Abstract
Silybum marianum L. commonly known as milk thistle is a medicinally potent plant with a multitude of pharmacological applications. The present investigations demonstrated the effects of Zinc Oxide nanoparticles (ZnO NPs) on callus growth and biosynthesis of silymarin in milk thistle under various light conditions. The callus cultures developed on Murashige and Skoog (MS) basal media containing ZnO NPs (0.15 mg/L), under the dark condition maintained for two weeks, followed by transference into normal light produced the maximum callus fresh weight (2294 mg/L FW). Further, the metabolite profiling revealed that ZnO NPs significantly augmented the production of silymarin and upregulated the antioxidant system in the callus cultures. Maximum TPC (total phenolic content: 37 ± 0.20 mg/g DW), TFC (total flavonoid content: 8.9 ± 0.023), DPPH antioxidant activity (91.5 ± 1.75%), Superoxide dismutase activity (SOD: 4.1 ± 0.045 nM/min/mg FW) and the highest silymarin content (14.6 ± 0.023 mg/g DW) were recorded in the callus cultures developed on MS media supplemented with solitary ZnO NPs (0.15 mg/L). While the callus culture evolved in presence of only PGRs (2,4 D and BA: 2 mg/L, each) accumulated the lesser fresh weight (562 mg/L FW). A higher concentration of ZnO NPs (0.15 mg/L) enhanced the secondary metabolite accumulation and silymarin content in the callus of Silybum marianum. This is the first standardized protocol to be applied on the industrial level for the production of silymarin.
Introduction
Nanotechnology has substantially impacted almost every field from basic to applied sciences. In the last decade, the emergence of different types of nanomaterials has revolutionized different walks of life with magnificent applications [Citation1]. Nanomaterials are fabricated materials of size in the range of 1–100 nm. At the nano size, these materials acquire some novel features in terms of faster activity due to the structural modifications that taken place at a molecular level. Engineered nanoparticles such as silver, gold, carbon, zinc, magnesium, copper, etc., with the size of ranging from 1–100 nm reported significant benefits in medicine, food, cosmetics, material sciences, and agricultural and environmental researches [Citation2–Citation4]. Amongst metallic nanoparticles (NPs), the unique catalytic properties of zinc oxide nanoparticles (ZnO NPs) have received greater attention worldwide for their diverse applications in metallurgy, engineering, medicine and agriculture [Citation5]. In-plant cell culture technology, ZnO NPs have shown differential effects on plant cell growth, physiological responses and secondary metabolism in many medicinal plants [Citation6]. Zn is an essential metal nutrient that plays a vital role in the healthy growth of plant cells through the provision of zinc ions at an optimal level in cell cultures. Since zinc deficiency in the culture media drastically damages the chlorophyll content and de-regulates the process of photosynthesis and thus can ultimately cause a reduction in plant growth. Therefore, ZnO NPs might be a suitable source that can release zinc ions at optimal levels in the growth media for efficient absorption and uptake by plant cells. These NPs have been reported to have the features of bio-safe elicitors for enhancing the synthesis of secondary metabolites and are non-toxic to plant cells during in vitro cultures [Citation7,Citation8]. Acting as elicitors ZnO NPs are involved in the activation of genes, different cell signalling and metabolic pathways during the growth and development of plants [Citation9]. After penetration into the plant cell, these NPs might trigger the accumulation of reactive oxygen species (ROS) that cause severe oxidative stress. In response to avoid the oxidative outburst, plant cell produces different secondary metabolites in higher quantities [Citation6].
Silybum marianum, also called milk thistle, cardus marianus, blessed thistle, is a vital medicinal plant that belongs to the family Asteraceae [Citation10]. It is an annual or biennial plant initially found in the Mediterranean region but is now found in many parts throughout the world. The phytochemical profile depicts the presence of a variety of bio-actives in S. maranum. The principal component is called silymarin which is a mixture of six isomeric flavonolignans including silydianin, silychristin, di-stereoisomers of silybin (silybin A & B), and di-stereoisomers of isosilybin (isosilybin A & B) [Citation11]. Silymarin is a potent medicine clinically prescribed for the treatment of hepatic disorders. It also has promising anti-carcinogenic effects, such as inhibition of human prostate cancer, breast cancer, cervical and skin cancer [Citation12]. It can help to protect human hepatic tissues by scavenging the free radicals and neutralizing the influence of oxidative damage [Citation13]. Silymarin extract has also been proposed to be more effective for the treatment of the obsessive-compulsive disorder (OCD), thalassaemia and non-alcoholic fatty liver disorder (NAFLD), diseases [Citation14]. Recent studies have emphasized on the potential use of silymarin as an anti-inflammatory and immunomodulatory agent by inhibiting the NF-B pathway [Citation15]. It has also been employed as an alternative source of medicine for the treatment of metabolic syndrome disease in humans and animals [Citation16].
S. marianum can efficiently grow in the autumn and spring season, at 2–15 °C temperature. It has the potential to grow under poor soil conditions however the minimal availability of nutrients in the soil can alter the quality and quantity of silymarin. Further, variation in different factors like geographic localities, environmental fluctuations and the absence of uniform cultivation methods affect the synthesis and activity of silymarin. The demand for silymarin has instantly increased in the last decade due to its diverse pharmacological applications. To meet the growing market demands, plant cell culture technology is an alluring and alternative approach for the production of healthy plant biomass enriched with quality metabolites under controlled conditions in a short period [Citation17,Citation18]. Plant in vitro cultures in certain circumstances may produce a higher amount of plant secondary metabolites, as compared with the wild counterparts in medicinal plants [Citation19]. Among different plant cell culture strategies, callus culture is a preferred strategy for the efficient and feasible production of healthy plant material with enhanced bioactive phytochemicals. Callus can also be used as a starting material for the in vitro regeneration of entire plantlets [Citation20,Citation21].
Light is the main factor affecting plant growth, development, and secondary metabolism [Citation22,Citation23]. For normal growth and development, every plant has its own best range of light intensities. The perceived signals from different light sources can directly control the gene expression of a plant cell that might lead to diverse physiological and metabolic responses and functions. However, developmental responses by plants are dependent on the relative exposure durations of cultures to different light intensities, and spectral composition [Citation17].
As described above, that ZnO NPs also possess unique catalytic activity, this property can be engineered by its application under the influence of different light intensities to initiate the phenomenon of photocatalysis for making these NPs more potent in their effects on plant cell growth and secondary metabolism in a plant as a biological model.
The main objectives of this research work were to investigate the interactive effects of ZnO NPs and different light treatments on the growth and accumulation of medicinal antioxidants and silymarin in the callus culture of S. marianum.
Materials and methods
In vitro seed germination
Seeds of S. marianum were collected from the National Agriculture research centre (NARC) Islamabad, Pakistan. All the seeds were first washed with tap water and were then surface sterilized by treating them with a mixture of a few drops of Tween 20 and Clorox 1.5% for 20 min. Then the seeds were washed three times with sterile distilled water and were then immersed in 0.05% mercuric chloride (HgCl2) solution for 10 min and then again washed with distilled water. The surface-sterilized seeds were incubated in test tubes containing growth media for germination.
The growth media contained iron source, MS basal salts, sucrose 30 g/L, vitamins, and agar 7 g/L with no addition of plant growth hormones. Before autoclaving the culture tubes at 121 °C for twenty min, the pH of the media was adjusted at 5.7–5.8. All the tubes were kept in a growth room under white light 16-h photoperiod at 25 ± 3 °C.
