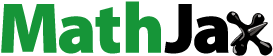
Abstract
In the present investigation, green synthesis of silver nanoparticles (AgNPs) was carried out using aqueous leaf extract of Argyreia nervosa. The results of the spectral characterisation have revealed that the surface Plasmon resonance band was observed at 421 nm confirms the formation of AgNPs. The Fourier Transform Infra-red Spectroscopy result shows the reduction of silver nitrate into AgNPs by the reduction of different functional groups. Transmission Electron Microscope analysis revealed that the particles are roughly spherical and poly-disperse in shape and size, the particles are within the size range of 10–55 nm. Dynamic Light Scattering revealed that the nanoparticles were also within the range of 10–50 nm, An-AgNPs have a high negative zeta potential value of −38.9 mV. An-AgNPs showed efficient free radical scavenging activity and showed excellent antimicrobial activity. Anti-proliferative and cytotoxic effect of An-AgNPs was carried out by MTT assay against KB oral cancer cells, the IC50 value of An-AgNPs is 58.64 µg/ml. The cell's growth is arrested at the G2/M phase, so the An-AgNPs activated the Caspase 3 pathway which leads to the Apoptosis of KB oral cancer cells. So it is concluded that the green synthesised An-AgNPs have manifold functions.
Introduction
Nano-material science is an interdisciplinary science. These promising science studies have wide potential in a sample array of areas of biomedicine, pharmaceutical, and industrial applications. These metal nanoparticles have a broad arrange of applications due to their distinguished physical and chemical properties [Citation1–4]. They are extensively used in several biomedical applications like efficient antimicrobial agents, in vivo imaging, drug delivery, anticancer agents, biosensors. So scientists working in the nanotechnology area are searching for an alternative method for the synthesis of metal nanoparticles with the help of biological sources which is the basis of many technological innovations in the twenty-first century with simple, fast, eco-friendly, and environmentally safe methods popularly known as “Green synthesis” [Citation5–8].
Oral squamous cell carcinoma (OSCC), defined as head & neck squamous cell carcinoma OSCC is about 6% of all malignant diseases diagnosed, and thus the eighth leading cause of cancer death worldwide, with an incidence of 600,000 new cases per year [Citation9,Citation10]. The twentieth century is the era of biologically active metal nanoparticles, due to their wide range of health benefits. However, the validated studies of metal nanoparticles and their effect on malignant tumour cells have begun within the past few decades back. The exceptional and wide range of properties of silver nanoparticles (AgNPs) fabricates them suitable for various biomedical, materials, antimicrobial applications, and nanotoxicology studies [Citation11,Citation12]. In present days the most common application of AgNPs is their use as an antimicrobial agent and in antiseptic wound dressings. In all studies to date, silver nanoparticle toxicity towards vital tissues is much less than the equivalent mass loading of silver salts and chemical compounds [Citation13]. So it is very essential to overcome and find alternative therapies or drugs to overcome these drawbacks. The cytotoxicity of AgNPs has been investigated in various cancer cell models, the results revealed that in vitro studies with different concentrations of AgNPs were capable to interfere with cellular functions of cancer cells and produce toxic effects, like DNA damage and apoptosis [Citation14–18].
In the present study, we have selected one such plant namely “Argyreia nervosa” which was reported to have several medicinal properties and is widely used in Ayurveda. A. nervosa attracted the attention of several researchers worldwide for its pharmacological activities. A. nervosa is mostly spread throughout India, belongs to the family Convolvulaceae is a climbing shrub with a woody tomentose stem. It is commonly known as Elephant Creeper and Samudrapala in Telugu and Samudra-sok in Hindi [Citation19]. The various plant parts of A. nervosa prove to have a broad spectrum of activity on quite a large number of ailments A. nervosa plant parts are reported to contain flavonoids, sterol glycoside, flavonoids, and essential oil which are responsible for several pharmacological properties [Citation20,Citation21].
Keeping given several abundant pharmacological properties, the current work was carried out to biosynthesis silver nanoparticles (AgNPs) using leaf extract of A. nervosa. The An-AgNPs were characterised by different spectral methods and also investigate the antioxidant, antimicrobial studies and carried out in vitro anticancer activity against KB Oral cancer cell line studies to discover their possible therapeutic applications.
Material and methods
Collection of plant material
Fresh leaves of A. nervosa were collected from the Seshachala hills of Tirumala, Tirupati and they are brought to the laboratory and washed with running tap water several times followed by distilled water. Then the leaves were air-dried on coarse filter paper to remove the water moiety then they are shaded for five days after that the leaves are made into powder with the help of an electric blender.
Green synthesis of An-AgNPs
10 grams of fresh leaf powder was added to 100 ml of sterile Milli Q water and it is left overnight at room temperature. The next day morning the sample was heated at 70°C for one hour in a hot water bath. The aqueous leaf extract of A. nervosa was filtered through cheesecloth followed by what man no 1 filter paper. This filtered extract was stored at 4 °C in the refrigerator for further studies and analysis. The green synthesis of AgNPs was carried out as per our previously published research articles protocol [Citation6,Citation8]. The final green synthesised AgNPs was named as An-AgNPs.
