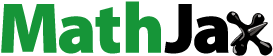
Abstract
In this research, we formulated new chemotherapeutic copper nanoparticles (Cu NPs) containing Allium noeanum Reut. ex Regel leaf for treating human endometrial cancer. For investigating the antioxidant activitiy, the 2,2-diphenyl-1-picrylhydrazyl (DPPH) test was used. MTT test was used on normal (Human umbilical vein endothelial cells (HUVECs)) and human endometrial cancer (Ishikawa, HEC-1-A, HEC-1-B, and KLE) cell lines for comparing the anti-human endometrial cancer properties of Cu(NO3)2, A. noeanum leaf aqueous extract, and copper nanoparticles. Copper nanoparticles had high cell death and anti-human endometrial cancer effects against Ishikawa, HEC-1-A, HEC-1-B, and KLE cell lines. The IC50 of A. noeanum leaf aqueous extract and copper nanoparticles against HEC-1-B cell line were 548 and 331 µg/mL, respectively; against HEC-1-A cell line were 583 and 356 µg/mL, respectively; against KLE cell line were 609 and 411 µg/mL, respectively; and against Ishikawa cell line were 560 and 357 µg/mL, respectively. Among the above cell lines, the best result of anti-human endometrial cancer properties of copper nanoparticles was gained in the cell line of HEC-1-B. This study indicated excellent anti-human endometrial cancer potentials of copper nanoparticles containing A. noeanum in the in vitro condition.
Introduction
Cancer is a global health issue and induces uncontrolled division and abnormal growth of cells [Citation1,Citation2]. Endometrial cancer is a cancer of the uterus lining. Endometrial cancer is the fifth leading cancer among women worldwide, with 320,000 new cases diagnosed in 2012, which accounts for 4.8% of all cancers in women [Citation1,Citation2]. Endometrial cancer is the third leading cancer in the Western world, accounting for 6–9% of all cancers in women [Citation3]. Endometrial cancer is often detectable in the early stages due to the nature of the disease and incidence of uterine and/or vaginal bleeding, with a 5-year survival rate of 85–91% [Citation2]. About 73% of patients with endometrial cancer are diagnosed in stage I, and the diagnosis may be made in stage II of the disease for about 10% of patients [Citation1,Citation2]. The endometrial cancer risk factors are increasing age, late menopause, tamoxifen, obesity, high levels of oestrogen, breast cancer, never having had a child, and diabetes mellitus. The signs of endometrial cancer are infertility, vaginal bleeding, uterine bleeding, pelvic pain, pain during sexual intercourse, and pain with urination [Citation1,Citation2]. Cervical screening tests, pap smear, transvaginal ultrasound, endometrial biopsy, CT scan, and hysteroscopy are used to diagnose endometrial cancer [Citation3]. For treating endometrial cancer, radiation therapy, chemotherapy, and immunotherapy are used. The major anti-endometrial cancer chemotherapeutic supplements/drugs are paclitaxel, docetaxel, doxorubicin, carboplatin, and cisplatin [Citation2–4]. Due to severe side effects of the chemotherapeutic drugs and supplements, the formulation of the chemotherapeutic drugs from metallic nanoparticles such as copper nanoparticles is the research priority of pharmacology, oncology, and organic chemistry researchers [Citation4–6]. Nanotechnology has emerged as a novel strategy to design smart nanostructure weapons to combat endometrial cancer. Recently, the biogenic copper nanoparticles (CuNPs) have attracted highlighted interest for endometrial cancer [Citation4,Citation6].
Nanoparticles, which are the subject of our study, have unique competencies different from their macro-scale counterparts due to their high surface/volume ratio and many advanced and new physiochemical properties such as colour, solubility, strength, prevalence, toxicity, magnetic, optical, thermodynamics [Citation6]. In recent years, biological methods that non-toxic, cost-effective, and environmentally friendly have become the focus of interest compared to physicochemical nanoparticle synthesis methods [Citation5,Citation6]. The synthesis of nanoparticles under purely “green” principles can be achieved by using an environmentally compatible solvent system with environmentally friendly reducing and stabilising agents. Various pathways have been developed for the biogenic or biological synthesis of nanoparticles from the salts of different metal ions [Citation7–9]. Microorganisms, marine algae, plant extracts, plant tissue, fruits, and all plants are used to synthesise nanoparticles [Citation7]. The basic principle in the biogenesis of nanoparticles is the reduction of metal ions of various biomolecules such as amino acids, polysaccharides, enzymes/proteins, and vitamins found in organisms. In addition to reducing the environmental impact of biological synthesis, it enables the production of large quantities of nanoparticles, which are well defined in size and morphology, independent of contamination [Citation8,Citation10i]. The reduction of metal ions using plant extracts has been a known method since the 1900s.
Preparation of Fe3O4 NPs via green synthesis approach using non-toxic, environmentally benign materials, which offers numerous benefits of eco-friendliness and compatibility for pharmaceutical, biotechnological and biological applications [Citation10a]. More recently, plant-mediated green synthesis of nanoparticles has attracted much attention due to cost-effective, environmentally friendly alternative to chemical and physical methods. There are successful reports for plant-mediated synthesis of Fe3O4 NPs using various plant extracts such as seed extract of Grape proanthocyanidin [Citation10b], leaf extract of Tridaxprocumbens [Citation10c], seaweed of Kappaphycusalvarezii [Citation10d], Caricaya papaya [Citation10e], peel extract of plantain [Citation10f], and seaweed of Sargassummuticum [Citation10g].
Nanotechnology is a growing science that may provide several new applications for medicine, food preservation, diagnostic technologies, and sanitation. Despite its beneficial applications, there are several questions related to the safety of nanomaterials for human use. The genotoxicity of different types of metal NPs should be noted before using [Citation10h].
Recently, scientists have revealed that medicinal plants green synthesised-metallic nanoparticles have excellent anti-cancer properties [Citation8,Citation9,Citation11,Citation12]. Metallic nanoparticles have achieved notable consideration in the field of medicine. Some studies conducted today have shown that some nanoparticles have therapeutic properties and it is an excellent alternative to physicochemically different metal-supported nanoparticles, antibacterial, and especially anticancer drugs [Citation10i,Citation13]. Medicinal plants green synthesised-copper nanoparticles as a special type of them well-known metallic nanoparticles have recently been used for the cure of several types of tumours and cancers [Citation8]. A study was reported the anti-human breast cancer properties of copper nanoparticles in the cellular and molecular conditions. In the previous study, copper nanoparticles significantly killed all malignant breast cells (MCF-7 cells) in the nano concentrations [Citation10i]. In another study, copper nanoparticles synthesised by Benzimidazole indicated the remarkable anticancer effects against cancer cells, i.e. A549 and NIH/3T3 cell lines [Citation10]. The report of Chatterjee et al. was indicated that the copper materials in the nano concentrations had excellent anticancer potentials against rat glioblastoma C6-G, human lung cancer A-549, and human skin melanoma A-375 [Citation14]. In the previous research, the IC50 of copper nanoparticles was 1.88, 1.81, and 1.71 µg/mL for the rat glioblastoma C6-G, human lung cancer A-549, and human skin melanoma A-375, respectively. Azizi et al. indicated excellent anticancer activities of copper nanoparticles against normal MCF-10A counterparts and invasive MDA-MB 231 cancer cells [Citation15].
