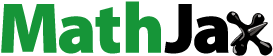
Abstract
The protection of the isolated heart is very important in heart transplantation surgery, meanwhile, the ischaemia/reperfusion (I/R) of the isolated heart is the main cause of its damage. A timely supply of oxygen can significantly improve the prevention of myocardial ischaemia, however, the cardioprotective solution does not have an oxygen supply function. Haemoglobin Based on Oxygen Carriers (HBOCs) is a kind of nano-oxygen drug, which can effectively and timely supply oxygen to hypoxic organs and tissues. However, the oxygen-carrying and releasing capacity (P50) is different with different HBOCs. The aim of our study was to investigate whether STS (a kind of cardioprotective solution, St Thomas Solution) +different P50 HBOCs provide superior myocardial protection and decrease myocardial injury compared to only STS in rats Langendorff isolated heart perfusion model. The results showed that STS + HBOCs can improve cardiac function at 37 °C for 35 min and 120 min, and reduce myocardial infarctions, pathological changes, and apoptosis of cardiomyocytes, and the STS + low P50 HBOCs is more effective than the other two higher P50 HBOCs. We further demonstrated the outstanding protective effect of STS + low P50 HBOCs on cardiac function, reducing myocardial infarctions and apoptosis of cardiomyocytes in rat Langendorff isolated heart perfusion model.
Keywords:
Introduction
Myocardial ischaemia/reperfusion (I/R) injury has become one of the most serious complications after reperfusion therapy in patients acute myocardial infarction and cardiopulmonary bypass [Citation1,Citation2]. Minimizing ischaemic time in both of these clinical scenarios has appropriately received a great deal of attention owing to the long-established relationship between duration of ischaemia and the extent of myocardial injury (MI). The application of nanoscience and technology in the field of medicine provides a simple and effective means for developing the cardioprotective solution. Haemoglobin- base on oxygen carriers (HBOCs), which have been successfully researched by many scientists in the past 20 years, is a typical representative of them [Citation3,Citation4]. Some research reports indicated that HBOCs have a significant protective effect on myocardial I/R injury. Caswell’s team [Citation5] pointed out that after pre-treatment with HBOCs in dog hearts, the myocardial ischaemia was reperfused for 270 min, and a significant 56% reduction in the infarcted area of myocardial tissue compared with the control group, and reduced the release of creatine kinase (CK). Dr. Tao Li [Citation6] found that rat Langendorff isolated heart perfusion model confirmed that HBOCs protected isolated heart from I/R injury and this protection was associated with attenuation of NO-mediated myocardial apoptosis and restoration of the nitroso-redox balance.
However, due to the different structure and quality standards of HBOCs, the effects of these products on MI are not completely understood. Natason [Citation7] and Silverman [Citation8] reported 3,711 and 3,489 clinical research reports in the United States, the five HBOCs products were used to rescue patients with traumatic blood loss, and the survival rates were 91.6% and 91.7% respectively. However, the MI in the HBOCs group was higher than that in the control group, the main cause of MI is oxidative stress caused by excessive oxygen loading. Thus, any imbalance between oxygen supply and metabolic demand leads to functional, metabolic, morphologic, and electrophysiologic alterations, causing cell death. Substantial evidence suggests that controlled reoxygenation may ameliorate postischemic organ dysfunction. We hypothesized that changing the oxygen affinity of HBOCs can relieve MI caused by excessive oxygen loading. P50 (refers to the partial pressure of blood oxygen when the haemoglobin oxygen saturation is 50%) is an important indicator for evaluating oxygen affinity. Theoretically, MI can effectively be lessened with the right dose of oxygen supply caused by oxidative stress. To evaluate this hypothesis, the rat Langendorff isolated heart perfusion model was used to study the protective effect for myocardial on cardioprotective solution without or with different oxygen supply. Theoretically, an ideal cardioprotective solution can not only effectively prolong the preservation time of the isolated heart by right oxygen, but also will not cause new MI due to excessive oxygen.
Therefore, the protective effect of HBOCs with different oxygen affinities on MI induced by I/R injury in rats were investigated by rat Langendorff isolated heart perfusion model, and evaluated its mechanism through enzymology and cytology.