Callus cultures establishment
For the establishment of callus cultures, three different explants (root, hypocotyl and cotyledon) sections of size approximately 1 to 1.5 cm were cut out from four weeks old in vitro germinated seedlings. The excised explants were inoculated on MS medium supplemented with a combination of plant growth hormones 2,4-Dichlorophenoxyacetic acid (2,4-D) and 6-Benzylaminopurine(BA) added at various concentrations (1.1 mg/L, 2.2 mg/L and 3.3 mg/L), respectively. For each treatment, an explant was cultured on MS medium in a single test tube. Therefore, to obtain three replicate, the experiment was repeated thrice (1 explant × 3 culture tubes). Total 27 test tubes were subjected for each explant inoculation with various concentrations of the selected hormones. The data collection for callus culture was initiated after the 4th day of inoculation till the 35th day. Fifth weeks old calli were then sub-cultured on respective hormonal media composition for callus proliferation.
Effect of ZnO NPs and light treatments on callus proliferation and growth kinetics
Zinc oxide nanoparticles (ZnO NPs) used in this study were purchased from Sigma (Aldrich) with catalogue number 677450 packed as nanopowder, <50 nm. The protocol of Ali et al. [Citation24] was adopted for the preparation of a stock solution of ZnO NPs. Further, the prepared stock solution was filter sterilized using a micro filter under aseptic conditions.
Fifth weeks old Calli which were established on MS media in presence of 2,4-D and BA (2 mg/L, each) were used in subsequent experiments on checking the impacts of ZnO NPs under different light regimes on callus proliferation. ZnO NPs at varying levels (0.05 mg/L, 0.10 mg/L and 0.15 mg/L) were tested either alone or in combination with PGRs. For the control treatment, the MS media containing 2,4-D + BA (2 mg/L, each) was used. After inoculation, all the cultures were kept under various light intensity treatments such as normal light (2000–2500 l×), diffused light (500–1000 l×), complete dark, and shifting from two weeks’ dark incubation to normal light condition respectively. Philips tubes were used as the main source of lighting while the light intensities were calculated via Lux metre. Black plastic sheets were used to cover the culture tubes to maintain complete dark conditions. During all experiments, the photoperiod was set at 8 h’ dark and 16 h’ light under constant temperature (25 ± 20 °C).
Experiments were conducted in triplicates and the growth curve for the accumulation of callus biomass was drawn in response to ZnO NPs either alone or in combination with PGRs using different light treatments. Data was recorded weekly with 7 days of interval until the 8th week of culture time and data on the total amount of callus were recorded after 56 d to evaluate the rate of callus proliferation, callus texture, callus colour, fresh weight and dry weight. To get fresh weight, each callus was harvested from the respective treatment and was washed with distilled water. Sterilized filter paper was used to dry the callus and was then accurately weighed. All the calli were then subjected to oven drying by keeping them in an oven at 50 °C for one day, then the final dry weight was respectively calculated.
Metabolites extraction and qualitative determination of secondary metabolites
For analysis of the secondary metabolites in the callus cultures grown under the interactive effects of ZnO NPs and light treatments, dried powder of all the selected calli were thoroughly mixed with 99.9% methanol and vortexed for 5 min. Further, the sonication process was followed for half an hour at room temperature. Finally, the mixture was centrifuged at 15,000 rpm for 10 min and the obtained supernatant was taken and kept at −4 °C for analysis. The Folin-Ciocalteu procedure was followed to estimate the total phenolic content in the selected plant samples according to the method of Khan et al. [Citation13]. For the assay, we prepared a reaction mixture by taken 20 μl of methanol extracted sample, Folin-Ciocalteu reagent (90 u/L) and sodium carbonate (90 u/L) to make the final volume of the mixture up to 200 u/L. In this method; Gallic acid (1 mg/mL) was applied as a standard and methanol (20 μl) as a negative control. Data was noted as Gallic acid equivalent (GAE/g) and absorbance of the reaction mixture was taken at 630 nm after 90 min using a micro plate reader.
Likewise, the methodology of Chang et al. [Citation25] with slight modifications was employed to measure the total flavonoid content (TFC). Briefly, 20 μl of extracted samples from each treatment, aluminium chloride (10 μl), distilled water (160 ul) and potassium acetate (10 ul) were sequentially added in the reaction mixture to make 200 μl final volume. The Quercitin was used as standard and methanol as a negative control respectively. Data were expressed as quercetin equivalent (QAE/g) per gram of dry weight before noting the absorbance of the reaction mixture at 450 nm, the reaction mixture was incubated for thirty min.
Phenylalanine ammonia-lyase (PAL) activity was determined by the cinnamic acid method developed by Syklowska-Baranek et al. [Citation26]. Initially, Fresh callus (50 mg) was homogenized in 0.8 mM Mercaptoethanol, 0.05 M Tris-HCl buffer and 1% (w/v) PVP under a control PH (8). Thereafter, the solution was filtered and centrifuged at 4 °C for 15 min at 14000 g and supernatant was collected for further assay. The solution used to determine PAL activity contained 1 ml tris-HCL buffer, 0.5 ml/L Phe (10 mM) and 0.1 ml enzymatic extract. After an hour 0.1 ml HCL was used to stop the reaction, after incubating the solution at 37 °C and at 290 nm absorbance was measured. The methodology of Ali et al. [Citation24] was followed for the determination of DPPH free radical scavenging activity. Nonetheless, the methodology of Kazmi et al. [Citation27] was used to examine the superoxide dismutase activity (SOD) in the selected plant samples.
High performance liquid chromatography (HPLC) analysis of silymarin
The methodology of Khan et al. [Citation17] was followed for the quantification of silymarin in the in vitro raised callus cultures. Briefly, each dried callus sample (2 g) was crushed and then sonicated in the mixture by using a 30 V: 70 V ratio of phosphoric acid (0.1%) and methanol for 30 min. The Shimadzu Lc8A system was utilized for HPLC analysis. For the assay, C18 (ODS) column of particle size (150 × 4.6 mm) and the chromatographic eluents comprising ultrapure water with H3PO4 (0.1%) in Pump A and C2H3N in pump B were subjected. For making silymarin gradient elution, the quantity of elution flow was adjusted as 1.0 ml/min with 10 µl of injection volume. Sigma (CA, USA) standard of silymarin was used for comparison of the analyzed samples on the basis of peak areas and retention times. The quantification of silymarin content was calculated in mg/g (DW).
Statistical analysis
The experiments were performed in a complete randomized design. The experiments were conducted in a triplet manner; each treatment consisted of three test tubes. All results of the experiments were calculated as mean values and standard deviation. The data were analyzed statistically to find out the variances by one-way ANOVA using SPSS ver. 16.0 software. The mean values and significant differences were calculated using DMRT at p < .05.