The history of science is an attractive area of interdisciplinary knowledge and research in humanity. Medical sciences are among the fascinating areas pertinent to the history of science. Traditional medicine is one of the main fields of medicine in the world [Citation5,Citation7,Citation8,Citation16]. In this context, it is predicated that Allium noeanum Reut. ex Regel leaves have significant anticancer properties. The antioxidant compounds of Allium genus are Allicin[diallyl thiosulfinate], tuberoside M, S-allylmercaptocysteine, thiosulfinates, S-propargyl-L-cysteine, Ace-AMP1, quercetin, S-benzyl-cysteine, diosgenin, polysulfanes, diosgenin, fisetin, diallylpolysulfides, onionin A, diallylpolysulfides, Z-ajoene, allyl mercaptan, amino-styrene-acrylic acids, flavonoids, furostanol saponins, allyl sulphides, saponins, glycosides, diallyl tetrasulfide, garlic oil, S-Propargyl-cysteine, diallyl monosulfide, and Z-ajoene [Citation17]. Previously, the anticancer, hepatoprotective, preventive cardiovascular diseases, nephroprotective, preventive heart diseases, hematoprotetive, anti-inflammatory, antiobesity, antidiabetes, immunoprotective, neuroprotective, antifungal, antiviral, and antibacterial effects of Allium genus have been proven [Citation17]. The anticancer properties of the Allium genus are related to the S-benzyl-cysteine, diosgenin, polysulfanes, diosgenin, fisetin, allicin[diallyl thiosulfinate], tuberoside M, S-allylmercaptocysteine, thiosulfinates, S-propargyl-L-cysteine, Ace-AMP1, and quercetin. In traditional medicine, the plant is used to treat many cancers such as breast, ovarian, stomach, and prostate cancers and blood disorders [Citation17].
In the recent experiment, we decided to survey the anti-human endometrial cancer potentials of copper nanoparticles synthesised by A. noeanum leaf aqueous extract against human endometrial cancer cell lines.
Experimental
Materials and apparatus
Antimycotic antibiotic solution, dimethyl sulfoxide (DMSO), hydrolyzate, Ehrlich solution, decamplmaneh foetal bovine serum, borax-sulfuric acid mixture, Dulbazolic mixture Modified Eagle Medium (DMED), 4-(Dimethylamino) benzaldehyde, 2,2-diphenyl-1-pikrilhydrazil (DPPH) and phosphate buffer solution (PBS) were supplied from the US Sigma-Aldrich company. All the reagents were purchased from Aldrich and Merck and were used without any purification. In the instrumental section, the particle size, morphology and atomic mapping analysis of the nanomaterial was investigated using a FESEM-TESCAN MIRA3 microscope equipped with EDX. The crystalline structures of the samples were evaluated by X-ray diffraction (XRD) analysis on a Bruker D8 Advance diffractometer with CuKα radiation at 40 kV and 20 mA. Fourier transform infra-red (FT-IR) spectra were recorded with a Perkin Elmer 65 spectrometer in the range of 400–4000 cm−1. Transmission electron microscopy (TEM) analysis was performed on a Phillips CM10 microscope at an accelerating voltage of 200 kV. Magnetisation measurements were carried out on a BHV-55 vibrating sample magnetometer (VSM).
Green synthesis of Cu nanoparticles
To obtain the aqueous extract of the plant, 250 g of the dried branches of the A. noeanum leaves were poured in a container containing 2000 ml boiled water, and the container lid was tightly closed for 4 h. Then, the container’s content was filtered, and the remaining liquid was placed on a bain-marie to evaporate. Finally, a tar-like material was obtained, which was powdered by a freeze dryer [Citation8,Citation9].
The extract of the A. noeanum leave plant obtained for the green synthesis method was extracted with distilled water in the microwave. In a 50 ml 1 (mM) aqueous solution of Cu(NO3)2⋅2H2O, 5 ml of the A. noeanum leaves extract solution was added under vigorous stirring at 25 °C for 1 h. During this process, the solution colour changes to brick-red colour, showing the copper nanoparticles (Cu NPs) formation. The resultant solution was refluxed and let to precipitate; the precipitate subsequently was filtered and washed with acetone, distilled water, and ethanol. To obtain a fine powder of Cu nanoparticles, the resultant precipitate was finally dried at 90 °C for 14 h.
The as-prepared Cu NPs were thoroughly characterised using energy-dispersive X-ray spectroscopy (EDX), Fourier transform infra-red spectroscopy (FT-IR), field emission scanning electron microscopy (FE-SEM), ultraviolet–visible spectroscopy (UV–Vis), transmission electron microscopy (TEM) and X-ray diffraction (XRD) techniques.
Investigation of antioxidant property of Cu NPs
To study the radical scavenging antioxidant property of our Cu(NO3)2, A. noeanum leaf aqueous extract, and CuNPs, 2,2-diphenyl-1-picrylhydrazyl (DPPH) is being used [Citation18]. A 39.4% DPPH solution (w/V) was prepared in 1:1 aqueous MeOH. At the same time, different samples of Cu(NO3)2, A. noeanum leaf aqueous extract, and Cu NPs of variable concentrations (0–1000 µg/mL) were prepared. The DPPH solution was then added to Cu(NO3)2, A. noeanum leaf aqueous extract, and CuNPs samples and incubated at 37 °C. After 30 min of incubation, the absorbances of the mixtures were measured at 570 nm. MeOH (50%) and butylated hydroxytoluene (BHT) were considered as negative and positive controls for DPPH assay, respectively in the study. The antioxidant property of Cu(NO3)2, A. noeanum leaf aqueous extract, and Cu NPs was determined in terms of % inhibition and expressed as
Determination of anti-human endometrial cancer effects of Cu NPs
In this assay, following human endometrial cell lines and the normal cell line (Human umbilical vein endothelial cells (HUVECs)) were used to study the cytotoxicity and anticancer potential of human endometrial over the Cu(NO3)2, A. noeanum leaf aqueous extract, and Cu NPs using the common cytotoxicity test, i.e. MTT assay in vitro condition:
Normal cell line: HUVEC.
Endometrial cancer cell lines:
Ishikawa.
KLE.
HEC-1-A.
HEC-1-B.
For culturing the above cells, several materials including penicillin, streptomycin, and Dulbecco’s modified Eagle’s medium (DMEM) were used [Citation18]. The distribution of cells was 10,000 cells/well in 96-well plates. All samples were transferred to a humidified incubator containing 5% CO2 at 37 °C. After 24 h incubation, all cells were treated with the different Cu(NO3)2, A. noeanum leaf aqueous extract, and Cu NPs samples (0–1000 µg/mL) and incubated again for 24 h. Subsequently, they were sterilised by UV-radiation for 2 h. Then 5 mg/mL of MTT was added to all wells and incubated again for 4 h at 37 °C. The percentage of cell viability of samples were determined following the given formula after the measurement of absorbance at 570 nm.
Qualitative measurement
The obtained results were loaded into the “SPSS-22” program and evaluated by “one-way ANOVA,” accompanied by a “Duncan post-hoc” check (p ≤ 0.01).
Results and discussion
In the current research, copper nanoparticles were prepared and synthesised using an aqueous extract of A. noeanum leaf as stabilising and reducing agents. The as-prepared Cu NPs were thoroughly characterised using energy-dispersive X-ray spectroscopy (EDX), fourier transform infra-red spectroscopy (FT-IR), field emission scanning electron microscopy (FE-SEM), ultraviolet–visible spectroscopy (UV-Vis), transmission electron microscopy (TEM) and X-ray diffraction (XRD) techniques. Also, we investigated the anti-human endometrial cancer potentials of these nanoparticles against common human endometrial cancer (Ishikawa, HEC-1-A, HEC-1-B, and KLE) cell lines.