Materials and methods
Experimental animals and groups
45 healthy male SPF Wistar rats (provided by Chengdu Dashuo Experimental Animal Co., Ltd., production licence number: SCKK 2015-030), 6–8 weeks old, weight: 220–300 g; were anaesthetized with an intraperitoneal injection of sodium pentobarbital (50 mg/kg) and heparin (2000 U/kg). 15 rats were randomly divided into 5 groups, 9 rats in each group, and only 3 valid data are selected for each group. Group1, Sham group: Removing the heart (RH), hanging heart, 185 min Krebs-Henseleit buffer (KHB: 120.0 mM NaCl, 4.5 mM KCl, 20.0 mM NaHCO3,1.2 mM KH2PO4, 1.2 mM MgCl2, 2.5 mM CaCl2 and 10.0 mM glucose, pH 7.4, 37 °C) were reperfused, non-arresting; group 2, control group, RH, hanging heart, 30 min KHB perfusion baseline, after 35 min of cardiac arrest with Thomas’ solution (STS: 10 mEq/L Na+, 20 mEq/L K+, 20 mEq/L Cl–, 277.8 mM glucose), 120 min of KHB were reperfused; group 3, low P50 HBOCs group:RH, hanging heart, 30 min KHB perfusion baseline, after 35 min of cardiac arrest with STS + low P50HBOCs, 120 min of KHB were reperfused; group 4, medium P50 HBOCs group: RH, hanging heart, 30 min KHB perfusion baseline, after 35 min of cardiac arrest with STS + medium P50HBOCs, 120 min of KHB were reperfused; group 5, high P50HBOCs group: RH, hanging heart, 30 min KHB perfusion baseline, after 35 min of cardiac arrest with STS + high P50HBOCs, 120 min of KHB were reperfused. The grouping and operation steps during the operation are shown in . The research follows the principles of the Declaration of Helsinki.
Reagents and instruments
Sodium pentobarbital (Sigma, USA, batch number 20171225); Heparin sodium injection (Nanjing Xinbai Pharmaceutical Co., Ltd., batch number 20171004); 0.9% sodium chloride injection (Sichuan Kelun Pharmaceutical Co., Ltd. Co., Ltd., batch number W2017032607); cardiac function analysis system (880-0168ss, AD Instrument); anhydrous calcium chloride (Chengdu Kelong Chemical Reagent Factory, batch number: 2017062101); Sodium chloride (Chengdu Kelong Chemical Reagent Factory, batch number 2017051001), sodium bicarbonate (Chengdu Kelong Chemical Reagent Factory, batch number 2017021301); Potassium dihydrogen phosphate (Chengdu Kelong Chemical Reagent Factory, Batch number 2015010101); potassium dihydrogen phosphate (Chengdu Kelong Chemical Reagent Factory, batch number 2015010101); potassium chloride (Chengdu Kelong Chemical Co., Ltd., batch number: 2017030101); magnesium sulphate heptahydrate (Chengdu Kelong Chemical Co., Ltd. Company, batch number: 2017032101); anhydrous glucose (Chengdu Kelong Chemical Reagent Factory, batch number: 2017060101); PE tube (PE9 050, AD Instrument).
Hbocs information
HBOCs in this study was the poly human placenta haemoglobin (PolyPHb), which was prepared as we previously described [Citation9], briefly, Hb from fresh human placenta blood (donated by Sichuan Neo-life Stem Cell Biotech INC, Sichuan, China) was purified and viral inactived by heat treatment, then intra- and inter-molecularly crosslinking were performed by using bis[3,5-dibromosalicyl]fumarate (DBBF)(different P50) and glutaraldehyde (GDA), respectively, and different doses of DBBF were added to the polymerized product, the HBOCs with different P50 was to be obtained. After that, ultrafiltration, filtration, deoxygenation were performed to harvest PolyPHb with molecular weight range from 64 KD to 600 KD. The prepared PolyPHb solution was added into STS to a final concentration of 5.0 gHb/L, and bubbled sterile air with 5% carbon dioxide for 10 min for oxygenation. The average molecular weight of three P50 HBOCs was 300.0 ± 35.0kd, the dimer content was less than 3.0% and the content of molecules greater than 600kd was less than 3.0%, the pH was controlled at 7.35 ± 0.05, the concentration was 6.0%, the MetHb content was less than 5.0%, and the P50 of the low P50 group was 10.5 mmHg, the P50 of the medium P50 group was 26.3 mmHg, the P50 of the high P50 group was 35.4 mmHg, sterile and pyrogen free. The molecular weight was determined by high performance liquid chromatography (waters e2695), the metHb is detected by multi-wavelength detection method (BECKMAN COULTER), and the P50 is detected by blood gas analyser (HEMOXTM-ANALYSER).
