that the expression of cyclin D1, cyclin D3and CDK2 was also decreased.
Figure 4. The effects of different components on B16F10 melanoma cell cycle in 3D cell culture at 24 h and 48 h. Ctr: control with saline. Ncap-PH: polylactide nanocarrier containing polyhaemoglobin. Ncap-PH-TYR50 polylactide nanocarrier containing poly-[haemoglobin-tyrosinase] with 50 units of tyrosinase.
![Figure 4. The effects of different components on B16F10 melanoma cell cycle in 3D cell culture at 24 h and 48 h. Ctr: control with saline. Ncap-PH: polylactide nanocarrier containing polyhaemoglobin. Ncap-PH-TYR50 polylactide nanocarrier containing poly-[haemoglobin-tyrosinase] with 50 units of tyrosinase.](/cms/asset/f26d2a5d-0fc4-4be9-8671-38845522b2a7/ianb_a_1918134_f0004_b.jpg)
Effects on migration (metastasis)
One reason for the malignant feature of melanoma is its ability to metastasize. Thus, we studied the invasive migration of tumour cells. The scratch area for the control group decreased quickly with time showing the migration ability of B16F10 melanoma cells. Treatment with Ncap-PH-TYR50 inhibited migration significantly (p < 0.005). Ncap-PH also resulted in some inhibition of migration (p < 0.05), but not as effective as Ncap-PH-TYR50 ().
Figure 5. The effects of different components on B16F10 melanoma migration in 3D cell culture. Ctr: control with saline. Ncap-PH: polylactide nanocarrier containing polyhemoglobin. Ncap-PH-TYR50 polylactide nanocarrier containing poly-[haemoglobin-tyrosinase] with 50 units of tyrosinase. Results are expressed as mean + s.e.m. Single asterisk indicates p < 0.05. Double asterisk indicates p < 0.005 compared with control.
![Figure 5. The effects of different components on B16F10 melanoma migration in 3D cell culture. Ctr: control with saline. Ncap-PH: polylactide nanocarrier containing polyhemoglobin. Ncap-PH-TYR50 polylactide nanocarrier containing poly-[haemoglobin-tyrosinase] with 50 units of tyrosinase. Results are expressed as mean + s.e.m. Single asterisk indicates p < 0.05. Double asterisk indicates p < 0.005 compared with control.](/cms/asset/14eac5f6-9e67-46a4-890a-b16ff1b2eb61/ianb_a_1918134_f0005_b.jpg)
In vivo suppression of melanoma growth in mice
Based on the result of the 3D culture study we carried out study in C57BL6 mice using two melanoma tumour cell loads: melanoma cell load of 0.5 × 105 B16F10 melanoma cells/0.15 ml test suspension and (2) doubled the melanoma cell load to 1.0 × 105 B16F10 melanoma cells/0.15 ml test suspension. This study was approved by the McGill University animal research ethics committee (McGill University Animal Use Protocol – no. 3816).
Summary
The in vivo effect of polylactide-nanocarriers containing PH-TYR on B16F10 murine melanoma was studied in C57BL6 mice. C57BL6 mice were purchased from Charles River Canada. Mice were kept with a 12 h light/dark schedule and fed conventional food and water ad lib. All animals were housed and cared for according to the animal care regulations of McGill University. All mice were acclimatized for at least 7 days prior to use in the experiment.
B16F10 murine melanoma cell suspension with different test solutions were used. (1) Saline, (2) polylactide nanocarriers containing PH-TYR, (3) polylactide nanocarriers with no contents, (4) Free PH-TYR in solution, (5) polylactide–nanocarriers containing only polyhaemoglobin. Each suspension was inoculated subcutaneously into the lateral flank of C57BL6 mice. A total of 6 mice were used for each group. The primary tumour size and body weight were followed every 2 days. The tumour volume was calculated as V = (A*B2)/2, where V is the volume (mm3); A is the longest diameter (mm); B is the shortest diameter (mm). Single asterisk indicated p < 0.05 compared with control. At the endpoint, the tumour tissues were collected for histological analysis.
Two B16F10 cell loads/test suspension volume were tested: (1) study using cell load of 0.5 × 105 B16F10 melanoma cells/0.15 ml test suspension and (2) study using cell load of 1.0 × 105 B16F10 melanoma cells/0.15 ml test suspension. In animal study we started with a tumour cell load of 0.5 × 105 B16F10 melanoma cells/0.15 ml test suspension. Ncap-PH-TYR and Ncap-PH effectively inhibited the growth of melanoma without changing the growth of the animal. When we doubled the tumour load to 1.0 × 105 B16F10 melanoma cells/0.15 ml test suspension, Ncap-PH with 200 units of tyrosinase could no longer inhibit the tumour growth during the 30 days. Ncap-PH-TYR with 800 units of tyrosinase was needed to inhibit the growth. In the control group, the large tumour nodules consisted of neoplastic tissue with viable tumour cells, necrosis, haemorrhage with no visible nanoparticles. In the Ncap-PH-TYR group, there was only foreign body granuloma tissue with no neoplastic cells but with visible nanocarriers. There was no change in body weight. There was no change in the histology of the lung, liver, spleen and kidney.