Chemical characterisation of Cu NPs
shows UV-Vis spectra of A. noeanum extract and copper nanoparticles obtained by green synthesis using A. noeanum extract plant. Analysis of the absorption spectrum of the final solution allowed us to more clearly understand this Cu2+–Cu0 conversion. The appearance band at a wavelength of about 585 nm after the green synthesis process, was evidence that the Cu2+ cation became completely reduced. It has been indicated in the previous study that the surface plasmon resonance (SPR) band of copper nanoparticles obtained by green synthesis using medicinal plants provides absorption from 570 to 600 nm [Citation19]. Surface plasmon resonance (SPR) is the manifestation of a resonance effect due to the interaction of conduction electrons of metal nanoparticles with incident photons. The interaction relies on the size and shape of the metal nanoparticles and on the nature and composition of the dispersion medium.
represents the FT-IR spectra of A. noeanum extract itself (a) and the biomolecule modified Cu NPs (b). The extract shows strong signals at 3400, 2865, and 1675 cm−1 attributed to phenolic O–H, C–H and C=O bonds respectively. The characteristic band at 621 cm−1 for the Cu NPs was ascribed to the vibrational mode of Cu–O in Cu phase. The FT-IR profile of the A. noeanum extract almost overlaps with the Cu NPs spectrum. The findings obtained from the spectra of FT-IR proved that copper nanoparticles are coated with extract biomolecules.
The structural morphology of the prepared material was analysed by the FE-SEM study. represents the particle shape and size. It assumes a wheat-like texture. In a closer look, the small globular particles of Cu NPs are seen. Due to high concentration in sampling the figure shows somewhat lump-like agglomeration. However, the surface immobilisation of biomolecules could not be observed from the images. Also, the sample FE-SEM mapping was assessed as well (). Considering the compositional maps Cu, O and C, the Cu NPs existence with excellent dispersion are analysed in the composite.
Figure 3. FESEM image of biosynthesized Cu NPs with its elemental mapping of C, O and Cu respectively.
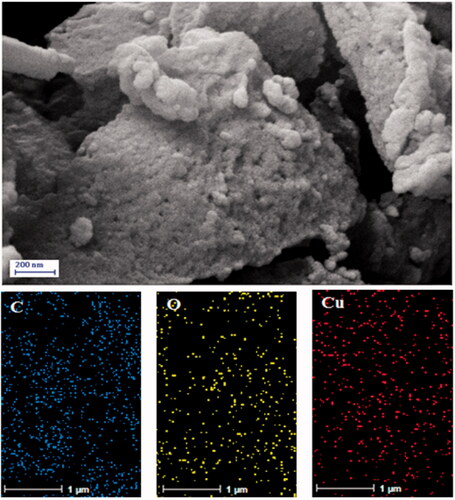
EDX test of the prepared material was carried out to assess the composition overall (). It exhibits the Cu and O atoms as core elements of the copper nanoparticles. The presence of a significant amount of C atoms signifies the attachment of organic species immobilised over the copper nanoparticles. The polyphenolic compounds from plant extract afford C and O atoms in the EDX spectrum.
TEM was used to present extra information on the size and structure and the morphology, shape and dimension of these NPs (). According to the results of TEM images, Cu nanoparticles were in the range sizes of ∼10–12 nm that were in excellent agreement with the XRD finding. The Cu nanoparticles with a high density and spherical shape had been well distributed. Also, some particles are interconnected to each other and formed bigger particles. Moreover, (inset) determines the particle size distribution histograms for Cu NPs whose average size are approximately 10.8 nm.
reveals the pattern of the material XRD, which clearly shows the crystalline nature of the Cu/A. noeanum composite. The several diffraction peaks appeared at the 2 Theta degree of 43.2°, 50.3° and 74.1° corresponds to the crystal planes (1 1 1), (2 0 0), and (2 2 0), respectively. All the peaks are authenticated standard data which satisfies with the highly pure crystalline Cu nanoparticles (JCPDS No. 04-0836).
Study of the radical scavenging antioxidant properties of copper nanoparticles in vitro
Free radicals are molecules that do not have a complete electron shell, which increases the chemical reaction relative to others. Free radicals are formed if you are exposed to tobacco smoke and radiation. In humans, the most important free radical is oxygen. When an oxygen molecule (O2) is exposed to radiation, it removes an electron from the other molecules, destroying DNA and other molecules [Citation20,Citation21]. Some of these changes cause disease. Problems such as heart problems, muscle failure, diabetes and cancer are all caused by these free radicals. Antioxidants act like a broom against free radicals, destroying free radicals and regenerating damaged cells. Laboratory evidence has shown that antioxidants can prevent cancer [Citation22–25].
Now, turning our attention to investigate the bioactivity of A. noeanum leaf aqueous extract green-synthesised copper nanoparticles, a concentration-dependent DPPH radical scavenging effect of catalyst was observed against BHT as a reference.
DPPH process is widely applied to determine the free radical scavenging effect of different antioxidant materials. The DPPH scavenging abilities are known to be because of the hydrogen donating activities of antioxidant materials. When DPPH results are examined, it is observed that it has increased in a dose-dependent manner [Citation20,Citation21].
The interaction between A. noeanum leaf aqueous extract green-synthesised copper nanoparticles and DPPH might have occurred by transferring electrons and hydrogen ions [Citation22–35]. The scavenging capacity of the A. noeanum leaf aqueous extract green-synthesised copper nanoparticles and BHT at different concentrations, expressed in terms of percentage inhibition, has been shown in . The IC50 of A. noeanum leaf, butylated hydroxytoluene, and copper nanoparticles were 445, 241, and 160 µg/mL, respectively ().
Figure 7. The antioxidant properties of Cu(NO3)2, A. noeanum leaf aqueous extract, CuNPs, and BHT against DPPH.
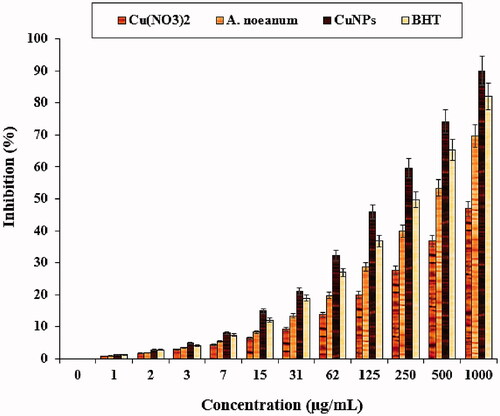
Table 1. The IC50 of Cu(NO3)2, A. noeanum leaf aqueous extract, CuNPs, and BHT in the antioxidant test.
Previous researches have indicated that flavonoids and phenolic compounds attached to the metallic nanoparticles have significant antioxidant properties [Citation21–24]. Previously it has been revealed that A. noeanum is full of antioxidant compounds such as Allicin[diallyl thiosulfinate], tuberoside M, S-allylmercaptocysteine, thiosulfinates, S-propargyl-L-cysteine, Ace-AMP1, quercetin, S-benzyl-cysteine, diosgenin, polysulfanes, diosgenin, fisetin, diallylpolysulfides, onionin A, diallylpolysulfides, Z-ajoene, allyl mercaptan, amino-styrene-acrylic acids, flavonoids, furostanol saponins, allyl sulphides, saponins, glycosides, diallyl tetrasulfide, garlic oil, S-Propargyl-cysteine, diallyl monosulfide, and Z-ajoene [Citation17]. Many studies were performed in the nanobiotechnology branch using several ethnomedicinal plants, but still, no study is present on A. noeanum leaf aqueous extract green-synthesised copper nanoparticles.