Operation method
The perfusion pressure and ventricular pressure sensor are adjusted, perform zero adjustment, calibrate the sensor pressure, connect the balloon and further check whether the balloon is leaking, and add the configured KHB to the perfusion tube. The test rats were weighed and numbered. Heparinization was performed at a dose of 2000 U/kg heparin sodium. After 10 min 2.0% pentobarbital sodium solution was used for anaesthesia. After anaesthesia, rats were fixed on the operating table and cut at the interstitial site abdominal skin, muscle layer, diaphragm, ribs, expose the heart, clamp the three major branches of the aortic arch with tweezers, quickly disconnect the aorta, pulmonary arteriovenous, and superior and inferior vena cava, pinch the aorta with the forceps, and place the heart in the ice-water bath at 0–4 °C. In the pre-cooled KHB, lightly squeeze the blood in the ventricle by hand, keep the peristaltic pump on, turn on the flow switch on the host, adjust the knob to make the flow rate about 2.5 mL/min, and quickly hang the isolated rat heart on aortic perfusion tube and ligate the aorta. Cut a small incision from the root of the left atrial appendage, pass the balloon through the base of the left atrial appendage, insert it into the left atrium, and finally insert it into the left ventricle through the mitral valve. By injecting water into the balloon, the left ventricular end-diastolic pressure (LVEDP) was adjusted to (8.5 ± 3.5) mmHg. Adjust the flow rate so that the perfusion pressure reaches (80.0 ± 5.0) mmHg, hold it on, and continuously maintain the heartbeat of the isolated heart through the constant pressure model, and record the data.
Evaluation indicators and related detection methods
Cardiac function evaluation
Record cardiac function indicators of rats during baseline, stagnation and reperfusion, including heart rate (HR), perfusion flow, LVEDP, left ventricular development pressure (LVDevP), the maximum rate of left ventricle pressure rise during isovolumic systole (+dp/dt max), the maximum rate of left ventricle pressure drop during isovolumic systole (-dp/dt max) and comparative analysis.
Evaluation of cardiac oxygen consumption
The blood gas index of arteries and coronary veins in the basic state and ischaemia-reperfusion stage is detected, and the cardiac oxygen consumption is evaluated and analysed. Based on the formula
the O2-releasing capacity of PolyPHb in STS was calculated from the O2 partial pressure (PO2) and O2 saturation (sO2) (i-STAT portable blood gas analyser; i-STAT Corp., Windsor, NJ, USA) of the samples, which were collected during the first 5 min of heart arrest, including the cardioplegic solutions (same as the preservation solutions) and the corresponding effluents.
Heart injury
Enzymatic indicators: including lactate dehydrogenase (LDH), creatine kinase MB (CK-MB), α-hydroxybutyrate dehydrogenase (α-HBDH), and troponin I (cTn I); HE staining of pathological sections; Western blot detection of apoptosis indicator Caspase-3. LDH, CK-MB,andα-HBDH were measured with commercial ELISA kit (Nanjing Jiancheng Bioengineering institute, China), cTnI was measured with a commercial ELISA kit (Roche Diagnostics, IN, USA).
Mitochondria isolation
Cardiac mitochondrial was prepared by differential centrifugation. Briefly, After myocardial tissue was minced, and the tissue samples were homogenized in 10 volumes of buffer A (250 mM sucrose; 10 mMTris-HCl, pH 7.4; 1 mM EDTA, pH 7-8; 1 mM orthovanadate; 1 mM NaF; 0. 3 mM phenylmethylsulfonyl fluoride (PMSF); 5 μg/mL each of leupeptin, aprotinin, and pepstatin A) and subjected to serial centrifugations of 1000, 10,000, and 100,000 g. The 1000 g pellet (nuclear fraction) was discarded and the 10,000 g pellet (mitochondrial fraction) was washed in buffer A and recentrifuged. The final pellet was resuspended in buffer B (150 mM NaCl; 20 mM Tris-HCl, pH 7.4; 10 mM EDTA, pH 7-8; 1 mM orthovanadate; 1 mM NaF; 0.3 mM PMSF; 0. 5 μg/mL pepstatin A; 5 μg/mL each of leupeptin and aprotinin; and 1% NP-40) and subjected to a 21,000 g centrifugation for 10 min. The resultant supernatant were defined as the mitochondrial fraction, isolated mitochondria was kept on ice and used within 3 h of isolation.