Results
Optimization for screening of suitable explant type and plant growth regulators (PGRs) in callus induction
In the initial experiments of this study, seeds of S. marianum were in vitro germinated on MS0 media (MS media with no PGRs) to obtain sterile explants. Maximum seed germination frequency (75%) was recorded after 4 weeks of incubation of seeds in culture tubes and healthy plantlets were developed. For the establishment of callus cultures, three different explants were excised from the in vitro germinated seedlings, including hypocotyl, root and cotyledon sections, those were inoculated on MS media supplement with 2.4-D and BA at concentrations of (1.1 mg/L, 2.2 mg/L and 3.3 mg/L). Among all the explants and PGRs combinations tested, maximum callus induction frequency (90%) was recorded for root explants grown on MS media supplement with 2.4-D and BA (2 mg/L; each) (). This treatment promoted the earliest callus induction (in 4 days following the culture initiation) and production of maximum callus biomass (1.3 g). This was followed by cotyledon explants (60%) on the same PGRs treatment. The calluses were observed to have a light brownish colour and fragile texture. However, the addition of the same PGRs at a higher level (3.0 mg/L) in MS media resulted in lower growth parameters in callus formation by contemplating late callus initiation (11 d), lowest percent response (20%) and minimum callus biomass (0.5 g) ().
Figure 1. Effects of different explants with different plant growth regulators on callus induction frequency. Data represent the mean values of triplicates with ± standard error for each treatment in three repeated experiments. Annotation of columns data with different alphabet (s) represents the significance at (p = .05).
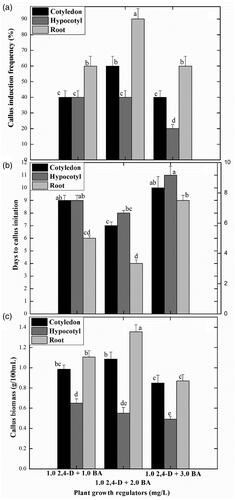
Impact of ZnO NPs on callus growth dynamics under different light regimes
The combined application of ZnO NPs and light treatments resulted in differential growth responses in callus morphology and biomass accumulation. Callus proliferation was observed in response to all the applied treatments. We observed a substantial increase in callus proliferation (FW: 2294 mg) when the cultures were grown over media containing 0.15 mg/L ZnO NPs, placed under dark for two weeks followed by transference to normal light (.
Figure 2. Effects of different levels of Zinc oxide nanoparticles and light regimes on callus biomass formation. Data represent the mean values of triplicates with ± standard error for each treatment in three repeated experiments. Annotation of columns data with different alphabet (s) represents the significance at (p = .05).
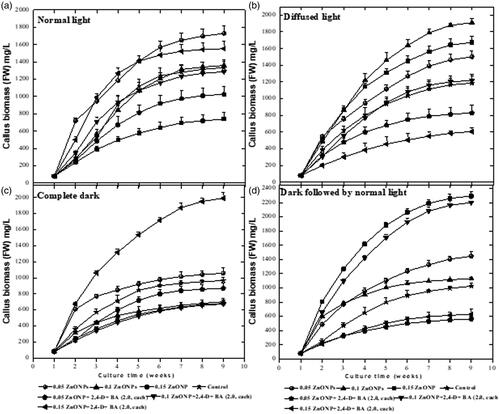
In response to normal light treatment, the highest callus biomass (1729 mg) was produced on the MS media augmented with solitary 0.05 mg/L of ZnO NPs, while at higher concentration of ZnO NPs (i.e. 0.15 mg/L) lowest callus growth (740 mg) and proliferation were observed (). Similarly, under diffused light conditions, MS media supplemented with ZnO NPs (0.10 mg/L) produced maximum callus biomass (1910 mg). The media containing a combination of ZnO NPs (0.05 mg/L) and PGRs (2,4 D + BA at 2 mg/L; each) significantly reduced the callus biomass formation (606 mg) under diffused light. Whereas, the same media under complete dark treatment produced the highest callus biomass (1920 mg).
Interestingly, ZnO NPs when employed at 0.15 mg/L under dark treatment for two weeks, the highest fresh weight of callus (2294 mg) was recorded in the cultures (, ). In the context of callus characteristics such as colour and texture, it was observed that ZnO NPs alone in culture media when treated with different light intensities produced yellowish and fragile callus. However, calli raised on growth media containing ZnO NPs along with PGRs incubated under all light treatments resulted in light brownish or dark brownish compact calluses ().
Figure 3. Interactive effects of ZnO NPs alone or in combination with PGRs under different light regimes on callus biomass formation in S. marianum. A1–A7= Normal light. A1: 0.05 mg/L ZnO NPs, A2: 0.1 mg/L ZnO NPs, A3: 0.15 mg/L ZnO NPs, A4: 2 mg/L BA + 2 mg/L 2,4-D, A5: 0.05 mg/L ZnO NPs+ 2 mg/L BA + 2 mg/L 2,4-D, A6: 0.1 mg/L ZnO NPs+ 2 mg/L BA + 2 mg/L 2,4-D, A5: 0.15 mg/L ZnO NPs+ 2 mg/L BA + 2 mg/L 2,4-D. A8-A14= Diffuse light. A8: 0.05 mg/L ZnO NPs, A9: 0.1 mg/L ZnO NPs, A10: 0.15 mg/L ZnO NPs, A11: 2 mg/L BA + 2 mg/L 2,4-D, A12: 0.05 mg/L ZnO NPs+ 2 mg/L BA + 2 mg/L 2,4-D, A13: 0.1 mg/L ZnO NPs+ 2 mg/L BA + 2 mg/L 2,4-D, A14: 0.15 mg/L ZnO NPs+ 2 mg/L BA + 2 mg/L 2,4-D. A15-A21= Complete dark. A15: 0.05 mg/L ZnO NPs, A16: 0.1 mg/L ZnO NPs, A17: 0.15 mg/L ZnO NPs, A18: 2 mg/L BA + 2 mg/L 2,4-D, A19: 0.05 mg/L ZnO NPs+ 2 mg/L BA + 2 mg/L 2,4-D, A20: 0.1 mg/L ZnO NPs+ 2 mg/L BA + 2 mg/L 2,4-D, A21: 0.15 mg/L ZnO NPs+ 2 mg/L BA + 2 mg/L 2,4-D. A22-A28= Complete dark followed by normal light. A22: 0.05 mg/L ZnO NPs, A23: 0.1 mg/L ZnO NPs, A24: 0.15 mg/L ZnO NPs, A25: 2 mg/L BA + 2 mg/L 2,4-D, A26: 0.05 mg/L ZnO NPs+ 2 mg/L BA + 2 mg/L 2,4-D, A27: 0.1 mg/L ZnO NPs+ 2 mg/L BA + 2 mg/L 2,4-D, A28: 0.15 mg/L ZnO NPs+ 2 mg/L BA + 2 mg/L 2,4-D.
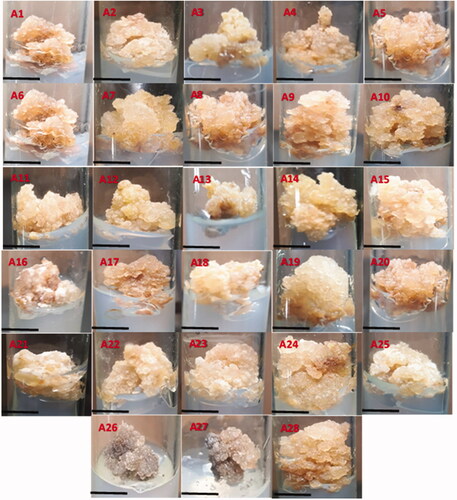
Table 1. Interactive effect of ZnO NPs and light treatments on callus morphology of S. marianum.