Study of the anticancer activity of copper nanoparticles in endometrial cancer in vitro
Among the different parameters of metallic nanoparticles such as size, texture and nature of surface functions, the size effect is most essential in the anticancer assay using standard cancer cell lines. Previous reports revealed that the anticancer activity increases with a decrease in particle size based on their better penetration ability over the cell lines. It has been surveyed that particle size lower than 50 nm displays better activity in the corresponding cancer cell lines [Citation8,Citation24–31]. In this study, the cytotoxicity of copper nanoparticles was explored by studying its interaction with HUVEC normal cell line, Ishikawa, HEC-1-A, HEC-1-B, and KLE cancer cell lines by MTT assay for 48 h. The interactions being expressed as cell viability (%) were observed at different Cu(NO3)2⋅2H2O, A. noeanum leaf aqueous extract, and copper nanoparticles concentrations (0–1000 μg/mL) with the three cell lines which have been shown in . In all the cases, the percentage of cell viability is reduced with increasing Cu(NO3)2, A. noeanum leaf aqueous extract, and copper nanoparticle concentrations. The IC50 of A. noeanum leaf aqueous extract and copper nanoparticles against HEC-1-B cell line were 548 and 331 µg/mL, respectively; against HEC-1-A cell line were 583 and 356 µg/mL, respectively; against KLE cell line were 609 and 411 µg/mL, respectively; and against Ishikawa cell line were 560 and 357 µg/mL, respectively (). Thereby, the best cytotoxicity results and anti-human endometrial cancer potentials of our copper nanoparticles was observed in the case of the HEC-1-B cell line.
Figure 8. The cytotoxicity properties of Cu(NO3)2, A. noeanum leaf aqueous extract, and CuNPs against the HUVEC cell line.
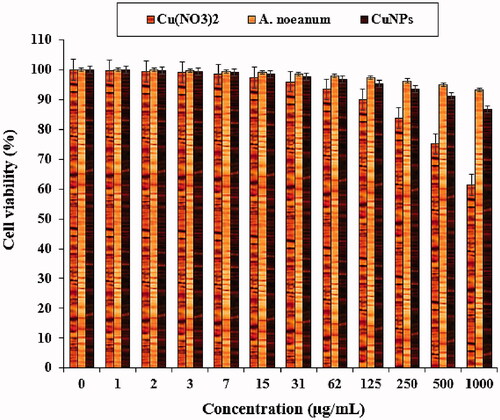
Figure 9. The anti-human endometrial cancer properties of Cu(NO3)2, A. noeanum leaf aqueous extract, and CuNPs against the HEC-1-B cell line.
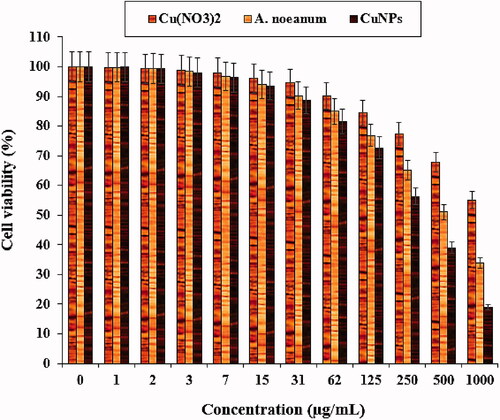
Figure 10. The anti-human endometrial cancer properties of Cu(NO3)2, A. noeanum leaf aqueous extract, and CuNPs against the HEC-1-A cell line.
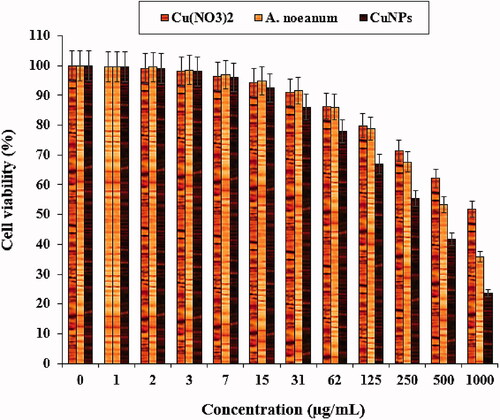
Figure 11. The anti-human endometrial cancer properties of Cu(NO3)2, A. noeanum leaf aqueous extract, and CuNPs against the KLE cell line.
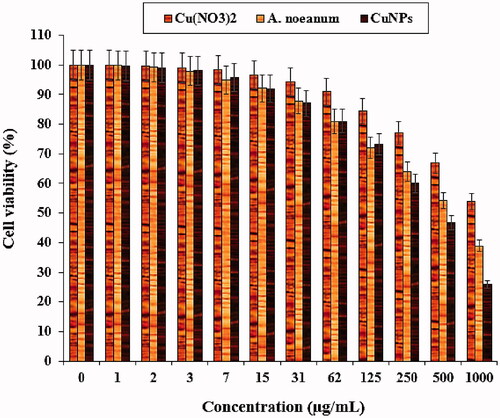
Figure 12. The anti-human endometrial cancer properties of Cu(NO3)2, A. noeanum leaf aqueous extract, and CuNPs against the Ishikawa cell line.
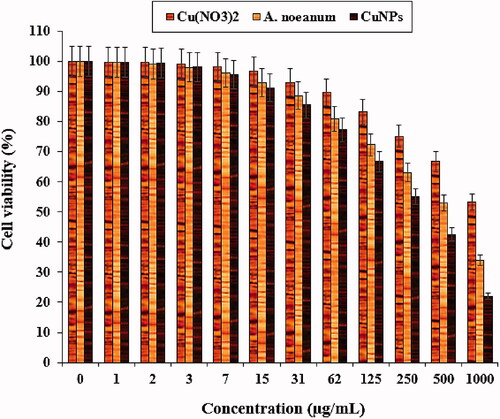
Table 2. The IC50 of Cu(NO3)2, A. noeanum leaf aqueous extract, and CuNPs in cytotoxicity test.
The anticancer effects of medicinal plants green-synthesised copper nanoparticles have been confirmed in the previous studies [Citation8,Citation24–31]. Ramaswamy et al. (2010) indicated when CuSO4 was combined with Sargassum polycystum brown seaweed, an anticancer supplement with significant effects against MCF-7 breast cancer cells was made [Citation24]. Also, Sumathi et al. [Citation27] and Roberson et al. [Citation28] mentioned notable anticancer properties of copper nanoparticles containing Prosopis cineraria against human MCF-7 and HeLa cancer cells. In other studies, the anticancer properties of copper nanoparticles green-synthesised by Nerium oleander [Citation29], Magnolia Kobus [Citation30], and Eclipta prostrata [Citation31] were confirmed against the human HepG2 cancer cell line.
Likely the significant anti-human endometrial cancer potentials of copper nanoparticles synthesised by A. noeanum leaf aqueous extract against human endometrial cancer cell lines are linked to their antioxidant effects. Similar reports have indicated the antioxidant materials such as metallic nanoparticles especially copper nanoparticles and ethno-medicinal plants reduce the volume of tumours by removing free radicals [Citation32]. In detail, the high presence of free radicals in the normal cells make many mutations in their DNA and RNA, destroy their gene expression and then accelerate the proliferation and growth of abnormal cells or cancerous cells [Citation30,Citation33]. The free radicals high presences in all cancers such as breast, gallbladder, stomach, rectal, liver, gastrointestinal stromal, oesophageal, bile duct, small intestine, pancreatic, colon, parathyroid, thyroid, bladder, prostate, testicular, fallopian tube, vaginal, ovarian, hypopharyngeal, throat, lung, and skin cancers indicate a significant role of these molecules in making angiogenesis and tumorigenesis [Citation33,Citation34]. Many researchers reported that copper nanoparticles synthesised by ethno-medicinal plants have a remarkable role in removing free radicals and growth inhibition of all cancerous cells [Citation34,Citation35].