Spectral characterization of An-AgNPs
The initial time of colour change of reaction mixture was recorded by visual observation, soon after the green synthesis the An-AgNPs were analysed in Nanodrop-8000, UV-visible spectrometer, and recorded its surface plasmon resonance (SPR) peak. Fourier transform infra-red spectroscopy (FTIR) analysis of A. nervosa leaf extract and green synthesised An-AgNPs was carried out (Bruker Tensor 27) to find out the bioactive compounds present in the leaf extract which are actively involved in bio-reduction and green synthesis of An-AgNPs. High-resolution Transmission electron microscopy (HR-TEM) analysis was used to the detect size and shape distribution and morphology green synthesised An-AgNPs. HR-TEM analysis was carried out by coating a drop of the green synthesised An-AgNPs on a carbon-coated copper grid. Energy Dispersive X-ray (EDAX) analysis was carried out to know the elemental percentage of silver ions and other elements present in green synthesised An-AgNPs. Both TEM and EDAX analysis was carried out using Transmission Electron Microscope (FEI-Tecnai G2 20 Twin). Particle size analysis was done to find out the size distribution of green synthesised An-AgNPs in the aqueous liquid sample through the dynamic light scattering (DLS) method and Zeta potential analysis was carried out to find out the stability of green synthesised An- AgNPs in the aqueous colloidal solution using Nanopartica Horiba SZ-100. Zeta potential analysis is a physical property that gives the net surface charge of the nanoparticles.
Antimicrobial analysis of An-AgNPs
The antibacterial efficiency of green synthesised An-AgNPs was determined against six bacterial strains of both gram-negative bacteria such as Escherichia coli, E. coli (Donor) E. coli (Mutant) E. coli (recipient), and gram-positive such as Staphylococcus aureus and Bacillus megatorium, by using Agar disc-diffusion method. Different concentration of green synthesised An-AgNPs has loaded on 5 mm of sterile Whatman No 1 filter paper discs as follows 10 micrograms (10 mcg), 20 mcg, 30 mcg, and 40 mcg. Amoxicillin 30 mcg (Himedia-SD063) was used as a standard drug for comparison zone of inhibition (ZOI) along with An-AgNPs.
Free radical scavenging activity of An-AgNPs by DPPH method
Free radical scavenging activity of the An-AgNPs was determined by using 2, 2′-diphenyl-1-picrylhydrazyl (DPPH) radical scavenging assay according to the method described by [Citation22]. The DPPH radical scavenging activity (RSA) was measured by determining the absorbance at 517 nm, using ascorbic acid as standard and the antioxidant activity was expressed as IC50. The IC50 is the half-maximum inhibitory concentration of any substance inhibiting a specific biological process or function.
In vitro MTT assay for cytotoxic activity of An-AgNPs
Cell viability was quantified by performing an MTT assay as described by renowned scientists [Citation23]. Seeded 200 μl KB cancer cell suspension in a 96-well plate at required cell density (20,000 cells per well) and allowed the cells to grow for about 24 h. After 24 h different concentrations of An-AgNPs 25 µl, 50 µl, 100 µl, and 200 µl along with standard drug Camptothecin 7.5 uM concentration was added and incubated on the plate for 24 h at 37 °C in a 5% CO2 atmosphere. After the incubation period, the plates were taken out from the incubator and removed spent media and then added MTT reagent to a final concentration of 0.5 mg/mL of total volume and 100 μl of DMSO solubilisation solution was added. The plate was read in a spectrophotometer or an ELISA reader at 570 nm and 630 nm used as the reference wavelength. The IC50 value was determined by using a linear regression equation i.e. Y = Mx + C. Here, Y = 50, M and C values were derived from the viability graph.
Analysis of cell cycle study of An-AgNPs on KB cell lines by flow cytometry
Culture cells in a 6-well plate at a density of 2 × 105 cells/2 ml were added and incubated in a CO2 incubator overnight at 37 °C for 24 h. Aspirate the spent medium and treat the cells with IC50 value of An-AgNPs at the concentration of 58.64 µl/mL and along with standard drug Camptothecin 7.5 uM concentration was added in 2 ml of culture medium, except in cell control and incubate the cells for 24 h. The cell pellet was washed twice with PBS to ensure that only DNA is stained with Propidium iodide (PI stains all nucleic acids), so the cell pellet is treated with 50 μl of Ribonuclease A to get rid of RNA and finally add 400 μl PI solution per million cells directly to cells and mix well. Finally, incubate the cells for 5–10 min at room temperature in dark and then analyse the samples by flow cytometry.
Apoptosis study by dual fluorescence by acridine orange, ethidium bromide and Hoechst 33258 on KB oral cancer cell by An-AgNPs
The KB oral cancer cells are cultured in a 6-well plate at a density of 2 × 105 cells/2 ml and incubate in a CO2 incubator overnight at 37 °C for 24 h. Aspirate the spent medium and treat the cells with IC50 value of An-AgNPs at the concentration of 58.64 µl/mL and along with standard drug Camptothecin 7.5 uM concentration, except in cell control in 2 ml of culture medium and incubate the cells for 24 h. The stained cells were observed under a fluorescence microscope with filter cube with Excitation 560/40 nm and Emission 645/75 nm for EtBr (Ethidium bromide) and Excitation 470/40 and Emission 525/50 for Acridine orange and Hoechst 33258 staining fluorescence microscopy Excitation 352/461 nm and Emission 510/40 nm. The images were overlaid using Image J Software v1.48.