Impact of ZnO NPs on callus secondary metabolism under different light regimes
In this study, the interaction of ZnO NPs at different levels with the various light intensities profoundly affected the expression of TPC, TFC, PAL and DPPH free radical scavenging activities, respectively in the callus cultures. Exposure of calli to ZnO NPs at 0.15 mg/L alone and keeping initially in dark condition for two weeks followed by shifting to normal light exhibited maximum TPC (37 mg/g), TFC (8.9 mg/g), and DPPH antioxidant activity (91%), followed by complete dark and then diffused light. However, at normal light conditions lower expression of these biochemical markers was observed in callus cultures grown at 0.15 mg/L ZnO NPs (). Under normal light condition highest TPC (26 ± 0.012 mg/g DW) and TFC (3.9 ± 0.023 mg/g DW) were recorded in the calluses proliferated on MS media augmented with ZnO NPs (0.15 mg/L) only, while the cultures developed on MS media supplemented with both ZnO NPs (0.15 mg/L) and PGRs (2,4-D and BA; 2 mg/L, each) under the same light treatment produced lesser quantities of TPC (18 ± 0.01 mg/g DW) and TFC (2.5 ± 0.024 mg/g DW). In response to diffused light, the callus cultures were grown in presence of ZnO NPs (0.15 mg/L) and PGRs, accumulated maximum TPC and TFC when compared with other NPS and PGRs doses ().
Figure 4. Effects of different levels of Zinc oxide nanoparticles and light regimes on secondary metabolites production in callus cultures. Data represent the mean values of triplicates with ± standard error for each treatment in three repeated experiments. Annotation of columns data with different alphabet (s) represents the significance at (p = .05).
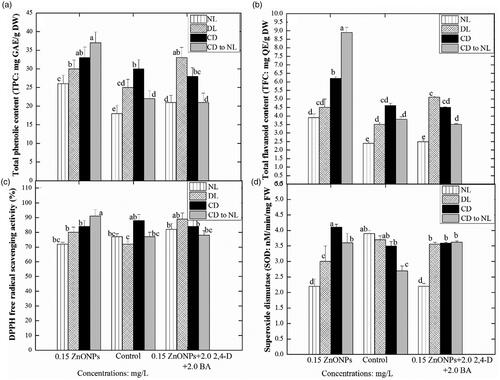
In complete dark condition maximum TPC (33 ± 0.024 mg/g DW) and TFC (6.2 ± 0.10 mg/g DW) were recorded in ZnO NPs (0.15 mg/L) mediated callus cultures. Whereas the minimum TPC (28 ± 0.021 mg/g DW) and TFC (4.5 ± 0.025 mg/g DW) were recorded in the callus cultures supplemented with ZnO NPs in combination with PGRs under dark treatment (. In this study, the phenyl ammonia-lyase (PAL) activity and DPPH free radical scavenging activity of the proliferated callus cultures developed either on ZnO NPs (0.15 mg/L) alone or in combination with PGRs (2,4-D and BA: 2 mg/L, each) under various light treatments were recorded with different patterns and trends (). Callus cultures treated with the combination of ZnO NPs and PGRs under normal light conditions, accumulated maximum PAL activity (9.1 mg/g DW) (). Callus cultures were grown under a normal light condition in PGRs (2,4-D and BA: 2 mg/L, each) containing media, accumulated maximum PAL activity (9.1 mg/gDW).
Figure 5. Effects of different levels of Zinc oxide nanoparticles and light regimes on the production of pal activity and silymarin content in callus cultures. Data represent the mean values of triplicates with ± standard error for each treatment in three repeated experiments. Annotation of columns data with different alphabet (s) represents the significance at (p = .05).
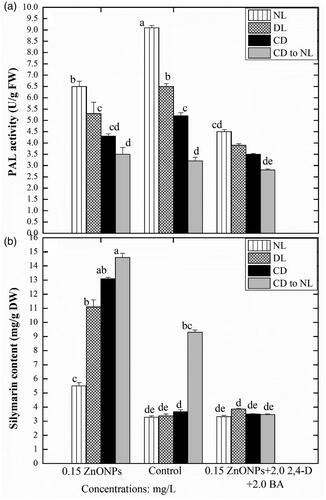
However, the least PAL activity (4.5 ± 0.025 mg/g DW) was detected in the calli proliferated in presence of ZnO NPs in combination with PGRs under normal light conditions, but the same treatment resulted in the highest DPPH FRSA activity (82 ± 1.32%). Nonetheless, the callus proliferated under a higher concentration of ZnO NPs (0.15 mg/L) showed minimum DPPH FRSA activity (72 ± 1.02%) (). The callus proliferated under diffused light and PGRs had enhanced PAL activity (6.5 ± 0.30 mg/g DW), while the same culture proliferated in presence of elevated stress conditions showed least PAL activity (3.9 ± 0.023 mg/g DW), but maximum DPPH FRSA activity (89 ± 1.32%). In complete dark conditions maximum PAL activity (5.2 ± 0.16 mg/g DW) and DPPH activity (88 ± 2.045%) was observed in callus proliferated on MS media containing PGRs (2,4-D and BA 2 mg/L each). A similar treatment with the presence of an elevated concentration of ZnO NPs resulted in the reduced PAL activity (3.5 ± 0.20 mg/g DW) ().
The callus culture developed in presence of ZnO NPs (0.15 mg/L) and kept under complete dark conditions showed lower PAL and higher DPPH activity (4.2 ± 0.20 mg/g DW and 91.5 ± 1.75%), respectively. While the presence of PGRs (2,4-D and BA 2,2 mg/L in the same media and growth conditions, significantly reduced the PAL and DPPH activity (2.8 ± 0.24 mg/g DW and 77 ± 1.32%), respectively in callus cultures.
Maximum activation of SOD activity (4.1 nM/min/mg) was observed when the callus cultures were established at ZnO NPs 0.15 mg/L alone under complete dark conditions. This was followed by the complete dark to normal light, diffused light and normal light condition. Similar trends of light impact were also noted in calli treated with a combination of ZnO NPs and PGRs, showing a moderate level of SOD activity when compared with the control treatment. In the control treatment, maximum SOD activity (3.9) was detected under normal light and a gradual decline was observed in diffused light followed by complete dark. Callus produced under normal light and without any elicitor stress showed maximum SOD activity (3.9 ± 0.046 nM/min/mg FW). The SOD activity was reduced (2.2 ± 0.042 nM/min/mg FW) when culture proliferated in presence of ZnO-NPs (0.15 mg/L). Similar results under diffused light conditions were also observed, highest SOD (3.7 ± 0.045 nM/min/mg FW) was found in the callus induced in MS media containing PGRs (2,4-D and BA 2 mg/L, each), while least in (3 ± 0.066 nM/min/mg FW) ZnO NPs (0.15 mg/L) treated culture ().
Effect of zinc oxide nanoparticles and light intensities on silymarin production
The current research work also quantified the main targeted bioactive compound in callus using HPLC analysis and confirmed the influence of ZnO NPs and light stress interaction on activation of the silymarin biosynthetic pathway. In the selected plant samples derived from callus cultures, silymarin content was identified and quantified through HPLC.
Among culture media composition, a considerable level of silymarin (14.6 mg/g) was detected in calli raised in vitro in response to ZnO NPs (0.15 mg/L) alone when compared with other NPS and PGRs treatments. However, in the control treatment (absence of NPs in MS media) a slight reduction in silymarin content was recorded. Moreover, the addition of ZnO NPs along with PGRs in the culture media drastically inhibited the production of silymarin. Among different light stress treatments, incubation of calli in the complete dark for two weeks and then shifted to normal light produced the highest silymarin content than the rest of the light treatments. While, normal light condition for all tested calli, exhibited severe stress condition that excessively declined the silymarin production in callus cultures.