Conclusion
In conclusion, a simple, straightforward and eco-friendly approach for the synthesis of well-dispersed nano Cu particles was reported. In the process, A. noeanum leaf aqueous extract was used as both a reducing and stabilising agent without the addition of any other reducing and capping agent. The analytical techniques, i.e. FT‐IR, FE‐SEM, UV-Vis, TEM, XRD, and EDX revealed that these nanoparticles had been formulated as soon as and as the best possible. The particles were highly crystalline in nature, spherical in shape and small in size with a narrow distribution from 10 nm to 15 nm. The copper nanoparticles were capped with extracts, preventing them from oxidation and agglomeration. In the UV-Vis, the sharp peak in the 585 nm wavelength demonstrated the Cu nanoparticles formation. The Cu nanoparticles revealed significant antioxidant activities against DPPH as a common free radical. The recent nanoparticles had effective anti-human endometrial cancer effects against Ishikawa, HEC-1-A, HEC-1-B, and KLE cell lines. The IC50 of copper nanoparticles were 331, 356, 411, and 357 µg/mL against HEC-1-B, HEC-1-A, KLE, and Ishikawa cell lines, respectively. Due to the significant results gained in the in vitro condition, it is recommended that the studies of the clinical trials are performed for proving these findings in humans.
Disclosure statement
No potential conflict of interest was reported by the author(s).
Data availability statement
The data that support the findings of this study are available from the corresponding author upon reasonable request.
Additional information
Funding
References
- Clarke MA, Long BJ, Morillo ADM, et al. Association of endometrial cancer risk with postmenopausal bleeding in women: a systematic review and meta-analysis. JAMA Intern Med. 2018;178(9):1210–1222.
- Burke WM, Orr J, Leitao M, et al. Endometrial cancer: a review and current management strategies: part I. Gynecol Oncol. 2014;134(2):385–392.
- Murali R, Soslow RA, Weigelt B, et al. Classification of endometrial carcinoma: more than two types. Lancet-Oncol. 2014;15:268–278.
- (a) Khatua A, Prasad A, Priyadarshini E, et al. Emerging antineoplastic plant-based gold nanoparticle synthesis: a mechanistic exploration of their anticancer activity toward cervical cancer cells. J Clust Sci. 2020;31(6):1329–1340. (b) Virmani I, Sasi C, Priyadarshini E, et al. Comparative anticancer potential of biologically and chemically synthesized gold nanoparticles. J Clust Sci. 2020;31(4):867–876.(c) Barabadi H, Vahidi H, Damavandi Kamali K, et al. Emerging theranostic gold nanomaterials to combat colorectal cancer: a systematic review. J Clust Sci. 2020;31(4):651–658. (d) Barabadi H, Vahidi H, Damavandi Kamali K, et al. Emerging theranostic gold nanomaterials to combat lung cancer: a systematic review. J Clust Sci. 2020;31(2):323–330.
- (a) Hamelian M, Zangeneh MM, Amisama A, et al. Green synthesis of silver nanoparticles using Thymus kotschyanus extract and evaluation of their antioxidant, antibacterial and cytotoxic effects. Appl Organometal Chem. 2018;32(9):e4458. (b) Hemmati S, Rashtiani A, Zangeneh MM, et al. Green synthesis and characterization of silver nanoparticles using Fritillaria flower extract and their antibacterial activity against some human pathogens. Polyhedron. 2019;158:8–14. (c) Hamelian M, Zangeneh MM, Shahmohammadi A, et al. Pistacia atlantica leaf extract mediated synthesis of silver nanoparticles and their antioxidant, cytotoxicity, and antibacterial effects under in vitro condition. Appl Organometal Chem. 2019;33:e5278.(d) Shahriari M, Hemmati S, Zangeneh A, et al. Biosynthesis of gold nanoparticles using Allium noeanum Reut. ex Regel leaves aqueous extract; characterization and analysis of their cytotoxicity, antioxidant, and antibacterial properties. Appl Organometal Chem. 2019;33(11):e5189. (e) Zangeneh MM, Saneei S, Zangeneh A, et al. Preparation, characterization, and evaluation of cytotoxicity, antioxidant, cutaneous wound healing, antibacterial, and antifungal effects of gold nanoparticles using the aqueous extract of Falcaria vulgaris leaves. Appl. Organometal. Chem. 2019;33:e5216. (f) Hemmati S, Heravi MM, Karmakar B, et al. Green fabrication of reduced graphene oxide decorated with Ag nanoparticles (rGO/Ag NPs) nanocomposite: a reusable catalyst for the degradation of environmental pollutants in aqueous medium. J Mol Liquid. 2020;319:114302.(g) Zangeneh MM. Green synthesis and formulation a modern chemotherapeutic drug of Spinacia oleracea L. leaf aqueous extract conjugated silver nanoparticles; chemical characterization and analysis of their cytotoxicity, antioxidant, and anti-acute myeloid leukemia properties in comparison to doxorubicin in a leukemic mouse model. Appl Organometal Chem. 2020;34:e5295. (h) Veisi H, Azizi S, Mohammadi P. Green synthesis of the silver nanoparticles mediated by Thymbra spicata extract and its application as a heterogeneous and recyclable nanocatalyst for catalytic reduction of a variety of dyes in water. J Cleaner Produc. 2018;170:1536–1543. (i) Lebaschi S, Hekmati M, Veisi H. Green synthesis of palladium nanoparticles mediated by black tea leaves (Camellia sinensis) extract: catalytic activity in the reduction of 4-nitrophenol and Suzuki-Miyaura coupling reaction under ligand-free conditions. J Colloid Interface Sci. 2017;485:223–231.
- (a) Veisi H, Hemmati S, Safarimehr P. In situ immobilized palladium nanoparticles on surface of poly-methyldopa coated-magnetic nanoparticles (Fe3O4@PMDA/Pd): a magnetically recyclable nanocatalyst for cyanation of aryl halides with K4 [Fe (CN) 6]. J Catal. 2018;365:204–212. (b) Veisi H, Hemmati S, Shirvani H, et al. Green synthesis and characterization of monodispersed silver nanoparticles obtained using oak fruit bark extract and their antibacterial activity. Appl Organometal Chem. 2016;30(6):387–391. (c) Veisi H, Kazemi S, Mohammadi P, et al. Catalytic reduction of 4-nitrophenol over Ag nanoparticles immobilized on Stachys lavandulifolia extract-modified multi walled carbon nanotubes. Polyhedron. 2019;157:232–240. (d) Veisi H, Karmakar B, Tamoradi T, et al. Biosynthesis of CuO nanoparticles using aqueous extract of herbal tea (Stachys lavandulifolia) flowers and evaluation of its catalytic activity. Sci Rep. 2021;11(1):1–13. (e) Veisi H, Karmakar B, Tamoradi T, et al. Bio-inspired synthesis of palladium nanoparticles fabricated magnetic Fe3O4 nanocomposite over Fritillaria imperialis flower extract as an efficient recyclable catalyst for the reduction of nitroarenes. Sci Rep. 2021;11(1):1–15. (f) Veisi H, Mohammadi L, Hemmati S, et. al. In situ immobilized silver nanoparticles on Rubia tinctorum extract-coated ultrasmall iron oxide nanoparticles: an efficient nanocatalyst with magnetic recyclability for synthesis of propargylamines by A3 coupling reaction. ACS Omega. 2019;4(9):13991–14003.
