Abstract
The vacuoles in Saccharomyces cerevisiae are the key players digesting the waste within the cell. This functional organelle corresponding to the lysosome of mammalians contains acidic hydrolases and specific membrane proteins. Vacuoles have more than 60 hydrolytic enzymes and can easily be modified by genetic engineering. In previous study, we optimised the encapsulation condition with appropriate time and concentration and confirmed the use of vacuole as drug delivery carrier for acute myeloid leukaemia treatment. In this study, recombinant vacuole that could target the acute myeloid leukaemia cell line was constructed. The vacuoles derived from genetic engineered yeast were decorated with targeting peptide that has specific affinity with TLR2 on AML cell membrane. The anti-cancer efficacy of AML targeting vacuoles carriers with encapsulated daunorubicin was shown to be higher than normal vacuole carriers and the crude daunorubicin. The results confirmed that target selective chemotherapy using the vacuole drug delivery system is effective and offers potential for cancer therapy.
Introduction
With the advent of the super-aged society, there is increasing demand for novel targeting drug treatments with lower side effects and high therapeutic efficiency. These strategies not only prevent damage to normal cells, but also increase drug delivery efficiency and are considered to be effective treatment methods. Several drug delivery systems (DDS), such as liposomes, polymer nanoparticles, and dendritic peptides, can be adapted to suit individual targets [Citation1]. However, there remains a gap between technological advances and clinical applications. The synthetic polymer DDS can cause intrinsic cytotoxicity and immune response [Citation2]. To overcome these huddles, bio-derived vesicles are reported as potential alternative drug delivery carriers [Citation3].
Budding yeast, Saccharomyces cerevisiae, has vacuoles that correspond to the lysosomes of mammalian cell. The vacuoles represent membrane-bound organelles, and the composition of the yeast vacuolar membrane is similar to that of the mammalian cell membrane. It is non-pathogenic, and easily modified by genetic engineering. The vacuolar enzymes have a variety of functions, specialised in the digestion and degradation of cellular components. With the advantage of vacuoles, specific targeting ability can be introduced by genetic engineering. To induce the targeting ability, specific binding peptide to cancer was expressed on the vacuolar membrane.
In the common eukaryotic lysosome or vacuole, membrane proteins are made with signal sequences that target them at the endoplasmic reticulum, and then sorted in the Golgi region away from proteins destined for the cell surface [Citation4]. For this reason, control of the expression of proteins on the vacuolar membrane is believed to help regulate trafficking pathways into the vacuole. We construct the backbone vector with the purpose of expressing foreign proteins on the vacuolar outer membrane. Among a wide range of vacuolar membrane proteins, vacuolar membrane ATPase 11 (VMA11) was used to construct the vector that can express interest peptide on the outer membrane of the vacuole. VMA11 protein has an N- and C-terminal localised in the vacuolar lumen. Rearrangement of the VMA11 protein can exhibit the N- and C-terminal positions [Citation5,Citation6].
Toll-like receptor 2 (TLR2), a member of TLR family, is membrane protein on the cell surface. This receptor recognizes foreign pathogenic substrate and induces the immune signal in the cell [Citation7]. Enhanced TLR2 expression has been observed in a wide variety of tumours, suggesting that TLR2 may play important roles in tumorigenesis and tumour progression. In the case of acute myeloid leukaemia (AML), differential expression of TLR 2 has been observed in AML [Citation8].
In this study, the recombinant S. cerevisiae was constructed for the production of targeting peptide-expressed vacuoles for AML treatment. To increase drug delivery efficiency, AML selective binding peptides that are known to penetrate cells were used [Citation8]. After the isolation of AML-specific vacuoles, daunorubicin was encapsulated into recombinant vacuoles, and HL-60 cell were treated with vacuole drug carriers. Also, we evaluated the selectivity of the vacuole carriers to HL-60 cell and confirmed the improvement of anti-cancer efficiency.
Materials and methods
DNA isolation manipulation and transformation
Plasmid DNA, such as pYES2.0.0 (Invitrogen, US), and pEBFP-N1 (Clontech Laboratories, Inc., US), were prepared from E. coli cells using lysis technique with Exprep™ Plasmid SV kit (GeneAll, Korea). DNA modification, analysis by agarose gel electrophoresis, and ligation were performed using standard procedures. The PCR experiment was carried out using a T Gradient Thermocycler (Biometra, Germany), Ex Taq DNA polymerase (Takara Bio Inc., Japan), and chromosomal DNA as the template. The PCR products were purified using Expin™ PCR SV kit (GeneAll, Korea). The oligonucleotides used for PCR amplification were purchased from AcuuOligo (Bioneer Co., Korea). The transformation of E. coli (DH5-alpha) was performed by heat shock method, and yeast transformation was performed by lithium acetate method.
Construction of S. cerevisiae that produce the TLR2 binding peptide (T2BP) on the vacuolar outer membrane
The backbone vector, pYES2::SS::MCS::VMA11::His-tag, for the protein expression on the outer membrane of yeast vacuole was constructed. Briefly, the original vector pYES2 (Invitrogen, USA) is used as a platform to build the target vector that can express any protein of interest on the yeast vacuolar outer membrane. Different parts of polypeptide VMA11, including signal sequence fragment (SS) (consisting of the first 39 amino acids), Part 2 fragment (P2) (consisting of the last 46 amino acids) and Part 1 fragment (P1) (consisting of the next 79 amino acids), are in turn inserted into pYES2. The sequence of P1 and P2 has been reversed from the original sequence. The multi cloning site (MCS) is located between SS and P2. The TLR2 binding peptide (T2BP) and enhanced green fluorescence protein (GFP) were inserted in this site.