Detection of mitochondria ATP and cytochrome C
mitochondria ATP and cytochrome C levels were measured using reverse-phase high-pressure liquid chromatography (waters 2695, 2489) with an ODS C18 column (5 μm, 4 × 125mm, Agilent),as described previously [Citation10].
Detection of oxidative stress
Detection of cardiac oxidative stress: MDA production, superoxide dismutase (SOD) activity; Caspase-3 activity test: take the tissue homogenate and measure the caspase-3 activity by commercial ELISA kit (Nanjing Jiancheng Bioengineering institute, China), the detection methods of all indicators refer to the operating specifications of the ELISA kit.
Histological examination
After 120 min perfusion in the Langendorff device, tissue was excised from the middle of the heart between the apex and base, fixed with 10% neutralized formalin, dehydrated, and embedded in paraffin. Five-micron sections were cut, and randomly selected sections were stained with haematoxylin and eosin. The sections were observed under the light microscope LV tissue was also stained with haematoxylin and eosin (HE) for standard histological examination. The pathological picture analysis of this study was provided by Chengdu Lilai Biotechnology Co., Ltd. The main basis for the scoring was the standard established by Chengdu Lilai Biotechnology Co., Ltd.: the scoring criteria for the degree of inflammation infiltration. Pathological slices with no or occasionally a single inflammatory cell scattered distribution are scored as 0; Pathological slices with mild, with a small amount of inflammatory cells are scored as 1; Pathological slices with moderate, with more inflammatory cell aggregation are scored as 2; Pathological slices with severe, with a large number of inflammatory cells seen Aggregate or diffuse distribution are scored as 3.
Data analysis
Data are expressed as Mean ± SD. The recovery of LVDevP, LVEDP, ±dp/dt, and HR at the 120 min reperfusion period between five groups was compared by ANOVA. The release of CK-MB, LDH and α-HBDH, cTnI, Caspase-3, Cyt-C, ATP, SOD and MDA was compared by ANOVA, and the data of two groups are compared using Duncan’s test, p < .05 was significant difference.
Results
Cardiac function indicators
There was no significant difference in HR, LVDevP, LVEDP, ±dp/dt at 30 min of basal perfusion (p > .05). There was no significant difference in HR between the groups during the 120 min reperfusion period (p > .05). LVDevP, LVEDP, the recovery of ± dp/dt of three HBOCs groups were better than the control group significantly (p < .05); LVDevP, LVEDP, the recovery of ± dp/dt of the low P50 HBOCs group was better than that of the medium and high P50 HBOCs groups significantly (p < .05) ().
Figure 2. The heart rate(A), LVEDP(B), LVDevP(C), ±dp/dt(D and E) of the heart in each group in the baseline and after 120 min reperfusion. The data of at the 120 min reperfusion period between five groups was compared by ANOVA (n = 3). *p < 0.05 **p < 0.01 vs Control group, &p < 0.05, &&p < 0.01 vs Low P50 HBOCs.
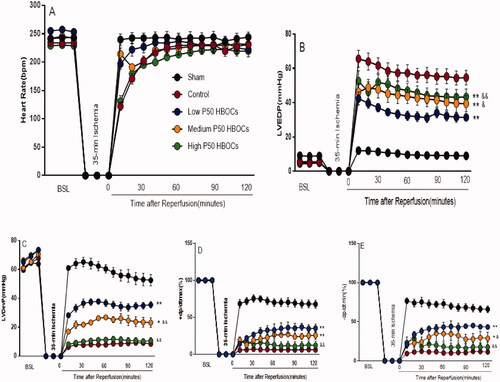
Cardiac oxygen consumption
At the baseline, ischaemic and end of reperfusion periods, there was no significant difference in blood gas PO2 among the each groups ().
Figure 3. Partial oxygen pressure (PO2) in the perfusion fluid during the baseline, ischemia, and after 120 min reperfusion. Data between five groups was compared by ANOVA (n = 3). At the baseline, ischemic and terminal periods, there was no significant difference in blood gas PO2 among the each groups.