The stress-induced by the elicitors (ZnO NPs) under various light conditions, significantly enhanced the silymarin content in in-vitro callus cultures. Under the normal light condition, the highest silymarin content in callus culture (5.5 ± 0.015 mg/g DW) was observed when proliferated in presence of ZnO NPs (0.15 mg/L). While the media lacked NPs did not enhance silymarin content. Similar sort of results was also observed when the culture was placed under diffuse light. ZnO-NPs (150 μg/L) treatment also enhanced the silymarin content (13.1 ± 0.021 mg/g DW) in culture kept under complete dark conditions ( and ). However, the media augmented with both ZnO NPs (150 μg/L) and PGRs (2,4-D and BA 2 mg/L, each), reduced the silymarin accumulation in culture with retarded growth. Similar results were also observed when the culture was placed under dark conditions for two weeks only.
Figure 6. HPLC chromatograms of the selected plant samples. (a): standard silymarin, (b): Callus raised in vitro at 0.15 mg/L ZNO NPs under complete dark for two weeks’ period followed by transference to normal light, (c): Callus raised in vitro at 0.5 mg/L ZNO NPs under complete dark, (d): Callus raised in vitro at 0.5 mg/L ZNO NPs under diffused light, (e): Callus raised in vitro at 0.5 mg/L ZNO NPs under normal light, (f): Control treatment under complete dark for two weeks’ period followed by transference to normal light, (g): Callus raised in vitro at 0.15 mg/L ZNO NPs + 2 mg/L BA + 2 mg/L BA under diffused light.
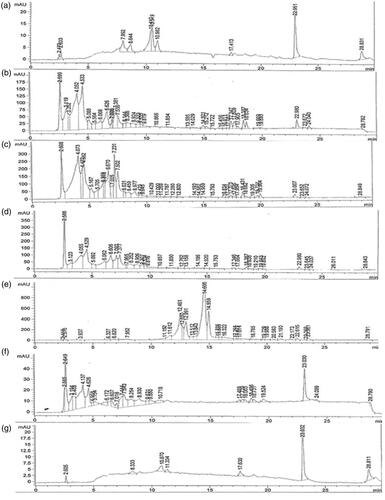
Discussion
The main objectives of the current research work were to investigate the photocatalytic effects of ZnO NPs alone or in combination with notable PGRs under different light sources on callus growth dynamics, secondary metabolism and biosynthesis of silymarin in S. marianum. In an initial experiment, seeds of S. marainum were in vitro germinated on MS0 media (MS media with no PGR) to obtain contamination-free germplasm as a source for a continual supply of different explants. In vitro seed germination is considered is one of the best choices for culture initiation to get sterilized plantlets to be used as a primary source of starter material for in vitro micropropagation studies in plants [Citation28]. In this study, maximum seed germination frequency (75%) was recorded in seeds after four weeks of incubation and healthy plantlets were developed in culture tubes. Similarly, MS media without fortification of PGRs was also reported by Zara et al. [Citation29], for seed germination to obtained the in vitro plantlets of Silybum marianum.
Callus induction is an important step to dedifferentiate the mature plant tissue and mediate the process of in vitro morphogenesis for the production of entire intact plantlets [Citation30]. However, in vitro callogenesis is affected by several factors, such as type and concentration of plant growth regulators age and type of explant, growth media composition and physical factors including pH, temperature and light conditions in the growth room [Citation31]. To select a suitable explant and PGRs for the initiation and establishment of callus cultures in S. marianum in the present study, we used three different explants including root, hypocotyl, and cotyledon cut out from the in vitro germinated seedlings and were cultured on MS media supplemented with the combination of 2.4-D and BA at various doses (1.1 mg/L, 2.2 mg/L or 3.3 mg/L). Significant differences in callus initiation were observed by application of the selected PGRs at different levels and different explants. In PGRs, the best response in callus growth parameters was shown by the combination of an auxin, i.e. 2,4-D and a cytokinin, i.e. BA at a concentration of 2 mg/L each. At this treatment, in less time (4 d), the highest callus induction frequency (90%) and callus biomass (1.3 g; Fresh weight) were recorded. The anticipated reason for the higher growth responses is the provision of the optimal ratio of auxin and cytokinin into growth media where auxin facilitated cell division, cell elongation and callus induction and cytokinin stimulated cell division and the rate of differentiation in the growing explants [Citation32]. Similarly, Sahar et al. (2017) concluded the highest callus induction frequency in Silybum marianum on growth media containing an optimal ratio of auxin (2, 4-D) with a cytokinin (Kn). In another study, it was noted that MS medium added with 2,4-D and BA exhibited the highest callus induction rate in Cnidium officinale [Citation33]. Amongst the different explants, the earliest callus initiating was achieved in root explants, followed by cotyledon and hypocotyl. Moreover, root explant also resulted in the highest callus induction frequency and maximum biomass. The significant variations among explants suggest that successful callus induction protocols are dependent on the source, type and developmental stage of explants [Citation10]. In agreement with our results, maximum callus frequency was achieved from root explant as compared to other explants in Cuminum cyminum [Citation34].
In another study, the combination of 2,4-D and Kin at 2 mg/L each added in MS media containing and 30 g/L sucrose resulted in optimal callus induction in S. marianum using hypocotyl and cotyledon explants, respectively [Citation35,Citation36]. After optimization of root explants as an appropriate explant and 2,4-D and BA (2 mg/L, each) as a suitable PGRs combination for the callus induction, the root explants mediated calli were incubated on MS media supplied with different concentration of ZnO NPs (0.05, 0.1 and 0.15 mg/L) either alone or in combination with PGRs (2,4-D and BA) and kept under various light condition for callus proliferation. Callus proliferation was observed in response to all the applied treatments. In response to normal light conditions, the highest callus biomass formation was recorded on the MS media augmented with 0.05 mg/L of ZnO NPs only, while at higher concentration (0.15 mg/L) of ZnO NPs, a significant decline in callus growth and proliferation was observed. Similarly, under diffused light conditions, MS media supplemented with ZnO NPs (0.1 mg/L) produced maximum callus biomass. Whereas, the same media composition under dark conditions produced the highest callus biomass (1920 mg). It was observed that callus cultures supplemented with a higher concentration of ZnO NPs (0.15 mg/L) and maintained under dark for two weeks followed by transference to normal light showed production of maximum callus biomass (2294 mg). However, when callus was raised at the same light treatment but at 0.10 mg/L ZnO NPs the callus biomass formation ceased significantly in cultures.
To date, no report is available on the interlinking effects of light and ZnO NPs on in vitro micropropagation-related studies including callus organogenesis in any medicinal plant and this is the first report of its kind. However, a few studies are reported on the effects of ZnO NPs without any interaction with a light stress on callus growth attributes. The results of Alharby et al. [Citation37] pointed out that; a highest dose of ZnO NPs (30 mg/L) stimulated the callus growth and biomass formation in Solanum lycopersicum. Likewise, in our study, the presence of 0.15 mg/L ZnO NPs in growth media was found as a suitable dose for callus proliferation.