In vivo effect of cell load of 0.5 × 105 B16F10 melanoma cells/0.15 ml test suspension
Effect of polylactide nanocarriers containing PH-TYR
We first followed the effect of polylactide nanocarriers containing PH-TYR on B16F10 melanoma cells bearing mice model. Soluble PH-TYR inside the nanocarriers were prevented from being dispersed quickly after subcutaneous injection thus allowing it to accumulate longer in the injection sites (). Furthermore, in cell culture study, these could enter the melanoma cells to act inside the cells () [Citation16,Citation17]. In this way, it could lower the tyrosine level in both the tumour cells and in the tumour microenvironment ().
Unlike the control group, the two treatment groups all exhibited significant tumour inhibition as shown by the tumour volume when followed for 30 days (). There was no change in body weight.
Figure 6. In vivo effect on growth of melanoma and body weight. Single asterisk indicates p < 0.05 compared with control. n = 6 mice for each group. Ctr: control with saline. Ncap-PH-TYR400 and Ncap-PH-TYR 800, polylactide nanocarrier containing poly-[haemoglobin-tyrosinase] with 400 and 800 units of tyrosinase.
![Figure 6. In vivo effect on growth of melanoma and body weight. Single asterisk indicates p < 0.05 compared with control. n = 6 mice for each group. Ctr: control with saline. Ncap-PH-TYR400 and Ncap-PH-TYR 800, polylactide nanocarrier containing poly-[haemoglobin-tyrosinase] with 400 and 800 units of tyrosinase.](/cms/asset/7392e3c9-8dd1-4f86-9f31-9a3490d595bc/ianb_a_1918134_f0006_c.jpg)
In vivo effects of polylactide nanocarrier vehicle
Our result showed that the polylactide nanocarrier vehicle itself has no tumour suppressive effect when compared with the control group (). There was also no difference in body weight (). The HE stain and melanoma cells-specific stain, Melan-A, CD31 and Ki67, all showed no differences compared with the control group. In addition, the histological analysis showed no improvement on tumour cells killing. Polylactide nanocarriers did not have any adverse effects on the histology the lung, liver, spleen and kidney. These indicated that the polylactide polymer itself had no impact on the inhibition of tumour growth. However, it played an important role as a nanocarrier vehicle for the PH-TYR. Without this, the soluble PH-TYR would not be able to stay at the site of the melanoma cells to carry out its function as shown in .
In vivo effect of the soluble PH-TYR component
Soluble PH-TYR containing 400 units of tyrosinase, but without the nanocarrier could not inhibit the growth of B16F10 melanoma () The soluble PH-TYR being a solution would diffuse out throughout the body from the site of injection and would not be able to stay at the site of injection of the melanoma cells to carry out its function. This further showed the important role of the nanocarrier vehicle for the PH-TYR. Without this, it cannot function adequately as shown in .
In vivo effects of component polylactide nanocarrier polyhaemoglobin (Ncap-PH)
The results showed that polylactide Ncap-PH could suppress the tumour growth () without any adverse effect on body weight (). Consistent with this, the HE stain and melanoma cells-specific stains, Melan-A, CD31 and Ki67, all showed significant differences in comparison to the control group. In addition, the histological analysis also verifies that few remaining tumour cells are found. Indeed, in our 2D study, we find that the polyhaemoglobin component of polylactide nanocarrier–polyhaemoglobin increases ROS production [Citation16,Citation17]. Others had also shown that ROS inhibits tumour growth [Citation18]. Our 3D culture studies () also showed that polylactide nanocarrier–polyhaemoglobin has some effect on melanoma cells though not as much as the polylactide nanocarrier–PH-TYR. In our earlier 2D culture study, tracking ROS generation with CellROX reagent showed that intracellular ROS generation significantly increased for 24 h and 48 h when compared with the untreated control. The generation of ROS by the polylactide nanocarrier–polyhaemoglobin itself was only effective at lower melanoma cells loads but sufficient tyrosinase would be needed when the melanoma cell load was doubled ().