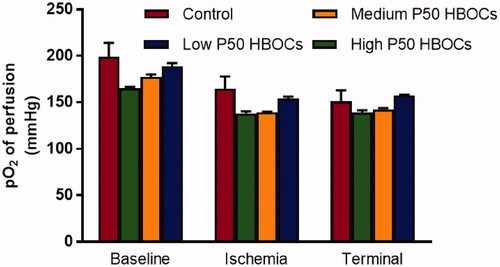
MI Marker enzyme
At baseline, there was no significant difference in the amount of CK-MB, LDH, α-HBDH, and cTnI released from each group of heart. After 120 min reperfusion, the release of CK-MB, LDH, α-HBDH and cTnI in the myocardium of the control group and HBOCs groups were significantly increased (p < .05). The release amount CK-MB, LDH, α-HBDH, and cTnI of the myocardium in HBOCs groups were significantly lower the control group (p < .05). The release amount of CK-MB, LDH, α-HBDH and cTnI of the low P50 HBOCs group was lower than that of the medium and high P50 HBOCs groups significantly (p < .05) ().
Pathological HE staining
The results of HE staining (both magnifications are 400 times) showed that: compared with the control group, the three groups containing HBOCs had improved with inflammation, the low P50 HBOCs group had the most obvious improvement, and the difference was statistically significant. The inflammation score of photomicrographs of HE-stained of cardiac tissue in the low P50 HBOCs was significantly lower the control group and Medium P50 HBOCs (, ).
Figure 5. Representative photomicrographs of HE-stained of myocardium in each group. Magnification *400, scale bar: 50 μm. 4 photomicrographs per group. The inflammation of photomicrographs of HE-stained of cardiac tissue in the low P50 HBOCs was significantly lower than the control group and Medium P50 HBOCs. The first group serves as a control group to study the protection of the myocardium in the other three groups.
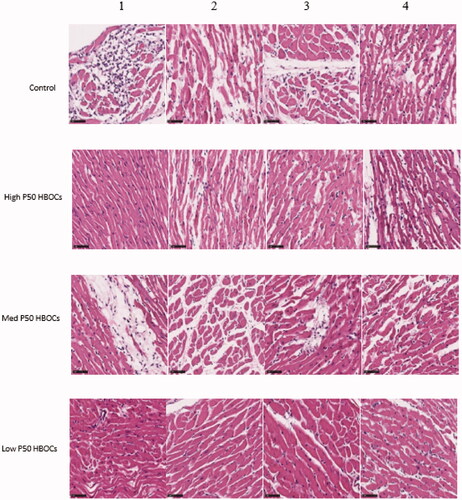
Table 1. Differences in the inflammation score of photomicrographs of HE-stained of cardiac tissue in each group (n = 4) (
±SD).
Caspase-3
The Activity of Caspase-3 in cardiomyocytes in the low P50 HBOCs group was significantly lower than in the other HBOCs groups ().
Cytochrome C
shows that compared with the sham group, the content of cytochrome C in cardiomyocytes of the control group was significantly reduced. The content of cytochrome C in the low P50 HBOCs group and medium P50 HBOCs group was higher significantly than the control group, the content of cytochrome C in the low P50 HBOCs group was higher significantly than the other two HBOCs groups (p < .05).
Mitochondrial ATP
shows that compared with the sham group, the content of ATP in mitochondria was significantly reduced in the other four groups, and there was no significant difference in the content of ATP in cardiomyocyte mitochondria of control group and three HBOCs groups.
Figure 8. Mitochondria ATP concentration in each group. Data between five groups was compared by ANOVA (n = 3). The mitochondria ATP concentration in sham group was significantly higher than the other experimental groups, and there was no statistically significant difference between the four experimental groups.
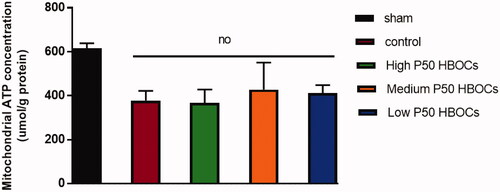
Oxidative stress
shows there was no significant difference of the content of SOD in cardiomyocytes of each group, and shows that the content of MDA in the low P50 HBOCs group was significantly lower than high P50 HBOCs group.