Apoptosis analysis of An-AgNPs on KB cell lines by flow cytometry
The KB oral cancer cells are used in this study. The cells are cultured in a 6-well plate at a density of 0.5 × 106 cells/2 ml and incubated in a CO2 incubator overnight at 37 °C for 24 h. Aspirate the spent medium and treat the cells with IC50 value of An-AgNPs at the concentration of 58.64 µl/mL and along with standard drug Camptothecin 7.5 uM concentration, except in cell control in 2 ml of culture medium and incubate the cells for 24 h. Add 5 μl of FITC Annexin V and gently vortex the cells and incubate for 15 min at RT (25 °C) in the dark, after incubation finally add 5 μl of PI and 400 μl of 1X Binding Buffer to each tube and vortex gently and analyse the samples by flow cytometry immediately.
Analysis of caspase 3 expressions in KB oral cancer cell lines by flow cytometry using An-AgNPs
The KB oral cancer cells were cultured in a 6-well plate at a density of 0.5 × 106 cells/2 ml and incubate in a CO2 incubator overnight at 37 °C for 24 h. Aspirate the spent medium and treat the cells with IC50 value of An-AgNPs at the concentration of 58.64 µl/mL and along with standard drug Camptothecin 7.5 uM concentration, except in cell control, in 2 ml of culture medium and incubate the cells for 24 h. The cells are pelleted at a higher speed and then aspirate the supernatant careful not to lose the pellet, then wash the pellet twice with PBS and then finally add 5 μL of FITC Capspase 3 antibody. Mix thoroughly and incubate for 30 min in the dark at Room Temperature (20° to 25 °C). The cells were washed with 1X PBS with 0.1% sodium azide once and then finally add 0.5 ml of PBS and mixed thoroughly and analyse the samples by Flow Cytometry.
Results and discussion
UV-Visible spectral analysis of An-AgNPs
The fresh aqueous leaf extract has the capability to change silver nitrate solution into an ionic form. In this reaction, the leaf extract reacts with 0.02 mM silver nitrate solution and is changed to dark brown colour (). The surface plasmon resonance spectrum (SPR) peak of green synthesised An-AgNPs was obtained at 421 nm (). In the previous reports on silver nanoparticles synthesised from various plants extracts, the SPR band was noticed to increase the intensity with an increase in time intervals and concentration of AgNO3 from 0.001 M to 0.005 M. Similarly, green synthesised AgNPs showed similar results with different plant extract like leaf extract Artemisia annua, Boerhavia erecta, Decaschistia crotonifolia, Fruit Extract of Solanum nigrum, Momordica charantia, Garlic clove, seed Extracts of Nigella sativa and flower extracts of Aerva lanata, rapeseed flower pollen extract, palm pollen extract and sumac extract mediated silver nanocomposites and so on [Citation24–35].
FTIR analysis of green synthesised An-AgNPs
FTIR analysis of A.nervosa leaf extract and green synthesised An-AgNPs is shown in (). The peaks for A. nervosa leaf extract were found at 3395, 1629, 999, 819, 505.67 cm−1. The peak at 3395 corresponds to the O-H stretch of free hydroxyl alcohol and phenols, The IR band at 1629 cm−1 is due to the N-H bond of primary amines. The IR band at 999 cm-1 and 819 cm−1 corresponds to the C-N stretch of aromatic amines, aliphatic amine groups. The bio synthesised An-AgNPs showed some prominent peaks in FTIR analysis such as 3388, 1629, and 496.49 cm−1. The peaks at 3388 cm−1 were due to O-H bonds of alcohol and phenols the intensity of the peak is reduced when compared with the extract, the peak at 1629 cm−1 corresponds to the stretch of N-H bending of primary amines and 496.49 cm−1 due to aliphatic iodo compounds of C-I stretch. The results revealed that the different phytoconstituents like flavonoids, polyphenols tannins, of the leaf extract have actively participated in the reduction of silver nitrate to An-AgNPs and in capping and stabilisation of the nanoparticles The results were similar to earlier reports on silver nanoparticles synthesised by green methods [Citation24–27]
Particle size and zeta potential analysis of green synthesised An-AgNPs
Dynamic light scattering (DLS) of An-AgNPs was calculated by the scattered light intensity with time, which in turn measures the Brownian motion of green synthesised nanoparticles in the sample. The size of green synthesised An-AgNPs was in the range of 1–39 nm. The average size was found to be 17.1 nm ± 1 nm (). The polydisperse index PI was detected at 0.284. The Zeta potential of bio-synthesised An-AgNPs was −38.9 mV shown in (). The surface charge of An-AgNPs was measured and confirmed that they have a very high zeta potential value of −38.9 mV which was ultimately due to Vander Wall interaction of green synthesised An-AgNPs in colloidal solution.