Figure 9. In vivo effect on growth of melanoma and body weight, n = 6 mice for each group. Ctr: control with saline. Ncap-PH: polylactide nanocarrier containing polyhaemoglobin.
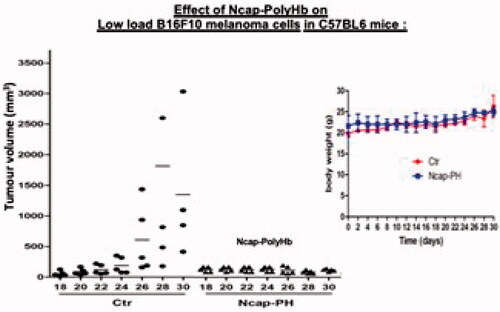
Figure 10. Effect of tumour cell load on the suppression of tumour growth. Ncap-PolyHb or Ncap-PolyHb-Tyr200 (nanocarrier containing polyhaemoglobin or nanocarrier containing polyhaemoglobin-tyrosinase with 200 units of tyrosinase) could suppress the growth of the tumour cell load of 0.5 × 105 but not when the tumour cell load was increased to 1.0 × 1010. When the tumour cell load was increased to 1.0 × 1010 Ncap-PolyHb-Tyr800 (nanocarrier containing polyhaemoglobin-tyrosinase with 800 units of tyrosinase) was needed to suppress the growth.
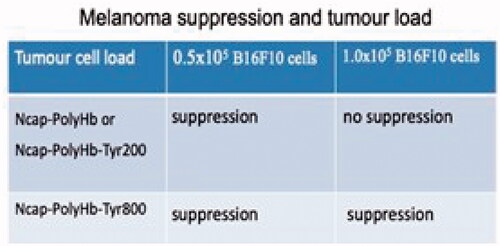
Effect of doubling the tumour cell load/1.5 ml nanocarrier suspension
At a cell load of 0.5 × 105/0.15 ml test suspension of Ncap-PolyHb or Ncap-PolyHb-Tyr200 (nanocarrier containing polyhaemoglobin or nanocarrier containing PH-TYR with 200 units of tyrosinase) could suppress the growth of the tumour (). However, doubling the cell load to 1.0 × 105 B16F10 cells/0.15 ml polylactide Ncap-PH or Ncqp-PH-TYR without sufficient tyrosinase could no longer inhibit melanoma growth even with tyrosinase of 200 units (). This shows that the generation of ROS by the polylactide nanocarrier–polyhaemoglobin itself is only effective at lower melanoma cells loads but sufficient tyrosinase would be needed when the melanoma cell load increases. We, therefore, carry out a detail study with the higher tumour load of ratio of 1.0 × 105 B16F10 melanoma cells/0.15 ml test suspension of polylactide nanocarriers containing PH-TYR with 800 unit of tyrosinase
In vivo effect of a tumour cell load of 1.0 × 105 B16F10 melanoma cells/0.15 ml Ncap-PH-TYR800 (800 units of tyrosinase)
At a tumour cell load of 1.0 × 105 B16F10 melanoma cells/0.15 ml, Ncap-PH-TYR800 (polylactide nanocarriers containing PH-TYR with 800 unit of tyrosinase) could inhibit the tumour growth during the 30 days (). The nodule of the Ncap-PH-TYR800 group after 30 days was significantly smaller than the control group. Tissues sections were stained with haematoxylin and eosin and analyzed by the pathologist. The expression profiles of Melan-A were analyzed by immunohistochemistry (IHC) methods. In the control group, histology of the large nodules () showed that they consisted of neoplastic tissue with viable tumour cells, necrosis, haemorrhage with no visible nanoparticles. In the Ncap-PH-TYR800 group, the small noodles consisted of foreign body granuloma tissue with no neoplastic cells but with visible nanocarriers (). There was no change in body weight (). There was no change in the histology of the lung, liver, spleen and kidney. The nanocarriers are injected locally and not intravenously and therefore did not enter the circulation to reach these organs.
Figure 11. (a) In vivo effect of Ncap-PH-TYR800 (polylactide nanocarrier containing poly-[haemoglobin-tyrosinase] with 800 units of tyrosinase) compared to Ctr (control with saline) on higher tumour load (1.0 × 105 B16F10 melanoma tumour cells: 0.15 ml Ncap-PH-TYR800. n = 6 mice for each group. Left: tumour volumes followed every 2 days for 30 days. Right: effect on growth as shown by body weight. (b) Pathology report of the injection site: in vivo effect of Ncap-PH-TYR800 (polylactide nanocarrier containing poly-[haemoglobin-tyrosinase] with 800 units of tyrosinase) compared to Ctr (control with saline) on higher tumour load (1.0 × 105 B16F10 melanoma tumour cells: 0.15 ml Ncap-PH-TYR800.