In another study, various levels (0.1, 1, 10, and 100 mg/L) of ZnO NPs produced a good quantity of callus fresh weight in Stevia rebaudiana [Citation38]. ZnO NPs enhanced callus growth and plant regeneration in tomato plants [Citation37]. A lower concentration of ZnO-NPs (0.01 mg/L) had a significant effect on the callus culture induction and biomass formation in Stevia rebaudiana (fresh weight 0.87 g) [Citation38]. In a similar study, ZnO NPs (100 mg/l) enhanced shoot, root length and seed germination of Brassica nigra [Citation37]. The enhancement of callus growth by employing ZnO NPs can be extrapolated in terms of its potential to provide Zn ions at a sufficient level in the culture media as an essential micronutrient. Zn is supplied to plants cells to tremendously activate the chloroplast pathways [Citation39]. Still, it is unclear that how ZnO NPs impact the chloroplast in a plant cell. But some previous reports endorsing the fact that chloroplast system depends on optimal absorption of zinc ion. Deficient or excessive absorption of zinc has shown detrimental effects on chlorophyll synthesis thus can cause a lower growth rate during callus formation.
It has also been proposed that the application of ionic particles such as zinc in the culture media possesses a positive charge at lower pH while a negative charge at high pH. It was reported that ZnO particle has a surface charge of zero at pH 9.3, and is positively charged at pH 6.0 [Citation40]. However, the pH of growth media used in the current study was adjusted to 5.8 and we can assume that at this pH, ZnO NPs would have positively charged. Interestingly, these positive charged NPs could have made strong interaction with a callus cell wall that has cellulose of negative charge (carboxyl group). Thus, ZnO NPs could adhere efficiently to the callus cell wall and could extensively release Zn+. As a result, absorption of optimal level of zinc ion would have influenced the callus growth and biomass [Citation41]. In this study, the addition of ZnO NPs at 0.15 mg/L to growth media might be the appropriate optimal dose that can fulfil the requirement of zinc ion in the culture media for feasible callus growth and biomass formation. The light factor is also one of the most pivotal growth conditions, playing a significant role in plant morphogenesis, plant architectural and photosynthetic development [Citation17].
In the present study, significant differences in growth parameters of callus were observed in response to different light treatments. Incubation of calli under complete dark conditions for two weeks and then shifting to normal light resulted in the production of a substantial amount of callus biomass as compared with other light treatments. Dark pre-treatment followed by exposure to light might influence the callus biomass formation, as different light intensity has a differential effect on the overall synchronization of physiological and metabolic regulation of plants. According to the observations of Adil et al. [Citation42], callus growth was accelerated by culture incubation under the dark condition as compared to other light treatments. Our results have collaborated with the findings of Bogale et al. [Citation43], where maximum callus proliferation in Colocasia esculenta was positive influenced when cultures were incubated under complete dark conditions for three weeks and then transference to normal light. In another recently published study, maintenance of Olive callus cultures for one week initially in dark conditions produced maximum biomass accumulation [Citation33]. Conversely, Ali et al. [Citation44] observed that dark conditions enhanced the amount of callus in comparison with those cultures kept under light conditions in A. bracteosa. In the context of callus nature such as colour and texture as indicated in , it was observed that ZnO NPs alone in culture media and exposed to normal light, diffused light, complete dark, shifting from complete dark to normal light after two weeks, respectively produced yellowish callus with fragile texture. However, calli raised on growth media ZnO NPs plus PGRs incubated in all the applied light stress treatments resulted in light brownish or dark brownish calli with a compact texture. Variations in callus colour and texture might be linked to cell activity during cell division [Citation42]. In a similar study, Ali et al. [Citation44], concluded that normal light produced soft and brown callus while dark conditions promoted the formation of compact and yellowish callus. It can be speculated that the colour of calluses becomes brown due to overproduction of phenolic compounds as a result of intense stress conditions by combined effects of ZnO NPs and light treatments. These phenolic compounds could slow down growth and can cause necrosis [Citation5]. Elicitation is one of the most effective strategies used to manipulate the in vitro cultures for higher production of appropriate secondary metabolites [Citation45,Citation46]. However, the metabolite productivity of in vitro cultures is dependent on the type of elicitors employed during in vitro cultures. It is therefore important to optimize the most suitable abiotic factors like culture media composition and culture growth conditions to obtain the perpetual production of secondary metabolites. For this purpose, we treated the root-derived calli to various elicitor treatments composed of ZnO NPs and different light stress treatments. In this study, different concentrations of ZnO NPs and light intensities treatments interaction significantly affected TPC, TFC, PAL and DPPH free radical scavenging activity. The phenolic and flavonoid content was significantly enhanced in callus cultures proliferated in presence of ZnO NPs induced stress conditions. The highest TPC and TFC were recorded in cultures developed under complete dark conditions (for two weeks only) containing 0.15 mg/L ZnO NPs.
ZnO NPs supplementation into cultures has shown to enhance TPC and TFC in callus cultures of notable medicinal plants. For instance, the callus culture of Stevia rebaudiana Bertoni developed on media supplemented with 100 mg/L ZnO NPs under normal light, accumulated the maximum TPC (5.65 μg/mg of DW) and TFC (2.85 μg/mg of DW) [Citation38]. In another study, ZnO NPs enhanced TPC (22.12 and 12.18 mg) and TFC (6.38 and 2.7 mg g−1) in in-vitro cultures of Verbena. officinales and Verbena. Tennuisecta, respectively [Citation47]. In this study, minimum TPC and TFC were detected in culture proliferated under normal light and PGRs (2,4-D and BA, 2 mg/L each) only. However, the callus cultures treated with ZnO NPs and kept under dark for 2 weeks followed by transference to normal light, accumulated maximum quantities of TPC and TFC, respectively.
In previous studies, ZnO NPs enhanced DPPH activity (85.91%) at lower concentrations (100 mg/L) in the in vitro micropropagated stevia plants [Citation38]. We also were observed a similar pattern in the present study, wherein ZnO NPs enhanced the DPPH activity of callus cultures in response to all light treatments. However, the culture kept under dark conditions for 2 weeks only, showed the maximum DPPH activity. Nevertheless, some previous studies endorsed the fact that ZnO NPs possess high photocatalytic efficiency among all other inorganic nanomaterials and have a great response to light which significantly activates the interaction of ZnO NPs with plants and microorganisms [Citation3]. Hitherto, the oxidative stress generated by ZnO NPs results in the production of ROS that begins to accumulate in plant cells. Their toxic effects are neutralized by the antioxidant compounds produced by plant cells. Due to a small nano size, increased surface area and having a strong potential to move inside the cells through apoplastic or symplastic route ZnO NPs could lead to more electrostatic interactions with the plant cell membranes. Eventually, they activate the pathways for the synthesis of polyphenols and enhance their accumulation in the cell [Citation35].
The results of the current research are very encouraging, and they show that there is a great potential for the use of such nanoparticles in combination with light intensity to enhance the production of important secondary metabolites in S. marianum. Further, the results of PAL activity showed opposite trends and pointed out that, absence of ZnO NPs in culture media (control treatment) under normal light conditions can produce a higher quantity of PAL (9.10 mg/g) in callus cultures. This was followed by a diffused light, completely dark and complete dark for two weeks then shifted to normal light condition. However, additions of NPs to PGRs containing media drastically reduced the PAL activity (2.8 mg/g) in calli incubated under dark conditions for two weeks and then shifting to normal light. This fashion in PAL activity is ascribed to the PAL turnover, the strategic enzyme responsible for the biosynthesis of phenolic acids and flavonoids in the shikimate pathway. More production of TPC and TFC is indirectly proportional to the PAL activity as some important isozymes of PAL may directly involve in the phenylpropanoid metabolism [Citation13]. In a previous study, a combination of different PGRs (ABA, GA3 and ethylene) enhanced the PAL activity in the hairy root of Salvia miltiorrhiza [Citation48].