TEM and EDAX analysis of green synthesised An-AgNPs
TEM analysis data reveals the sizes of green synthesised An-AgNPs were a range of 2 nm −30 nm ± 5 nm with spherical shape with an average size 15.4 ± 2 nm (), the results are similar to DLS data and also the shape of nanoparticles obtained by TEM was similar to earlier reports [Citation5–8,Citation36–40]. Selected area diffraction pattern (SAED) from the TEM analysis shows well-defined sharp spots in a symmetric manner around the bright spots indicates the crystalline nature of An-AgNPs. The elemental composition of the An-AgNPs shows the highest proportion peak of Ag obtained at 3.0 KeV indicates the presence of the major element of silver is present in green synthesised An-AgNPs followed by some minor peak of C, O, Cu. Energy-dispersive X-ray analysis shows the weight percentage of elemental silver is 68.11%, Cu 10.82%, carbon 20.39%, and oxygen 0.69% (). The results were similar to our earlier studies [Citation6,Citation8,Citation24,Citation27].
Antimicrobial analysis of green synthesised An-AgNPs
The antibacterial activity leaf extract mediated green synthesised An-AgNPs showed excellent antibacterial activity against six bacterial species Gram + ve S. aureus, Bacillus megatrium, and Gram –ve E. coli, and antibiotic resistant E. coli (Donor strain) and E. coli (Recipient strain) and E. coli (mutant strain). The zone of inhibition (ZOI) of An-AgNPs at different concentrations of 10 mcg, 20 mcg, 30 mcg, and 40 mcg in different bacterial cultures was shown in and ZOI values in mm were measured and tabulated in by comparing with standard antibiotic Amoxicillin 30 mcg (Himedia-SD063). The fresh aqueous leaf extract at various concentrations does not exhibit any antibacterial activity against all tested bacteria strains. It is concluded that the An-AgNPs at lower and higher concentrations revealed the excellent zone of inhibition lower concentration equal to standard antibiotic and at higher concentration better than an antibiotic. The MIC of An-AgNPs was detected to be less than 5 mcg. The results reveal that the green synthesised An-AgNPs can be used as effective antibacterial agents. The shape of silver nanoparticles also plays the most important role in antimicrobial properties. When compared with spherical and rod-shaped nanoparticles truncated AgNPs exhibited more ZOI activity. Similarly, AgNPs green synthesised using by various plants exhibited very good antibacterial activities on both gram-negative and gram-positive bacteria [Citation6–8,Citation29–35].
Figure 5. (A) Antibacterial activity of green synthesised An- AgNPs on Gram-negative antibiotic-resistant E. coli strains E.coli Mutant strain, E.coli Donor strain, E.coli recipient strain, and gram-positive S. aureus and Bacillus megaterium at different concentrations of 10 mcg, 20 mcg, 30 mcg, and 40 mcg and centre Ab- Antibiotic (Amoxycillin 30 mcg, Himedia-SD063). (B) Graphical representation of Antibacterial activity of green synthesised An- AgNPs on Gram-negative antibiotic-resistant E.coli strains E.coli Mutant strain, E.coli Donor strain, E.coli recipient strain, and gram-positive S. aureus and B. megaterium and antibiotic Amoxycillin 30 mcg Himedia-SD063. (C) Antioxidant activity of green synthesised An- AgNPs by DPPH method.
Table 1. Antimicrobial activity of An-AgNPs.
DPPH antioxidant activity of green synthesised An-AgNPs
Different reactive oxygen species cause oxidative stress in various human disorders like inflammation, cancer, ageing, and neurodegenerative disorders. Various side effects are caused by synthetic antioxidants, so scientists have decided more on the use of bioactive compounds as natural antioxidants to protect the different human disorders against oxidative stress. In the present study, in vitro antioxidant activity of the An-AgNPs was determined by DPPH free radical scavenging assay. An-AgNPs exhibited concentration-dependent scavenging activity against both DPPH [ & ] A. nervosa leaf extract (An-LE) and An-AgNPs showed the maximum inhibition of 54.58% and 69.71% respectively against DPPH radicals at the highest concentration of 250 µg/mL used in this assay. The DPPH activity of An-AgNPs is almost equivalent to standard ascorbic acid 70.71% at the highest concentration. The IC50 values A. nervosa leaf extract (An-LE) 48.63, green synthesised An-AgNPs 35.16, and standard Ascorbic were found to be around 37.73. The results revealed the An-AgNPs have excellent antioxidant activity. The results are similar to most of the earlier reports on silver nanoparticles [Citation6,Citation8,Citation31–34].
Table 2. DPPH antioxidant activity An-AgNPs.
In vitro MTT assay for cytotoxic activity of green synthesised An-AgNPs
In the present study, an MTT assay was carried out to find out the cytotoxic effect and cell viability of KB oral cancer cell lines with An-AgNPs along with standard drug (Camptothecin). KB oral cancer cells were treated with 12.5 µg/ml; 25 µg/ml; 50 µg/ml; 100 µg/ml; and 200 µg/ml concentrations An-AgNPs for 24 h (). After 24 h the IC50 values were calculated. The IC50 value was determined by using a linear regression equation i.e. Y = Mx + C. Here, Y = 50, M and C values were derived from the viability graph (.
Figure 6. (A) MTT assay of cytotoxic studies of green synthesised An-AgNPs on KB-human oral carcinoma cell line and its morphological changes of KB cells observed under an inverted light microscope. (a) Untreated KB cell line, (b) KB cell line cells treated with 25 µg of An-AgNPs, (c) KB-cell line treated with 50 µg of An-AgNPs, (d) KB cell lines treated with 100 µg of An-AgNPs, (e) KB cell lines treated with 200 µg of An-AgNPs, and (f) Standard control(Camptothecin 7.5 uM). (B and C) Comparative percentage of Cell viability studies of green synthesised An-AgNPs on KB-human oral carcinoma cell line by MTT assay and the IC50 value is calculated to be = 58.64 µl/mL.