![Figure 11. (a) In vivo effect of Ncap-PH-TYR800 (polylactide nanocarrier containing poly-[haemoglobin-tyrosinase] with 800 units of tyrosinase) compared to Ctr (control with saline) on higher tumour load (1.0 × 105 B16F10 melanoma tumour cells: 0.15 ml Ncap-PH-TYR800. n = 6 mice for each group. Left: tumour volumes followed every 2 days for 30 days. Right: effect on growth as shown by body weight. (b) Pathology report of the injection site: in vivo effect of Ncap-PH-TYR800 (polylactide nanocarrier containing poly-[haemoglobin-tyrosinase] with 800 units of tyrosinase) compared to Ctr (control with saline) on higher tumour load (1.0 × 105 B16F10 melanoma tumour cells: 0.15 ml Ncap-PH-TYR800.](/cms/asset/10936928-b5d6-4642-a714-3bcd49260412/ianb_a_1918134_f0011_c.jpg)
Discussion
A recent editorial emphasized the need to analyze the safety and clinical feasibility of research [Citation5]. We have indeed carried out such analysis starting as the first to use polylactide for the bioencapsulation of biologically active materials [Citation19]. Polylactide biodegrades in the body into lactic acid and finally carbon dioxide and water [Citation19]. It is now a FDA approved polymer [Citation20] and is used in a number of drug carriers [Citation6,Citation13–15,Citation20]. For intravenous injection, polyethyleneglycol–polylactide (PEG-PLA) is used to increase its circulation time. However, we used dl-PLA because we administered this by local injection to function at the site of injection. In animal study we started with a tumour cell load ratio of 0.5 × 105 B16F10 melanoma cells/0.15 ml test suspension. Both Ncap-PH and Ncap-PH-TYR effectively inhibited the growth of melanoma without changing the growth of the animal. Ncap-PH containing polyhaemoglobin acted as an oxygen therapeutic converting oxygen into oxygen radicals ROS [Citation12]. Ncap-PH-TYR contained PH-TYR and acted as a combined system. We then carried out more detail study using double the tumour load of 1.0 × 105 B16F10 melanoma cells/0.15 ml test suspension. With this higher tumour load, ROS of the oxygen therapeutic alone or even Ncap-PH-TYR200 containing only 200 units of tyrosinase was not sufficient. A higher tyrosinase activity of 800 units was needed to be effective. Ncap-PH-TYR800 could inhibit the tumour growth when followed for 30 days. In the control group, the large tumour nodules consisted of neoplastic tissue with viable tumour cells, necrosis, haemorrhage with no visible nanoparticles. In the Ncap-PH-TYR group, there was only foreign body granuloma tissue with no neoplastic cells but with visible nanocarriers. This further supported the presence of the Ncap-PH-TYR800 at the tumour site where it suppressed the tumour growth. There was no change in body weight. There was no change in the histology of the lung, liver, spleen and kidney.
The editorial also mentioned the problem that with intravenous administration of different drug carrier systems, the delivery efficiency was less than 1% [Citation4]. In the present study of local injection, the nanoencapsulation efficiency of PH-TYR into the nanocarrier is 75.4% [Citation16,Citation17]. In the present report, by administrating this locally, most of this reached the site of injection. Analysis at the site of injection showed a small foreign body granular containing the nanocarriers with no inflammation. With time the polylactide–lipid will be biodegraded. Decreasing the molecular weight of the polylactide would increase the rate of biodegradation of the polymer. The content of PH-TYR will be metabolized like another of our enzyme–oxygen therapeutic poly-[haemoglobin–catalase–superoxide dismutase-carbonic anhydrase] that did not show any safety or immunological problem [Citation21] and is now under development for clinical trial.
The results obtained shows promise of safety and clinical feasibility and encourages further research and development of this approach.
Acknowledgements
Professor TMS Chang acknowledges the grant support from the Canadian Institutes of Health Research [#39609]. Yun Wang is the recipient of a graduate student scholarship from the China Scholarship Council.
Disclosure statement
No potential conflict of interest was reported by the authors.
Data availability statement
More details and data are available in http://www.medicine.mcgill.ca/artcell
References
- NCI National Cancer Institute. 2020. The cancer genome atlas (TCGA). Available from: https://www.cancer.gov/tcga.
- World Health Organization. 2020. Cancer mortality database. Available from: http://www-dep.iarc.fr/WHOdb/WHOdb.htm.