Further, we observed that; ZnO NPs either alone or in combination with PGRs in the growth caused a significant increase in SOD activity under different light stress conditions than control treatment (absence of ZnO NPs). Maximum activation of SOD activity (4.1 nM/min/mg) was observed when callus was established at ZnO NPs at 0.15 mg/L alone under complete dark conditions. Since ZnO is a photosensitizer in nature and can generate electron-hole pairs in the presence of light. During intense light, a large bandgap is generated in ZnO that they can’t harvest efficiently the normal light [Citation49]. That way, in our study, ZnO NPs under dark conditions were found to enhance the SOD activity in comparison to other light treatments. Among all of the antioxidant enzymes, SOD is a well-known metalloprotein that catalyzes the dismutation of the superoxide anion to hydrogen peroxide [Citation44], and its activation may provide protection to plant cells from oxidative damages caused by intense stress conditions [Citation27]. Moreover, light is also regarded among limiting factors that affect enzymatic antioxidant mechanism in both in vitro and in vivo conditions depending on the light intensity, and exposure duration [Citation17]. A combination of PGRs (BA, TDZ, GA3) moderately enhanced SOD activity in the in vitro cultures of S. marianum under complete dark and normal light conditions [Citation15]. The silymarin content was also assessed and quantified in all cultures by using HPLC. The highest amount of Silymarin was detected in calli exposed to complete dark condition for two weeks then kept under normal light. This higher production of silymarin might be attributed to the plant defense system for the elimination of ROS to survive in such a stress environment. The induced stress in the plant cell results in ROS production. The positive connection of polyphenols and silymarin biosynthesis might be involved to scavenge the ROS species and provide useful culture conditions for considerable growth. In a previous report, salicylic acid significantly enhanced the silymarin content in the hairy root culture of S. marianum [Citation50]. Among the light treatments, callus culture kept under complete dark conditions accumulated the maximum level of silymarin (13.1 ± 0.021 mg/g DW). Similar results were reported by Hasanloo et al. [Citation51], under complete dark conditions highest silymarin content was accumulated in the fruit of S. marianum.
Conclusions
In conclusion, the interactive role of ZnO NPs and light intensities strongly influenced the callus biomass and biosynthesis of potent secondary metabolites in silybum marianum. A combination of 2, 4-D and BA (2 mg/L, each) favoured optimal callus induction from root explants. Further, for enhancing the callus biomass, calli in the growth media containing ZnO NPs (0.15 mg/L) alone and incubated in complete dark condition for two weeks followed by transference to normal light proved to be the most effective strategy. Besides, similar media composition and culture conditions were also found effective for maximum production of polyphenols, antioxidant compounds and silymarin. Taken together, the outcomes from this study established a first inclusive view of the collaborative influence of ZnO NPs and light intensities on the growth and antioxidant responses in invitro callus cultures. To address various health and commercial concerns, the current protocol paves the way for the production of silymarin and can be used effectively at pharmaceutical, nutraceuticals or cosmeceutical industries. However, further studies are required to elucidate the impact of ZnO NPs and different light intensities on silymarin biosynthesis at a molecular level.
Disclosure statement
No potential conflict of interest was reported by the author(s).
Additional information
Funding
References
- Prakasham RS, Kumar BS, Kumar YS, et al. Production and characterization of protein encapsulated silver nanoparticles by marine isolate Streptomyces parvulus SSNP11. Indian J Microbiol. 2014;54(3):329–336.
- Sabir S, Arshad M, Chaudhari SK. Zinc oxide nanoparticles for revolutionizing agriculture: synthesis and applications. Sci World J. 2014;2014:1–8.
- Ashok B, Hariram N, Siengchin S, et al. Modification of tamarind fruit shell powder with in situ generated copper nanoparticles by single step hydrothermal method. J Bioresour Bioprod. 2020;5(3):180–185.
- Yorseng K, Siengchin S, Ashok B, et al. Nanocomposite egg shell powder with in situ generated silver nanoparticles using inherent collagen as reducing agent. J Bioresour Bioprod. 2020;5(2):101–107.
- Khan MA, Khan T, Riaz MS, et al. Plant cell nanomaterials interaction: growth, physiology and secondary metabolism. Comprehensive Analytical Chemistry, Elsevier, USA; 2019. p. 23–54.
- Ahmad MA, Javed R, Adeel M, et al. Engineered ZnO and CuO nanoparticles ameliorate morphological and biochemical response in tissue culture regenerants of candyleaf (Stevia rebaudiana). Molecules. 2020;25(6):1356.,
- Sirelkhatim A, Mahmud S, Seeni A, et al. Review on zinc oxide nanoparticles: antibacterial activity and toxicity mechanism. Nanomicro Lett. 2015;7(3):219–242.
- Vaseem M, Umar A, Hahn Y-B. ZnO nanoparticles: growth, properties, and applications. Metal oxide nanostructures and their applications. Elsevier, USA. Vol. 5; 2010; p. 1–36.
- Ramesh P, Rajendran A, Meenakshisundaram M. Green synthesis of zinc oxide nanoparticles using flower extract Cassia auriculata. J Nanosci Nanotechnol. 2014;2:41–45.
- Abbasi BH, Khan MA, Mahmood T, et al. Shoot regeneration and free-radical scavenging activity in Silybum marianum L. Plant Cell Tiss Organ Cult. 2010;101(3):371–376.
- Sánchez-Sampedro MA, Fernández-Tárrago J, Corchete P. Yeast extract and methyl jasmonate-induced silymarin production in cell cultures of Silybum marianum (L.) Gaertn. J Biotechnol. 2005;119(1):60–69.
- Post-White J, Ladas EJ, Kelly KM. Advances in the use of milk thistle (Silybum marianum). Integr Cancer Ther. 2007;6(2):104–109.
- Khan MA, Abbasi BH, Shah NA, et al. Analysis of metabolic variations throughout growth and development of adventitious roots in Silybum marianum L.(milk thistle), a medicinal plant. Plant Cell Tiss Organ Cult. 2015;123(3):501–510.
- Grant JE, Odlaug BL. Silymarin treatment of obsessive-compulsive spectrum disorders. J Clin Psychopharmacol. 2015;35(3):340–342.
- Abenavoli L, Izzo AA, Milić N, et al. Milk thistle (Silybum marianum): a concise overview on its chemistry, pharmacological, and nutraceutical uses in liver diseases. Phytother Res. 2018;32(11):2202–2213.
- Tajmohammadi A, Razavi BM, Hosseinzadeh H. Silybum marianum (milk thistle) and its main constituent, silymarin, as a potential therapeutic plant in metabolic syndrome: a review. Phytother Res. 2018;32(10):1933–1949.
- Khan MA, Abbasi BH, Ahmed N, et al. Effects of light regimes on in vitro seed germination and silymarin content in Silybum marianum. Ind Crops Prod. 2013;46:105–110.