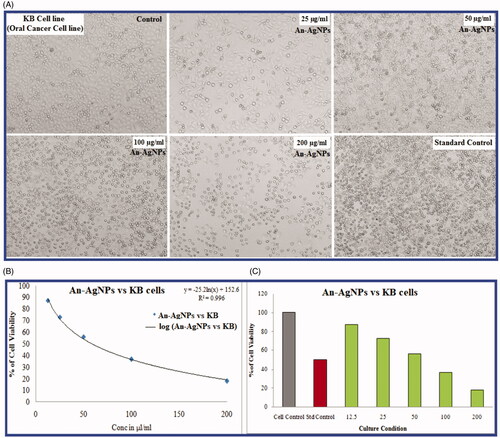
The statistical data of the cell cytotoxicity study reveals that treated KB cells with the An-AgNPs showing IC50 values 58.64 µl/ml respectively. It is clearly seen that the cytotoxicity of An-AgNPs is dosage-dependent on manner from lower concentration to higher concentration as the concentration increase the cell viability decreases shown in . Due to its anticancer nature, the nanoparticles showed cytotoxic potency against Human Oral cancer cells. It is required to conduct further studies to determine the molecular mechanism behind anticancer properties of the An-AgNPs. The earlier reports on various silver nanoparticles biosynthesized also revealed the similar type of results in various cancers cell lines [Citation14]. The green synthesised AgNPs by Artemisia turcomanica, Cynara scolymus, Podophyllum hexandrum showed an anti-cancer effect on gastric cancer cell line (AGS), MCF7 cells, and toxic on human cervical carcinoma cells [Citation15–17]. Likely, Garlic-silver nanoparticles (G-AgNPs), silver NPs (AgNPs) coated with palm pollen extract (Ag–PP(NPs), revealed a remarkable anticancer effect on human breast cancer cell (MCF-7), RFP/Ag-NPs and Ru-AgNPs showed cytotoxicity effects on MDA-MB-231 carcinoma cell line [Citation30,Citation33–35]. AgNPs synthesised by using marine sponge extract Haliclona exigua showed cytotoxic activity on oral cancer cell lines. The AgNPs formed by Coleus amboinicus extracts showed the cytotoxic effect on human oesophageal adenocarcinoma (EAC) cell lines [Citation36,Citation37]. It is concluded that the results of the cytotoxicity study performed by MTT assay suggest that the An-AgNPs are significantly cytotoxic in nature against KB oral cancer cells in a dose-dependent manner as reported in earlier studies on silver nanoparticles.
Cell cycle study of green synthesised An-AgNPs on KB cell lines by flow cytometry
The An-AgNPs showed significant cell inhibition, we have selected IC50 concentrations of 58.64 µg/ml, against the KB cell lines and evaluated the Cell Cycle study by Flow Cytometry to check the stages of the cell cycle arrest. The cells treated with standard control and An-AgNPs with a concentration of 58.64 µg/ml respectively are showing a high % of cells at S and G2/M stages when compared to untreated cells. Hence the cell cycle got arrested at G2/M and S stages. An-AgNPs exhibiting prominent Cell Cycle phase arrest similar to the standard Control, Camptothecin on KB cells (. The AgNPs green synthesised by Hydrastis canadensis and Thuja occidentalis also induce differential cytotoxicity through G2/M arrest in A375 cells which are similar to our results [Citation41], AgNPs green synthesised by Nepeta deflersiana, also revealed that the cells in SubG1 arrest and lead to apoptotic/necrotic cell death in cervical cancer cell lines [Citation42] Silver–palm pollen nanocomposite also showed the morphological changes associated with cell death and accumulation of dead cells in sub-G1 phase [Citation43]. Similarly in the present study also the An-AgNPs showed cell cycle arrest at the G2/M stage which leads to induce cell death, and thus An-AgNPs was proven to be an efficient anticancer agent against oral cancer cells.
Apoptosis studies in KB oral cancer cell by An-AgNPs by dual fluorescence microscopy
The results of fluorescent staining to reveal the apoptotic nature by An-AgNPs treated KB cells that compared to untreated cells, it is showing that more number of early apoptotic cells and late apoptotic cells and necrotic cells were observed with blebbing of the cell membrane and chromatin condensation in the human oral carcinoma cells (). A similar type of results was observed within different cancer cells treated with a range of different concentrations of green synthesised AgNPs [Citation17,Citation18,Citation41–43]. Furthermore, anti-apoptotic effects of Ru-AgNPs on the MDA-MB-231 carcinoma cell line were reported using acridine orange/propidium iodide staining [Citation44]. The apoptotic properties of AgSu/NPs were assessed by st morphological analysis and acridine orange/propidium iodide (AO/PI) and DAPI staining on human breast cancer cells (MCF-7) [Citation45].