Discussion
HBOCs developed through nanotechnology have maintained the red blood cell’s carrying/releasing oxygen function. The radius of the HBOCs particles is only tens of nanometres, which is less than one percent of normal human red blood cells. The red blood cells cannot pass through the pathological capillaries, while the HBOCs can easily pass through and diffuse to the distal end of the damaged microcirculation blood vessels. It is of great significance to effectively alleviate the body’s hypoxia and improve ischaemia-reperfusion injury [Citation11,Citation12]. However, all organs and tissues require different amounts of oxygen under hypoxic conditions, which requires evaluating the effect of HBOCs with different oxygen affinities on hypoxic tissues. Oxygen affinity is an important indicator for evaluating the role of polymerized haemoglobin in transporting oxygen. Theoretically, different conditions and hypoxic tissues may have different needs for oxygen, so it cannot be generalized, and different oxygen supply doses and modes are required for different organs and tissues [Citation10,Citation13,Citation14]. In this study, the main purpose is to develop a cardioplegia protection solution to increase the protective effect on the heart during cardiac arrest, an isolated heart model was designed to evaluate the protective effect of different P50 HBOCs.
Low P50 HBOCs have better protective effect on cardiac function, the indexes in this study to evaluate cardiac function included LVDevP, LVEDP, ±dp/dt and HR. The results suggest that the recovery of ± dp/dt in the HBOCs groups was better than that of the control group (p < .05), the recovery of ± dp/dt in low P50 HBOCs group was better than that of the medium and high P50 HBOCs groups (p < .05). The possible reason is that the heart is an organ that consumes a lot of oxygen, the ischaemic area should be capillaries firstly, the high P50 HBOCs can provide sufficient oxygen to the large blood vessels in time, but it can’t be maintained oxygen during transportation to the capillaries. Aerobic conditions, because this study belonging to a model study of an isolated heart cannot be supplemented with oxygen in the body’s circulation, it is necessary to convert all haemoglobin to oxyhaemoglobin before perfusion in order to play a role of oxygen supply in the isolated heart. The role of oxygen supplementation is particularly important for the balanced release of oxygen in large blood vessels and capillaries [Citation15,Citation16]. So, the control group can’t provide oxygen, hypoxic tissues and organs cannot get oxygen supply in time, causing damage to cells and the body, and the high P50 HBOCs can provide sufficient oxygen to the large blood vessels in time, but it can’t be maintained oxygen during transportation to the capillaries, low P50 HBOCs can release oxygen in large blood vessels and appropriate amount of oxygen in capillaries. Therefore, it can effectively protect myocardial cells and optimize the cardiac function.
The index for evaluating MI in this study was to select cTnI and three myocardial enzymes, CK-MB, LDH, and α-HBDH. After 30 min of ischaemia, the release of cTnI, CK-MB, LDH, and α-HBDH in the myocardium of the control group was significantly increased (p < .05), indicating that the myocardium had shown necrosis. Compared with the control group, the release of cTnI, CK-MB, LDH, and α-HBDH in the myocardium of the groups containing HBOCs significantly decreased, indicating that HBOCs, both low P50 and high P50 HBOCs, have obvious protective effect on myocardium. The possible reason is that HBOCs can effectively provide oxygen and can play an important role in improving myocardial hypoxia [Citation17].
The inflammation score of photomicrographs of HE-stained of cardiac tissue in the low P50 HBOCs was significantly lower the control group (p < .05). The results suggest that a reasonable oxygen supply can effectively improve MI. It is most commonly thought that main cause of toxicity is not oxygen but ROS that are formed as a product of oxygen metabolism. Protons are transported across the inner mitochondrial membrane by an electron transport chain that finishes in the acceptance of electrons by molecular oxygen. Meanwhile, a small amount of these electrons are incompletely reduced to form the superoxide radical, oxygen. Superoxide can react with lipids to form lipid peroxides, and excessive superoxide can cause apoptosis and premature cell death. Thus one important implication that oxygen supply be carefully linked to energy production is that ATP production, in excess of demand, could lead to excessive accumulation of ROS. The possible reason is that the effects of ischaemic injury in the control group are similar the cause of MI of ROS generated in the body by overload of oxygen supply in high and medium P50 HBOCs, so the myocardium of rats in the high and medium P50 HBOCs group are similar to the control group, the results appear to exclude ROS as culprit of injury, and oxidative stress as a mechanism of protection for low P50 HBOCs.