- (a) Ali K, Ahmed B, Ansari SM, et al. Comparative in situ ROS mediated killing of bacteria with bulk analogue, Eucalyptus leaf extract (ELE)-capped and bare surface copper oxide nanoparticles. Mat Sci Eng C. 2019;100:747–758. (b) Rajput V, Minkin T, Ahmed B, et al. Interaction of copper-based nanoparticles to soil, terrestrial, and aquatic systems: critical review of the state of the science and future perspectives. Rev Environ Contam Toxicol. 2019;252:51–96. (c) Saleem S, Ahmed B, Saghir Khan M, et al. Inhibition of growth and biofilm formation of clinical bacterial isolates by NiO nanoparticles synthesized from Eucalyptus globulus plants. Microb Pathog. 2017;111:375–387. (d) Ahmed B, Hashmi A, Saghir Khan M, et al. ROS mediated destruction of cell membrane, growth and biofilms of human bacterial pathogens by stable metallic AgNPs functionalized from bell pepper extract and quercetin. Adv Powder Tech. 2018;29(7):1601–1616. (e) Ali K, Ahmed B, Saghir Khan M, et al. Differential surface contact killing of pristine and low EPS Pseudomonas aeruginosa with Aloe vera capped hematite (α-Fe2O3) nanoparticles. J Photochem Photobiol B. 2018;188:146–158. (f) Haroon M, Zaidi A, Ahmed B, et al. Effective inhibition of phytopathogenic microbes by eco-friendly leaf extract mediated silver nanoparticles (AgNPs). Indian J Microbiol. 2019;59(3):273–287. (g) Ahmed B, Ameen F, Rizvi A, et al. Destruction of cell topography, morphology, membrane, inhibition of respiration, biofilm formation, and bioactive molecule production by nanoparticles of Ag, ZnO, CuO, TiO2, and Al2O3 toward beneficial soil bacteria. ACS Omega. 2020;5(14):7861–7876. (h) Ali K, Saquib Q, Ahmed B, et al. Bio-functionalized CuO nanoparticles induced apoptotic activities in human breast carcinoma cells and toxicity against Aspergillus flavus: an in vitro approach. Proc Biochem. 2020;91:387–397. (j) Tahvilian R, Zangeneh MM, Falahi H, et al. Green synthesis and chemical characterization of copper nanoparticles using Allium saralicum leaves and assessment of their cytotoxicity, antioxidant, antimicrobial, and cutaneous wound healing properties. Appl Organometal Chem. 2019;33(12):e5234.
- Zangeneh MM, Ghaneialvar H, Akbaribazm M, et al. Novel synthesis of Falcaria vulgaris leaf extract conjugated copper nanoparticles with potent cytotoxicity, antioxidant, antifungal, antibacterial, and cutaneous wound healing activities under in vitro and in vivo condition. J Photochem Photobiol B. 2019;197:111556. (b) Hassan SE-D, Salem SS, Fouda A, et al. New approach for antimicrobial activity and bio-control of various pathogens by biosynthesized copper nanoparticles using endophytic actinomycetes. J Radiat Res Appl Sci. 2018;11(3):262–270. (c) Hassan SE, Fouda A, Radwan AA, et al. Endophytic actinomycetes Streptomyces spp mediated biosynthesis of copper oxide nanoparticles as a promising tool for biotechnological applications. J Biol Inorg Chem. 2019;24(3):377–393. (d) Shaheen TI, Amr F, Salem SS. Integration of cotton fabrics with biosynthesized CuO Nanoparticles for bactericidal activity in the terms of their cytotoxicity assessment. Ind Eng Chem Res. 2021;60(4):1553–1563.
- (a) Nasrollahzadeh M, Sajadi M, Atarod M, et al. An introduction to green nanotechnology. Vol. 28. Interface science and technology. Elsevier; 2019; p. 1–337. (b) Nasrollahzadeh M, Sajadi M, Sajadi SM. Biosynthesis of copper nanoparticles supported on manganese dioxide nanoparticles using Centella asiatica L. leaf extract for the efficient catalytic reduction of organic dyes and nitroarenes. Chin J Catal. 2018;39(1):109–117. (c) Nasrollahzadeh M, Sajjadi M, Dadashi J, et al. Pd-based nanoparticles: plant-assisted biosynthesis, characterization, mechanism, stability, catalytic and antimicrobial activities. Adv Colloid Interface Sci. 2020;276:102103. (d) Nasrollahzadeh M, Sajadi M, Dasmeh HR, et al. Green synthesis of the Cu/sodium borosilicate nanocomposite and investigation of its catalytic activity. J Alloys Comp. 2018;763:1024–1034. (e) Khodadadi B, Bordbar M, Nasrollahzadeh M. Achillea millefolium L. extract mediated green synthesis of waste peach kernel shell supported silver nanoparticles: application of the nanoparticles for catalytic reduction of a variety of dyes in water. J Colloid Interface Sci. 2017;493:85–93. (f) Nasrollahzadeh M, Sajadi M, Rostami-Vartooni A. Green synthesis of CuO nanoparticles by aqueous extract of Anthemis nobilis flowers and their catalytic activity for the A3 coupling reaction. J. Colloid Interface Sci. 2015;459:183–188. (g) Nasrollahzadeh M, Sajadi M. Preparation of Pd/Fe3O4 nanoparticles by use of Euphorbia stracheyi Boiss root extract: a magnetically recoverable catalyst for one-pot reductive amination of aldehydes at room temperature. J Colloid Interface Sci. 2016;464:147–152. (h) Nasrollahzadeh M, Sajadi M. Green synthesis of Pd nanoparticles mediated by Euphorbia thymifolia L. leaf extract: catalytic activity for cyanation of aryl iodides under ligand-free conditions. J Colloid Interface Sci. 2016;469:191–195. (i) Nasrollahzadeh M, Sajadi M. Preparation of Au nanoparticles by Anthemis xylopoda flowers aqueous extract and their application for alkyne/aldehyde/amine A3-type coupling reactions. RSC Adv. 2015;5(57):46240–46246. (j) Naghdi S, Sajjadi M, Nasrollahzadeh M, et al. Cuscuta reflexa leaf extract mediated green synthesis of the Cu nanoparticles on graphene oxide/manganese dioxide nanocomposite and its catalytic activity toward reduction of nitroarenes and organic dyes. J Taiwan Inst Chem Eng. 2018;86:158–173. (k) Nasrollahzadeh M, Sajadi M. Synthesis and characterization of titanium dioxide nanoparticles using Euphorbia heteradena Jaub root extract and evaluation of their stability. Ceram Int . 2015;41(10):14435–14439.