Figure 8. (A) Effect of green synthesised An-AgNPs on nuclear damages in KB oral cancer cells were observed with Hoechst 33258 staining under fluorescence microscopy. (a) Control (untreated), (b) Standard Control (7.5 µg/mL) and (c) An-AgNPs (80 µg/mL) for 24 h Analysis of dual fluorescent staining of acridine orange and ethidium bromide on KB oral cancer cells of (a) Untreated, (b) Standard Control and (c) An-AgNPs used to identify apoptosis-associated changes of cell membranes during the process of apoptosis and also to accurately distinguish cells in different stages of apoptosis. (B) Apoptotic assay of green synthesised An-AgNPs on KB-human oral carcinoma cell line by flow cytometry assay. (C) Expression of Apoptotic protein Caspase 3 anlaysis on KB-human oral carcinoma cell line treated with green synthesised An-AgNPs.
Apoptotic assay of An-AgNPs on KB cancer cell by annexin V-FTIC assay
The results of the present study by flow cytometry were shown in . It is observed that KB cancer cell lines treated with An-AgNPs at conc. 58.64 µg/ml and standard drug Camptothecin (7.5 µM) along with cell controls reveals that early apoptosis is 5.76% for An-AgNPs and 49.06% for the standard drug is significantly lower. Whereas the rate of late apoptosis induced by both An-AgNPs and standard control was 71.96% and 11.28% was significantly increased. whereas the percentage of necrotic cells were also observed are as follows 17.62% for An-AgNPs and 1.77% for standard control. The KB oral cancer cells that are in early apoptosis are FITC Annexin V positive and PI negative; and KB oral cancer cells that are in late apoptosis or already dead are both FITC Annexin V and PI positive. So it concluded that the KB cells treated with IC50 concentration of An-AgNPs (obtained in MTT assay) and standard drug Camptothecin (7.5 µM) revealed that the total apoptosis cells were Q1(% of necrotic cells or dead cells) Q2 (% of late apoptosis cells) and Q4(% of early apoptosis) are as follows 95.34% and 62.11% of total apoptosis cells and dead cells and finally Q3 (% of live cells) 4.66% and 37.89 indicating that the An-AgNPs can be an excellent anti-cancer agent against KB oral cancer cells. The apoptosis was significantly increased with biologically synthesised silver nanoparticles when compared with standard control.
Expression of caspase 3 in KB cancer cell line treated with An-AgNPs
The expression of apoptosis protein caspase 3 was evaluated with the dose of IC50 An-AgNPs at conc. 58.64 µg/ml and standard drug Camptothecin (7.5 µM) after 24 h was represented in . Caspase 3 histogram of the gated KB singlet’s distinguishes cells at the M1 and M2 phases. In the present study, M1 refers to negative expression/region whereas M2 refers to the positive expression/region. So the treated and untreated cells were gated at M1 and M2 phases are approximate and can be refined using software (Cell Quest Software, Version 6.0) analysis. The observations in statistical data of Caspase 3 expression study by flow cytometry suggest that the expression of Caspase 3 is very low in Untreated KB Cells in 9.90 MFI [Mean Fluorescence Intensity (MFI)] compared to the standard drug, camptothecin showing 71.06 MFI after the incubation period of 24 h. The test compound An-AgNPs showed Caspase3 expression at 50.05 MFI after the incubation period of 24 h. The results clearly reveal that the An-AgNPs with the IC50 concentration after the incubation period of 24 h showed significant apoptotic potential compared to the standard control, Camptothecin showed the increased expression of apoptotic protein caspase 3. Similar results were observed when treated MCF-7 and Hep 2 cells cell lines with silver nanoparticles biosynthesized from Mentha arvensis, which induced caspase 9 dependent apoptosis. This is due to the Caspase-3 activation and oxidative stress are the reasons for cell deaths [Citation38]. So it is concluded that the An-AgNPs have possible therapeutic potential against human oral cancer-derived diseases. There are several studies on the effect of AgNPs on different cancers, but very few studies are noticed on KB oral cancer cell lines.
Summary and conclusion
The present investigation is concluded that green synthesised AgNPs synthesised by A. nervosa has revealed that the SPR band at 421 nm confirms the formation of silver nanoparticles. The FT-IR results revealed that various functional groups have actively participated in reduction. Transmission Electron Microscope analysis revealed the particles are within the size range from 10 nm to 55 nm which is similar to that of Dynamic Light Scattering results, with a high negative zeta potential value of −38.9 mV. The green synthesised An-AgNPs (A. nervosa-AgNPs) revealed competent free radical scavenging activity and excellent antimicrobial activity against both gram + ve and gram –ve bacteria. The antiproliferative and cytotoxic studies against KB oral cancer cells reveals excellent cytotoxic effect with an IC50 value of 58.64 µg/ml. Further studies on KB oral cancer cells by means of cell cycle assay, Apoptosis assay, and Caspase activity by flow cytometry analysis revealed that the green synthesised An-AgNPs showed wonderful multifunctional therapeutic applications with promising results. It is concluded that An-AgNPs can be employed as future novel anticancer therapeutic agents.
Acknowledgement
The author GK, VSK is grateful to DST-PURSE Centre, S.V.University, Tirupati for providing the facility to carry out the research.
Disclosure statement
The authors declare that there are no conflicts of interest and financial support for the present research article.
Data availability statement
All the data related to our research article is present in the manuscript submitted
References
- Kotakadi VS, Gaddam SA, Venkata SK, et al. Biofabrication and spectral characterization of silver nanoparticles and their cytotoxic studies on human CD34 +ve stem cells. 3 Biotech. 2016;6(2):216.