- Andersen R, Donia M, Ellebaek E, et al. Long-lasting complete responses in patients with metastatic melanoma after adoptive cell therapy with tumor-infiltrating lymphocytes and an attenuated IL2 regimen. Clin Cancer Res. 2016;22(15):3734–3745.
- Wilson MA, Schuchter LM. Chemotherapy for melanoma. Cancer Treat Res. 2016;167:209–229.
- Editorial. The two directions of cancer nanomedicine. Nat Nanotechnol. 2019;14:1083.
- Riley RS, June CH, Langer R, et al. Delivery technologies for cancer immunotherapy. Nat Rev Drug Discov. 2019;18(3):175–196.
- Gershenwald JE, Scolyer RA, Hess KR, for members of the American Joint Committee on Cancer Melanoma Expert Panel and the International Melanoma Database and Discovery Platform, et al. Melanoma staging: evidence-based changes in the American joint committee on cancer eighth edition cancer staging manual. CA Cancer J Clin. 2017;67(6):472–492.
- Perego M, Maurer M, Wang JX, et al. A slow-cycling subpopulation of melanoma cells with highly invasive properties. Oncogene. 2018;37(3):302–312.
- Du J, Su Y, Qian C, et al. Raman-guided subcellular pharmaco-metabolomics for metastatic melanoma cells. Nat Commun. 2020;11(1):4830.
- Meadows GG, Fu YM. 2005. Dietary restriction of specific amino acids modulates tumor and host interactions. In: Meadows GG, editor. Integration/interaction of oncologic growth. Cancer growth and progression, Vol. 15. Dordrecht: Springer. p. 271.
- Slominski A, Zmijewski MA, Pawelek J, et al. l-Tyrosine and l-dihydroxyphenylalanine as hormone-like regulators of melanocyte functions. Pigment Cell Melanoma Res. 2012;25(1):14–27.
- Chang TMS. Monograph on “ARTIFICIAL CELLS: biotechnology, nanotechnology, blood substitutes, regenerative medicine, bioencapsulation, cell/stem cell therapy”. Singapore: World Scientific Publisher/Imperial College Press; 2007. 435 pp. (Since April 2010 free online viewing http://www.medicine.mcgill.ca/artcell/2007ebookartcellweb.pdf).
- Chang TMS. Therapeutic applications of polymeric artificial cells. Nat Rev Drug Discov. 2005;4(3):221–235.
- Chang TMS. ARTIFICIAL CELL evolves into nanomedicine, biotherapeutics, blood substitutes, drug delivery, enzyme/gene therapy, cancer therapy, cell/stem cell therapy, nanoparticles, liposomes, bioencapsulation, replicating synthetic cells, cell encapsulation/scaffold, biosorbent/immunosorbent haemoperfusion/plasmapheresis, regenerative medicine, encapsulated microbe, nanobiotechnology, nanotechnology. Artif Cells Nanomed Biotechnol. 2019;47(1):997–1013. https://www.tandfonline.com/doi/full/10.1080/21691401.2019.1577885
- Poon W, Kingston BR, Ouyang B, et al. A framework for designing delivery systems. Nat Nanotechnol. 2020;15(10):819–829.
- Wang Y, Chang TM. 2012. Nanobiotechnological nanocarriers containing polyhemoglobin-tyrosinase: effects on murine B16F10 melanoma cell proliferation and attachment. J Skin Cancer 2012: 673291. http://www.hindawi.com/journals/jsc/2012/673291/.
- Wang Y, Chang TMS. Biodegradable nanocarriers containing a nanobiotechnological complex for the in-vitro suppression of a melanoma cell line B16F10. J Nanosci: Curr Res. 2016;1:1–6. www.medicine.mcgill.ca/artcell/2016.pdf
- Zhang Q, Guo X, Cheng Y, et al. Use of copper-cysteamine nanoparticles to simultaneously enable radiotherapy, oxidative therapy and immunotherapy for melanoma treatment. Sig Transduct Target Ther. 2020;5(1):58.
- Chang TM. Biodegradable semipermeable microcapsules containing enzymes, hormones, vaccines, and other biologicals. J Bioeng. 1976;1(1):25–32.
- Parveen S, Sahoo SK. Polymeric nanoparticles for cancer therapy. J Drug Target. 2008;16(2):108–123.
- Guo C, Chang TMS. Long term safety and immunological effects of a nanobiotherapeutic, bovine poly-[hemoglobin-catalase-superoxide dismutase-carbonic anhydrase], after four weekly 5% blood volume top-loading followed by a challenge of 30% exchange transfusion. Artif Cells Nanomed Biotechnol. 2018;46(7):1349–1363. http://www.artcell.mcgill.ca/safety_immune.pdf