- (a) Kharissova OV, Dias HVR, Kharisov BI, et al. The greener synthesis of nanoparticles. Trends Biotechnol. 2013;31(4):240–248. (b) Narayanan S, Sathy BN, Mony U, et al. Biocompatible magnetite/gold nanohybrid contrast agents via green chemistry for MRI and CT bioimaging. ACS Appl Mater Interfaces. 2012;4(1):251–260. (c) Ramesh C, Kumar KTM, Latha N, et al. Green synthesis of Cr2O3 nanoparticles using Tridax procumbens leaf extract and its antibacterial activity on Escherichia coli. CNANO. 2012;8(4):603–607. (d) Basavegowda N, Mishra K, Lee YR. Sonochemically synthesized ferromagnetic Fe3O4 nanoparticles as a recyclable catalyst for the preparation of pyrrolo [3,4-c] quinolone-1,3-dione derivatives. RSC Adv. 2014;4(106):61660–61666. (e) Latha N, Gowri M. Biosynthesis and characterization of Fe3O4 nanoparticles using Caricaya papaya leaves extract. Int J Sci Res. 2014;3(11):1551–1556. (f) Venkateswarlu S, Subba Rao Y, Balaji T, et al. Biogenic synthesis of Fe3O4 magnetic nanoparticles using plantain peel extract. Mater Lett. 2013;100:241–244. (g) Mahdavi M, Namvar F, Ahmad M, et al. Green biosynthesis and characterization of magnetic iron oxide (Fe3O4) nanoparticles using seaweed (Sargassum muticum) aqueous extract. Molecules. 2013;18(5):5954–5964. (h) Mortezaee K, Najafi M, Samadian H, et al. Redox interactions and genotoxicity of metal-based nanoparticles: a comprehensive review. Chem Biol Interact. 2019;312:108814.(i) Adwin Jose P, Dhaveethu Raja J, Sankarganesh M, et al. Evaluation of antioxidant, DNA targeting, antimicrobial and cytotoxic studies of imine capped copper and nickel nanoparticles. J Photochem Photobiol B. 2018;178:143–151.
- (a) Pugazhendhi A, Kumar SS, Manikandan M, et al. Photocatalytic properties and antimicrobial efficacy of Fe doped CuO nanoparticles against the pathogenic bacteria and fungi. Microbial Pathogenesis. 2018;122:84–89. (b) Subbaiya R, Saravanan M, Priya AR, et al. Biomimetic synthesis of silver nanoparticles from Streptomyces atrovirens and their potential anticancer activity against human breast cancer cells. IET Nanobiotechnol. 2017;11(8):965–972. (c) Barabadi H, Kobarfard F, Vahidi H, et al. Biosynthesis and characterization of biogenic tellurium nanoparticles by using Penicillium chrysogenum PTCC 5031: A novel approach in gold biotechnology. Iranian J Pharma Res. 2018;17:87–97. (d) Barabadi H, Alizadeh A, Ovais M, et al. Efficacy of green nanoparticles against cancerous and normal cell lines: a systematic review and meta-analysis. IET Nanobiotechnol. 2018;12(4):377–391. (e) Barabadi H, Honary S, Ebrahimi P, et al. Optimization of myco-synthesized silver nanoparticles by response surface methodology employing Box-Behnken design. Inorg.Nano-Metal Chem. 2019;49(2):33–43. (f) Honary S, Barabadi H, Gharaei-Fathabad E, et al. Green synthesis of silver nanoparticles induced by the fungus Penicillium citrinum. Trop J Pharm Res. 2013;12:7–11. (g) Barabadi H, Alizadeh Z, Rahimi MT, et al. Nanobiotechnology as an emerging approach to combat malaria: A systematic review. Nanomed Nanotec Biol Med. 2019;18:221–233. (h) Honary S, Barabadi H, Ebrahimi P, et al. Development and optimization of biometal nanoparticles by using mathematical methodology: a microbial approach. JNanoR. 2015;30:106–115. (j) Barabadi H, Honary S, Mohammadi MA, et al. Green chemical synthesis of gold nanoparticles by using Penicillium aculeatum and their scolicidal activity against hydatid cyst protoscolices of Echinococcus granulosus. Environ Sci Poll Res. 2017;24:800–5810. (k) Barabadi H. Nanobiotechnology: a promising scope of gold biotechnology. Cell Mol Biol (Noisy-le-Grand). 2017;63(12):3–4. (l) Barabadi H, Honary S, Ebrahimi P, et al. Microbial mediated preparation, characterization and optimization of gold nanoparticles. Braz J Microbiol. 2014;45(4):1493–1501. (m) Kanagamani K, Muthukrishnan P, Shankar K, et al. Antimicrobial, cytotoxicity and photocatalytic degradation of norfloxacin using Kleinia grandiflora mediated silver nanoparticles. J Clust Sci. 2019;30(6):1415–1424. (n) Balachandar R, Gurumoorthy P, Karmegam N, et al. Plant-mediated synthesis, characterization and bactericidal potential of emerging silver nanoparticles using stem extract of Phyllanthus pinnatus: a recent advance in phytonanotechnology. J Clust Sci. 2019;30(6):1481–1488.
- (a) Saravanan M, Asmalash T, Gebrekidan A, et al. Nano-medicine as a newly emerging approach to combat human immunodeficiency virus (HIV). Pharm Nanotechnol. 2018;6(1):17–27. (b) Varadharaj V, Ramaswamy A, Sakthivel R, et al. Antidiabetic and antioxidant activity of green synthesized starch nanoparticles: an in vitro study. J Clust Sci. 2020;31(6):1257–1266. (c) Khatua A, Priyadarshini E, Rajamani P, et al. Phytosynthesis, characterization and fungicidal potential of emerging gold nanoparticles using Pongamia pinnata leave extract: a novel approach in nanoparticle synthesis. J Clust Sci. 2020;31(1):125–131. (d) Barabadi H, Tajani B, Moradi M, et al. Penicillium family as emerging nanofactory for biosynthesis of green nanomaterials: a journey into the world of microorganisms. J Clust Sci. 2019;30(4):843–856. (e) Boomi P, Gurumallesh GP, Palanisamy S, et al. Evaluation of antibacterial and anticancer potential of polyaniline-bimetal nanocomposites synthesized from chemical reduction method. J Clust Sci. 2019;30(3):715–726. (f) Barabadi H, Ovais M, Shinwari ZK, et al. Anti-cancer green bionanomaterials: present status and future prospects. Green Chem Lett Rev. 2017;10(4):285–314. (g) Rahimi MT, Ahmadpour E, Esboei BR, et al. Scolicidal activity of biosynthesized silver nanoparticles against Echinococcus granulosus protoscolices. Int J Surg. 2015;19:128–133. (h) El-Sayed IH, Huang X, El-Sayed MA. Selective laser photo-thermal therapy of epithelial carcinoma using anti-EGFR antibody conjugated gold nanoparticles. Cancer Lett. 2006;239(1):129–135.
- (a) Fouda A, Salem SS, Wassel AR, et al. Optimization of green biosynthesized visible light active CuO/ZnO nano-photocatalysts for the degradation of organic methylene blue dye. Heliyon. 2020;6(9):e04896.(b) Alsharif SM, Salem SS, Abdel-Rahman MA, et al. Multifunctional properties of spherical silver nanoparticles fabricated by different microbial taxa. Heliyon. 2020;6(5):e03943.(c) Fouda A, Hassan SED, Abdo AM, et al. Antimicrobial, antioxidant and larvicidal activities of spherical silver nanoparticles synthesized by endophytic Streptomyces spp. Biol Trace Elem Res. 2020;195(2):707–724. (d) Eid AM, Fouda A, Niedbała G, et al. Endophytic Streptomyces laurentii mediated green synthesis of Ag-NPs with antibacterial and anticancer properties for developing functional textile fabric properties. Antibiotics. 2020;9(10):641.(e) Salem SS, EL-Belely EF, Niedbała G, et al. Bactericidal and in-vitro cytotoxic efficacy of silver nanoparticles (Ag-NPs) fabricated by endophytic actinomycetes and their use as coating for the textile fabrics. Nanomaterials. 2020;10(10):2082.(f) Salem SS, Fouda A. Green synthesis of metallic nanoparticles and their prospective biotechnological applications: an overview. Biol Trace Elem Res. 2021;199(1):344–370. (g) Fouda A, Hassan SED, Saied E, et al. An eco-friendly approach to textile and tannery wastewater treatment using maghemite nanoparticles (γ-Fe2O3-NPs) fabricated by Penicillium expansum strain (K-w). J Environ Chem Eng. 2021;9(1):104693.(h) El-Belely EF, Farag MMS, Said HA, et al. Green synthesis of zinc oxide nanoparticles (ZnO-NPs) using Arthrospira platensis (Class: Cyanophyceae) and evaluation of their biomedical activities. Nanomaterials. 2021;11(1):95.