- Jia M, Zhang W, He T, et al. Evaluation of the genotoxic and oxidative damage potential of silver nanoparticles in human NCM460 and HCT116 cells. IJMS. 2020;21(5):1618.
- Ong C, Lim JZ, Ng CT, et al. Silver nanoparticles in cancer: therapeutic efficacy and toxicity. Curr Med Chem. 2013;20(6):772–781.
- Siddiqi KS, Husen A, Rao RAK. A review on biosynthesis of silver nanoparticles and their biocidal properties. J. Nanobiotechnology. 2018;16(1):14.
- Kotakadi VS, Gaddam SA, Venkata SK, et al. New generation of bactericidal silver nanoparticles against different antibiotic resistant Escherichia coli strains. Appl Nanosci. 2015;5(7):847–855.
- Kanagala P, Gaddam SA, Gunji P, et al. Synthesis of bio-inspired silver nanoparticles by ripe and unripe fruit extract of Tinospora cordifolia and its antioxidant, antibacterial and catalytic studies. Nano Biomed. Eng. 2020;12(3):214–226.
- Slepička P, Slepičková Kasálková N, Siegel J, et al. Methods of gold and silver nanoparticles preparation. Materials. 2019;13(1):1.
- Kumaram VS, Gaddam SA, Kotakadi VS, et al. Multifunctional silver nanoparticles by fruit extract of terminalia belarica and their therapeutic applications: a 3-in-1 system. Nano Biomed Eng. 2018;10:274–279.
- International Agency for Research on Cancer. GLOBOCAN 2012: estimated cancer incidence, mortality and prevalence worldwide in 2012. Lyon (France): World Health Organization; 2012.
- Howlader N, Noone AM, Krapcho M, et al. SEER cancer statistics review, 1975–2011. Bethesda (MD): National Cancer Institute; 2014.
- Zhao Y, Nalwa H. Nanotoxicology—interactions of nanomaterials with biological systems. Valencia (CA): American Scienfic Publishers; 2007.
- Gwinn MR, Vallyathan V. Nanoparticles: health effects-pros and cons. Environ Health Perspect. 2006;114(12):1818–1825.
- Farmer L, Graff A, Szameit S, et al. Toxicological investigation of nanoparticles: effects on human cells. BMC Pharmacol. 2007;7(S2):A55.
- Hembram KC, Kumar R, Kandha L, et al. Therapeutic prospective of plant-induced silver nanoparticles: application as antimicrobial and anticancer agent. Artif Cells Nanomed Biotechnol. 2018;46(Suppl. 3):S38–S51.
- Mousavi B, Tafvizi F, Zaker Bostanabad S. Green synthesis of silver nanoparticles using Artemisia turcomanica leaf extract and the study of anti-cancer effect and apoptosis induction on gastric cancer cell line (AGS). Artif Cells Nanomed Biotechnol. 2018;46(Suppl. 1):499–510.
- Erdogan O, Abbak M, Demirbolat GM, et al. Green synthesis of silver nanoparticles via Cynara scolymus leaf extracts: the characterization, anticancer potential with photodynamic therapy in MCF7 cells. PLOS One. 2019;14(6):e0216496.
- Jeyaraj M, Sathishkumar G, Sivanandhan G, et al. An investigation on the cytotoxicity and caspase-mediated apoptotic effect of biologically synthesized silver nanoparticles using podophyllum hexandrum on human cervical carcinoma cells. Colloids Surf B Biointerfaces. 2013;102:708–717.
- Khorrami S, Zarrabi A, Khaleghi M, et al. Selective cytotoxicity of green synthesized silver nanoparticles against the MCF-7 tumor cell line and their enhanced antioxidant and antimicrobial properties. Int J Nanomedicine. 2018;13:8013–8024.
- Warrier PK, Nambiar VPK, Raman Kutty C. Indian med plant. Orient Longman. 1997;I:191–193.
- Srivatasav A, Shukla Tau SP, Kumar S. Aryl esters and a coumarin from Argyreia speciosa. J Aroma Med Plant. 1998;20(3):774–778.
- Ali R, Ali M, Khan WZ. Pharmacognostical and preliminary phytochemical studies of Argyreia nervosa Burm. Pharmazie. 2003;58(1):60–62.
- Mittal AK, Chisti Y, Banerjee UC. Synthesis of metallic nanoparticles using plant extracts. Biotechnol Adv. 2013;31(2):346–356.
- Mosmann T. Rapid colorimetric assay for cellular growth and survival: application to proliferation and cytotoxicity assays. J Immunol Methods. 1983;65(1–2):55–63.
- Netala VR, Bethu MS, Sana S, et al. Eco-friendly synthesis of silver nanoparticles using leaf extract of flemingia wightiana: spectral characterization, antioxidant and anticancer activity studies. SN Appl Sci. 2020;2:884.
- Adoni M, Yadam M, Gaddam SA, et al. Antimicrobial, anti oxidant and dye degradation properties of biosynthesized silver nanoparticles from Artemisia annua L. Lett Appl BioNanoSci. 2021;10(1):1981–1992.