Caspase-3 is a protein in the cytoplasm that plays a key regulatory role in cell apoptosis. Compared with the other two HBOCs groups, the activity of caspase-3 in the low P50 HBOCs group is significantly reduced (p < .05), which indicating that the low P50 HBOCs can reduce myocardial apoptosis effectively. Some studies [Citation18,Citation19] had pointed out that during cell hypoxia, the intracellular caspase-3 will be significantly increased, and the caspase-3 will be reduced after oxygen treatment. Other studies [Citation20,Citation21] had pointed out that the oxidative stress response will also increase the activity of caspase-3. The low P50 HBOCs can provide reasonable oxygen to cardiomyocytes, which neither cause obvious hypoxia, nor cause obvious oxidative damage.
Cytochrome C is a very important electron transporter for biological oxidation. It is arranged in the respiratory chain with other oxidases on the mitochondrial crest and participates in the cell respiration process. The content of cytochrome C in the low P50 HBOCs group and medium P50 HBOCs group was higher significantly than the control group, the content of cytochrome C in the low P50 HBOCs group was higher significantly than the other two HBOCs groups (p < .05), suggesting that under hypoxic conditions, the cytochrome C content will be significantly reduced, after appropriate oxygen supply intervention, cytochrome C content increased.
The results showed that there was no significant difference in the content of ATP in mitochondria of all groups. And the results showed that the content of MDA was significantly lower in the low P50 HBOCs group than in the other two groups of HBOCs group. If oxygen is oversupplied by HBOCs, there were at least one predictable consequence: the means to detoxify oxygen radicals could be overwhelmed, increasing the overall level of oxidative stress. It is proved that compared with the higher P50 HBOCs, the level of oxidative stress is significantly reduced in the low P50HBOCs group.
HBOCs can improve cardiac ischaemia and ischaemia-reperfusion injury. The possible reason is that the size of HBOCs is much smaller than erythrocytes, which can freely pass through microcapillaries to improve the perfusion effect. HBOCs have good ability to carry/release oxygen, and can meet organs and tissues have a balanced oxygen supply and demand; HBOCs have a small molecular weight and a lower viscosity than erythrocytes, which is beneficial to the perfusion of ischaemic and hypoxic tissues, relieves the glycolytic pathway, reduces lactose production, and reduces ischaemic reperfusion injury.
The process of ischaemia-reperfusion injury may include three ways. First, insufficient oxygen is not provided to the organs or tissues that are hypoxic in time, and the process of hypoxia causes damage to the myocardium; Second, the oxygen supply overload causes oxidative damage to the cardiomyocytes; Third, the process of ischaemia and reperfusion itself causes damage to the myocardium. These three pathways are related to each other and interact with each other to cause the final impact on ischaemic cardiomyocytes, not only in the myocardial function, but also in changes in myocardial enzymes. The possible reason is that HBOCs with low P50 HBOCs can provide sufficient oxygen for the hypoxic myocardial in time, without the possibility of oxygen overload, and have the lowest oxidative damage for cardiomyocytes. However, how the three pathways specifically affect the release of myocardial enzymes is unclear and needs further research to determine.
In conclusion, STS solution containing HBOCs can improve the function of reperfusion of the heart at 37 °C for 120 min, and reduce myocardial infarction, pathological changes and apoptosis of cardiomyocytes, and the STS solution containing low P50 HBOCs is more effective than the other two higher P50 products.
Supplemental Material
Download PDF (134.2 KB)Supplemental Material
Download PDF (113.3 KB)Acknowledgments
The data that supports the findings of this study are available in the supplementary material-for review of this article, and all data is included in the manuscript file.
Disclosure statement
No potential conflict of interest was reported by the author(s).
Additional information
Funding
References
- Slottosch I, Liakopoulos O, Kuhn E, et al. Controlled lung reperfusion to reduce pulmonary ischaemia/reperfusion injury after cardiopulmonary bypass in a porcine model. Interact Cardiovasc Thorac Surg. 2014;19(6):121–970.
- Guo J, Wang SB, Yuan TY, et al. Coptisine protects rat heart against myocardial ischemia/reperfusion injury by suppressing myocardial apoptosis and inflammation. Atherosclerosis. 2013;231(2):384–391.
- You Z, Li Q, Li B, et al. Isovolemic hemodilution with glutaraldehyde-polymerized human placenta hemoglobin (PolyPHb) attenuated rat liver ischemia/reperfusion injury. Artif Cells Nanomed Biotechnol. 2014;42(2):83–87.