- Chatterjee AK, Sarkar RK, Chattopadhyay AP, et al. A simple robust method for synthesis of metallic copper nanoparticles of high antibacterial potency against E. coli. Nanotechnol. 2012;23(8):085103.
- Azizi M, Ghourchian H, Yazdian F, et al. Cytotoxic effect of albumin coated copper nanoparticle on human breast cancer cells of MDA-MB 231. PLoS One. 2017;12(11):e0188639.
- (a) Hagh-Nazari L, Goodarzi N, Zangeneh MM, et al. Stereological study of kidney in streptozotocin-induced diabetic mice treated with ethanolic extract of Stevia rebaudiana (bitter fraction). Comp Clin Pathol. 2017;26(2):455–463. (b) Ghashghaii A, Hashemnia M, Nikousefat Z, et al. Wound healing potential of methanolic extract of Scrophularia striata in rats. Pharm Sci. 2017;23(4):256–263. (c) Moradi R, Hajialiani M, Salmani S, et al. Effect of aqueous extract of Allium saralicum R.M. Fritsch on fatty liver induced by high-fat diet in Wistar rats. Comp Clin Pathol. 2019;28(5):1205–1211. (d) Sayyedrostami T, Pournaghi P, Vosta-Kalaee SE, et al. Evaluation of the wound healing activity of Chenopodium botrys leaves essential oil in rats (a short-term study). J Essent Oil Bear Plants. 2018;21(1):164–174. (e) Zhaleh M, Sohrabi N, Zangeneh MM, et al. Chemical composition and antibacterial effects of essential oil of Rhus coriaria fruits in the west of Iran (Kermanshah). J Essent Oil Bear Plants. 2018;21(2):493–501. (f) Sherkatolabbasieh H, Hagh-Nazari L, Shafiezadeh S, et al. Ameliorative effects of the ethanolic extract of Allium saralicum R.M. Fritsch on CCl4-induced nephrotoxicity in mice: a stereological examination. Arch Biol Sci (Beogr). 2017;69(3):535–543.
- Zeng Y, Li Y, Yang J, et al. Therapeutic role of functional components in Alliums for preventive chronic disease in human being. Evid Based Complement Alternat Med. 2017;2017:1–13.
- Hosseinimehr SJ, Mahmoudzadeh A, Ahmadi A, et al. The radioprotective effect of Zataria multiflora against genotoxicity induced by γ irradiation in human blood lymphocytes. Cancer Biother Radiopharm. 2011;26(3):325–329.
- Veisi H, Karmakar B, Tamoradi T, et al. Biosynthesis of CuO nanoparticles using aqueous extract of herbal tea (Stachys lavandulifolia) flowers and evaluation of its catalytic activity. Sci Rep. 2021;11:1–13.
- Reuter S, Gupta SC, Chaturvedi MM, et al. Oxidative stress, inflammation, and cancer: how are they linked? Biol Med. 2010;11:1603–1616.
- Gultekin DD, Alayli A, Önem H, et al. Synthesis of copper nanoparticles using a different method: determination of their antioxidant and antimicrobial activity. J Turk Chem Soc A Chem. 2016;3(3):623–636.
- Rehana D, Mahendiran D, Senthil Kumar R, et al. Evaluation of antioxidant and anticancer activity of copper oxide nanoparticles synthesized using medicinally important plant extracts. Biomed Pharmacother. 2017;89:1067–1077.
- Del Mar Delgado-Povedano M, Sánchez de Medin V, Bautista J, et al. Tentative identification of the composition of Agaricus bisporus aqueous enzymatic extracts with antiviral activity against HCV: a study by liquid chromatography–tandem mass spectrometry in high resolution mode. J Funct Foods. 2016;24:403–419.
- Jeong SC, Koyyalamudi SR, Jeong YT, et al. Macrophage immunomodulating and antitumor activities of polysaccharides isolated from Agaricus bisporus white button mushrooms. J Med Food. 2012;15(1):58–65.
- Wang YX, Tang XF, Yang ZG. A novel wet-chemical method of preparing highly monodispersed Cu2O nanoparticles. Colloids Sur A. 2011;388(1–3):38–40.
- (a) Barabadi H, Mahjoub MA, Tajani B, et al. Emerging theranostic biogenic silver nanomaterials for breast cancer: a systematic review. J Clust Sci. 2019;30(2):259–279. (b) Barabadi H, Damavandi Kamali K, Jazayeri Shoushtari F, et al. Emerging theranostic silver and gold nanomaterials to combat prostate cancer: a systematic review. J Clust Sci. 2019;30(6):1375–1382. (c) Barabadi H, Hosseini O, Damavandi Kamali K, et al. Emerging theranostic silver nanomaterials to combat lung cancer: a systematic review. J Clust Sci. 2020;31(1):1–10. (d) Barabadi H, Vahidi H, Damavandi Kamali K, et al. Emerging theranostic silver nanomaterials to combat colorectal cancer: a systematic review. J Clust Sci. 2020;31(2):311–321.
- Sumathi S, Dharani B, Sivaprabha J, et al. Cell death induced by methanolic extract of Prosopis cineraria leaves in MCF-7 breast cancer cell line. Inter J Pharma Sci Invent. 2013;2:21–26.
- Roberson M, Rangari V, Jeelani S, et al. Synthesis and characterization silver, zinc oxide and hybrid silver/zinc oxide nanoparticles for antimicrobial applications. Nano Life. 2014;04(01):1440003.
- Gopinath M, Rangari V, Jeelani S, et al. Synthesis of copper nanoparticles from Nerium oleander leaf aqueous extract and its antibacterial activity. Int J Curr Microbiol App Sci. 2014;3:814–818.
- Lee H, Song Y. Synthesis of copper nanoparticles using Eclipta prostrata leaves extract and their antioxidant and cytotoxic activities. Exp Ther Med. 2017;14:18–24.
- Lu Y, Wan X, Li L, Sun P, Liu G. Synthesis of a reusable composite of graphene and silver nanoparticles for catalytic reduction of 4- nitrophenol and performance as anti-colorectal carcinoma. Journal of Materials Research and Technology. 12 (2021) 1832–1843.
- Katata-Seru L, Moremedi T, Aremu OS, et al. Green synthesis of iron nanoparticles using Moringa oleifera extracts and their applications: removal of nitrate from water and antibacterial activity against Escherichia coli. J Mol Liq. 2018;256:296–304.
- Sangami S, Manu B. Synthesis of green iron nanoparticles using laterite and their application as a fenton-like catalyst for the degradation of herbicide Ametryn in water. Environ Technol Innov. 2017;8:150–163.
- Beheshtkhoo N, Jadidi Kouhbanani MA, Savardashtaki A, et al. Green synthesis of iron oxide nanoparticles by aqueous leaf extract of Daphne mezereum as a novel dye removing material. Appl Phys A. 2018;124:363–369.
- Radini IA, Hasan N, Malik MA, et al. Biosynthesis of iron nanoparticles using Trigonella foenum-graecum seed extract for photocatalytic methyl orange dye degradation and antibacterial applications. J Photochem Photobiol B. 2018;183:154–163.