- Palle SR, Penchalaneni J, Lavudi K, et al. Green synthesis of silver nanoparticles by leaf extracts of Boerhavia erecta and spectral characterization and their antimicrobial. Antioxidant Ad cytotoxic studies on ovarian cancer cell lines. Lett Appl NanoBioSci. 2020;9:1165–1176.
- Palithya S, Gaddam SA, Kotakadi VS, et al. Biosynthesis of silver nanoparticles using leaf extract of Decaschistia crotonifolia and its antibacterial, antioxidant, and catalytic applications. Green Chem Lett Rev. 2021;14(1):137–151.
- Malaikozhundan B, Vijayakumar S, Vaseeharan B, et al. Two potential uses for silver nanoparticles coated with Solanum nigrum unripe fruit extract: biofilm inhibition and photodegradation of dye effluent. Microb Pathog. 2017;111:316–324.
- Malaikozhundan B, Vaseeharan B, Vijayakumar S, et al. Antibacterial and antibiofilm assessment of Momordica charantia fruit extract coated silver nanoparticle. Biocatal Agric Biotechnol. 2016;8:189–196.
- Vijayakumar S, Malaikozhundan B, Saravanakumar K, et al. Garlic clove extract assisted silver nanoparticle – antibacterial, antibiofilm, antihelminthic, anti-inflammatory, anticancer and ecotoxicity assessment. J Photochem Photobiol B. 2019;198:111558.
- Vijayakumar S, Divya M, Vaseeharan B, et al. Biological compound capping of silver nanoparticle with the seed extracts of black cumin (Nigella sativa): a potential antibacterial, antidiabetic, anti-inflammatory, and antioxidant. J Inorg Organomet Polym. 2021;31(2):624–635.
- Palithya S, Gaddam SA, Kotakadi VS, et al. Green synthesis of silver nanoparticles using flower extracts of Aerva lanata and their biomedical applications. Part Sci Technol. 2021. DOI: https://doi.org/10.1080/02726351.2021.1919259.
- Tabrizi MH, Soltani M, Karimi E, et al. Putative mechanism for anticancer properties of Ag-PP (NPs) extract. IET Nanobiotechnol. 2019;13(6):617–620.
- Hajebi S, Tabrizi MH, Moghaddam MN, et al. Rapeseed flower pollen bio-green synthesized silver nanoparticles: a promising antioxidant, anticancer and antiangiogenic compound. J Biol Inorg Chem. 2019;24(3):395–404.
- Ghorbani P, Soltani M, Tabrizi MH, et al. Sumac silver novel biodegradable nano composite for bio-medical application: antibacterial activity. Molecules. 2015;20(7):12946–12958.
- Inbakandan D, Kumar C, Bavanilatha M, et al. Ultrasonic-assisted green synthesis of flower like silver nanocolloids using marine sponge extract and its effect on oral biofilm bacteria and oral cancer cell lines. Microb Pathog. 2016;99:135–141.
- Vadivel S, Suja S. Green synthesis of silver nanoparticles using Coleus amboinicus lour, antioxidant activity and in vitro cytotoxicity against Ehrlich’s Ascite Carcinoma. J Pharm Res. 2012;55:1268–1272.
- Banerjee PP, Bandyopadhyay A, Harsha SN, et al. Mentha arvensis (linn.)-mediated green silver nanoparticles trigger caspase 9-dependent cell death in MCF7 and MDA-MB-231 cells. Breast Cancer. 2017;9:265–278.
- Al-Sheddi ES, Farshori NN, Al-Oqail MM, et al. Anticancer Potential of Green Synthesized Silver Nanoparticles Using Extract of Nepeta deflersiana against Human Cervical Cancer Cells (HeLA). Bioinorg Chem Appl. 2018; Nov 1:2018:9390784.
- Tabrizi MH, Karimi E, Namvar F, et al. Silver–palm pollen nanocomposite exhibits antiproliferative, antioxidant, and proapoptotic properties on MCF-7 breast cancer cells. Research on Chemical Intermediates. 2018;44:6537–6548.
- Das S, Das J, Samadder A, et al. Biosynthesized silver nanoparticles by ethanolic extracts of Phytolacca decandra, Gelsemium sempervirens, Hydrastis canadensis and Thuja occidentalis induce differential cytotoxicity through G2/M arrest in A375 cells. Colloids Surf B Biointerfaces. 2013;101:325–336.
- Al-Sheddi ES, Farshori NN, Al-Oqail MM, et al. Anticancer potential of green synthesized silver nanoparticles using extract of Nepeta deflersiana against human cervical cancer cells (HeLA). Bioinorg Chem Appl. 2018;2018:9390784.
- Tabrizi MH, Karimi E, Namvar F, et al. Silver–palm pollen nanocomposite exhibits antiproliferative, antioxidant, and proapoptotic properties on MCF-7 breast cancer cells. Res Chem Intermed. 2018;44(11):6537–6548.
- Ghandehari S, Tabrizi MH, Ardalan P, et al. Green synthesis of silver nanoparticles using rubia tinctorum extract and evaluation the anti-cancer properties in vitro. IET Nanobiotechnol. 2019;13(3):269–274.
- Ghorbani P, Namvar F, Tabrizi MH, et al. Apoptotic efficacy and antiproliferative potential of silver nanoparticles synthesised from aqueous extract of sumac (Rhus coriaria L.). IET Nanobiotechnol. 2018;12(5):600–603.