- Nikolić R, Mitić N, Miletić R, et al. Effects of cytokinins on in vitro seed germination and early seedling morphogenesis in Lotus corniculatus L. J Plant Growth Regul. 2006;25(3):187–194.
- Khan MA, Abbasi BH, Shinwari ZK. Thidiazuron enhanced regeneration and silymarin content in Silybum marianum L. Pak. J. Bot. 2014;46:185–190.
- Manjkhola S, Dhar U, Joshi M. Organogenesis, embryogenesis, and synthetic seed production in Arnebia euchroma—a critically endangered medicinal plant of the Himalaya. In Vitro Cell Dev Biol – Plant. 2005;41(3):244–248.
- Rani R, Khan MA, Kayani WK, et al. Metabolic signatures altered by in vitro temperature stress in Ajuga bracteosa Wall. ex. Acta Physiol Plant. 2017;39(4):97.
- Zoratti L, Karppinen K, Luengo Escobar A, et al. Light-controlled flavonoid biosynthesis in fruits. Front Plant Sci. 2014;5:534.
- Willson KG, Cox LE, Hart JL, et al. Three-dimensional light structure of an upland Quercus stand post-tornado disturbance. J For Res. 2020;31(1):141–153.
- Ali A, Mohammad S, Khan MA, et al. Silver nanoparticles elicited in vitro callus cultures for accumulation of biomass and secondary metabolites in Caralluma tuberculata. Artif Cells Nanomed Biotechnol. 2019;47(1):715–724.
- Chang C-C, Yang M-H, Wen H-M, et al. Estimation of total flavonoid content in propolis by two complementary colorimetric methods. J Food Drug Anal. 2020;10(3): 34–39.
- Sykłowska-Baranek K, Pietrosiuk A, Naliwajski MR, et al. Effect of L-phenylalanine on PAL activity and production of naphthoquinone pigments in suspension cultures of Arnebia euchroma (Royle) Johnst. In Vitro Cell Dev Biol Plant. 2012;48(5):555–564.
- Kazmi A, Khan MA, Mohammad S, et al. Elicitation directed growth and production of steviol glycosides in the adventitious roots of Stevia rebaudiana Bertoni. Ind Crops Prod. 2019;139:111530.
- Khan I, Khan MA, Shehzad MA, et al. Micropropagation and production of health promoting lignans in Linum usitatissimum. Plants. 2020;9(6):728.
- Rady MR. Plant biotechnology and milk thistle. UK: Springer; 2019. p. 97–147.
- Tůmová L, Tůma J, Megušar K, et al. Substituted pyrazinecarboxamides as abiotic elicitors of flavolignan production in Silybum marianum (L.) gaertn cultures in vitro. Molecules. 2010;15(1):331–340.
- Khan MA, Ali A, Mohammad S, et al. Iron nano modulated growth and biosynthesis of steviol glycosides in Stevia rebaudiana. Plant Cell Tiss Organ Cult. 2020;142(1):1–10.
- Jiménez VM, Bangerth F. Endogenous hormone concentrations and embryogenic callus development in wheat. Plant Cell, Tissue Organ Culture. 2001;67(1):37–46.
- Adil M, Ren X, Kang DI, et al. Effect of explant type and plant growth regulators on callus induction, growth and secondary metabolites production in Cnidium officinale Makino. Mol Biol Rep. 2018;45(6):1919–1927.
- Soorni J, Kahrizi D. Effect of genotype, explant type and 2, 4-D on cell dedifferentiation and callus induction in cumin (Cuminum cyminum L.) medicinal plant. J App Biotechnol Rep. 2015;2:265–270.
- Rady M, Saker M, Matter M. In vitro culture, transformation and genetic fidelity of milk thistle. J Genet Eng Biotechnol. 2018;16(2):563–572.
- Jeeshna MV, Paulsamy S. Evaluation of certain flavonoids of medicinal importance in the wild and micropropagated plants of the endangered medicinal species, Exacum bicolor Roxb. J App Pharm Sci. 2011;1:99.
- Alharby HF, Metwali EM, Fuller MP, et al. Impact of application of zinc oxide nanoparticles on callus induction, plant regeneration, element content and antioxidant enzyme activity in tomato (Solanum lycopersicum Mill.) under salt stress. Arch Biol Sci. 2016;68(4):723–735.,
- Javed R, Yucesan B, Zia M, et al. Elicitation of secondary metabolites in callus cultures of Stevia rebaudiana Bertoni grown under ZnO and CuO nanoparticles stress. Sugar Tech. 2018;20(2):194–201.
- Jabeen N, Maqbool Q, Bibi T, et al. Optimised synthesis of ZnO-nano-fertiliser through green chemistry: boosted growth dynamics of economically important L. esculentum. IET Nanobiotechnol. 2018;12(4):405–411.
- Zhai J, Tao X, Pu Y, et al. Core/shell structured ZnO/SiO2 nanoparticles: preparation, characterization and photocatalytic property. Appl Surf Sci. 2010;257(2):393–397.
- Yoshihara S, Yamamoto K, Nakajima Y, et al. Absorption of zinc ions dissolved from zinc oxide nanoparticles in the tobacco callus improves plant productivity. Plant Cell Tiss Organ Cult. 2019;138(2):377–385.
- Adil M, Ren X, Jeong BR. Light elicited growth, antioxidant enzymes activities and production of medicinal compounds in callus culture of Cnidium officinale Makino. J Photochem Photobiol B. 2019;196:111509.
- Bogale A. Micro-propagation of Colocasia esculenta (cv. Bolosso I) from corm and sprout tip explants. J Agric Biotechnol Sustain Dev. 2018;10:147–156.
- Ali H, Khan MA, Ullah N, et al. Impacts of hormonal elicitors and photoperiod regimes on elicitation of bioactive secondary volatiles in cell cultures of Ajuga bracteosa. J Photochem Photobiol B. 2018;183:242–250.
- Ali M, Abbasi BH. Light-induced fluctuations in biomass accumulation, secondary metabolites production and antioxidant activity in cell suspension cultures of Artemisia absinthium L. J Photochem Photobiol B. 2014;140:223–227.
- Wang H, Provan GJ, Helliwell K. Determination of rosmarinic acid and caffeic acid in aromatic herbs by HPLC. Food Chem. 2004;87(2):307–311.
- Afridi MS, Hashmi SS, Ali GS, et al. Comparative antileishmanial efficacy of the biosynthesised ZnO NPs from genus Verbena. IET Nanobiotechnology. 2018;12:1067–1073.
- Liang Z, Ma Y, Xu T, et al. Effects of abscisic acid, gibberellin, ethylene and their interactions on production of phenolic acids in Salvia miltiorrhiza Bunge hairy roots. PLOS One. 2013;8(9):e72806.
- Georgekutty R, Seery MK, Pillai SC. A highly efficient Ag-ZnO photocatalyst: synthesis, properties, and mechanism. J Phys Chem C. 2008;112(35):13563–13570.
- Khalili M, Hasanloo T, Tabar KSK, et al. Influence of exogenous salicylic acid on flavonolignans and lipoxygenase activity in the hairy root cultures of Silybum marianum. Cell Biol Int. 2009;33(9):988–994.
- Hasanloo T, Khavari-Nejad R, Majidi E, et al. Flavonolignan production in cell suspension culture of Silybum marianum. Pharm Biol. 2008;46(12):876–882.