- Chang TMS. Blood replacement with nanobiotechnologically engineered hemoglobin and hemoglobin nanocapsules. Wiley Interdiscip Rev Nanomed Nanobiotechnol. 2010;2(4):418–430.
- Caswell JE, Strange MB, Rimmer DM, III, et al. A novel hemoglobin-based blood substitute protects against myocardial reperfusion injury. Am J Physiol-Heart Circ Physiol. 2005;288(4):H1796–1801.
- Li T, Li J, Liu J, et al. Polymerized placenta hemoglobin attenuates ischemia/reperfusion injury and restores the nitroso-redox balance in isolated rat heart. Free Radic Biol Med. 2009;46(3):397–405.
- Natanson C, Kern SJ, Lurie P, et al. Cell-free hemoglobin-based blood substitutes and risk of myocardial infarction and death: a meta-analysis. JAMA. 2008;299(19):2304–2312.
- Silverman TA, Weiskopf RB. Hemoglobin-based oxygen carriers: current status and future directions. Transfusion. 2009;49(11):2495–2515.
- Zhou W, Zhao L, Wang J, et al. An optimal polymerization conditions for poly-human placenta hemoglobin with lower mean molecular weight. Artif Cells Nanomed Biotechnol. 2013;41(5):289–292.
- García-Ruiz JM, Galán-Arriola C, Fernández-Jiménez R, et al. Bloodless reperfusion with the oxygen carrier HBOC-201 in acute myocardial infarction: a novel platform for cardioprotective probes delivery. Basic Res Cardiol. 2017;112(2):17–17.
- Meng F, Kassa T, Jana S, et al. Comprehensive biochemical and biophysical characterization of hemoglobin-based oxygen carrier therapeutics: all HBOCs are not created equally. Bioconjug Chem. 2018;29(5):1560–1575.
- Alayash AI. Mechanisms of toxicity and modulation of hemoglobin-based oxygen carriers (HBOCs). Shock. 2019;52(1S):41–49.
- Li T, Yang G, Zhu Y, et al. Beneficial effects of novel cross-linked hemoglobin YQ23 on hemorrhagic shock in rats and pigs. J Surg Res. 2017;210:213–222.
- Kuang L, Zhu Y, Zhang J, et al. A novel cross-linked haemoglobin-based oxygen carrier is beneficial to sepsis in rats. Artif Cells Nanomed Biotechnol. 2019;47(1):1496–1504.
- Edmondson M, Jana S, Meng F, et al. Redox states of hemoglobin determine left ventricle pressure recovery and activity of mitochondrial complex IV in hypoxic rat hearts. Free Radic Biol Med. 2019;141:348–361.
- Zhang Z, Zhang Y, Deng Y, et al. Polymerized human placenta haemoglobin attenuates myocardial injury and aortic endothelial dysfunction in a rat model of severe burns. Artif Cells Nanomed Biotechnol. 2018;46(6):1141–1145.
- Wang Q, Hu L, Hu Y, et al. Carbon monoxide-saturated hemoglobin-based oxygen carriers attenuate high-altitude-induced cardiac injury by amelioration of the inflammation response and mitochondrial oxidative damage. Cardiology. 2017;136(3):180–191.
- Pan YL, Han ZY, He SF, et al. miR-133b-5p contributes to hypoxic preconditioning-mediated cardioprotection by inhibiting the activation of caspase-8 and caspase-3 in cardiomyocytes. Mol Med Rep. 2018;17(5):7097–7104.
- Li H, Lv B, Kong L, et al. Nova1 mediates resistance of rat pheochromocytoma cells to hypoxia-induced apoptosis via the Bax/Bcl-2/caspase-3 pathway. Int J Mol Med. 2017;40(4):1125–1133.
- Owumi SE, Dim UJ. Manganese suppresses oxidative stress, inflammation and caspase-3 activation in rats exposed to chlorpyrifos. Toxicol Rep. 2019;6:202–209.
- Khafaga AF, El-Sayed YS. All-trans-retinoic acid ameliorates doxorubicin-induced cardiotoxicity: in vivo potential involvement of oxidative stress, inflammation, and apoptosis via caspase-3 and p53 down-expression. Naunyn Schmiedebergs Arch Pharmacol. 2018;391(1):59–70.