In order to treat AML more efficiently, TLR2 binding peptide, T2BP, which could specifically bind to TLR2, was cloned with the GAL1 promoter, allowing gene expression in the presence of galactose pYES2, and attached to EGFP following TLR2-binding eptide (T2BP), to confirm the expression of T2BP on the membrane of vacuole (named pYES2::SS::T2BP::GFP::VMA11::His). This T2BP was reported as a TLR2 targeting and penetrating peptide [Citation8]. Plasmid pYES2::SS::T2BP::GFP::VMA11::His-tag was constructed for the expression of T2BP with GFP, and the coding regions of T2BP gene were synthesised (Bioneer, South Korea), and EGFP gene amplified from the pEGFP-N1 by PCR using primer pairs SST2BP-F (5′ - GGCAAGCTTATGTCAACGCAA − 3′), SST2BP-R (5′ - ATAGGATCCCCACGGGCTCAC − 3′), GFP-F (5′ – ATAGGATCCATGGTGAGCAAG − 3′), and GFP-R (5′ – ATACTCGAGCTTGTACAGCTC − 3′). The PCR product of SS::T2BP was digested with HingIII/BamHI, and GFP was digested with BamHI/XhoI and then ligated into the restricted plasmid. S cerevisiae s2805 was transformed with pYES2::SS::T2BP::GFP::VMA11::His-tag to construct recombinant yeast. The empty vector pYES2 was transformed into S. cerevisiae s2805 to construct a control strain. Subsequently, pYES2.0::SS::T2BP::GFP::VMA11::His-tag was transformed into S. cerevisiae by lithium acetate method. lists the Plasmid, Escherichia coli and Saccharomyces cerevisiae strains used in this study.
Table 1. Strains and plasmids information used in this study.
Isolation of vacuoles from recombinant yeast
To extract vacuoles from the recombinant strains, they were cultured under the previously mentioned culture conditions. The yield of cells obtained was approximately 5 g/L. Tris-SO4 buffer was used for the complete removal of the cell walls. The 5 g of cell was mixed with 0.1 M Tris-SO4 buffer (100 mM Tris-SO4 (pH 9.4), 10 mM dithiothreitol (DTT)) and was incubated at 30 °C in a shaking incubator at 90 rpm for 15 min. After suspending the mixture, it was centrifuged at 3,000 rpm for 10 min, and the supernatant was discarded. The 5 g of cells was re-suspended in braking buffer (20 mM Tris-Cl (pH 7.4), 0.6 mM Sorbitol, 1 mM phenyl methane sulphonyl fluoride (PMSF)), to relax the membrane of cell. Glass beads were added to the cells in a mass ratio of 1:1. The mixtures were repeatedly vortexed, and kept in ice at intervals of 1 min on and off for a total 20 min. After the 10 min of stabilisation in the ice, centrifugation was carried out (500× g, 5 min). Supernatant was collected in microtube and was then centrifuged at 20,000× g for 30 min at 4 °C, and 1 g of vacuoles was obtained.
To quantify the vacuoles, we measured total proteins. The obtained vacuoles were strongly vortexed 10 times (10 s on/off) with lysis buffer pH 3.7 (0.1% NP-40, 5 mM DTT, and 0.1 mM PMSF) at a ratio of 1:1, and were reacted on ice for 30 min. All the obtained proteins were measured by Bradford assay (Bio-Rad, Richmond, CA, USA).
Confirmation of T2BP expression
To confirm the expression of foreign peptide, cell lysate was carried out. Mock and recombinant S. cerevisiae were grown in SD medium at 30 °C and 180 rpm for 24 h. To induce the GAL1 promoter of the pYES2 vector, the cells were grown to the exponential phase. After growth in SD medium, galactose was added at a concentration of 2%. The cell was grown in SG medium at 30 °C and 180 rpm for 4 h. The yeast was grown until log-phase, and it was harvested through centrifugation at 3,000 rpm for 10 min, discarding the supernatant, and washed 5 times with distilled water. Expression of foreign peptide that was inserted was confirmed by dot blot assay. To separate the vacuolar membrane proteins, isolated vacuoles were strongly vortexed 10 times (10 s on/off) with lysis buffer pH 3.7 (0.1% NP-40, 5 mM DTT, and 0.1 mM PMSF) at a ratio of 1:1, and put on ice for 30 min. After breakdown of vacuoles, lysates were centrifuged at 13,000 rpm for 15 min at 4 °C, and the supernatant was discarded. The separated vacuolar membrane proteins were quantified by Bradford assay. Dot bolt assay was carried out with 20 µg of vacuole that contained the foreign peptide, followed by 6X His-tag. Briefly, vacuoles were put on nitrocellulose (NC) membrane, and dried for 1 h. Blocking with blocking buffer (2% skin milk) was carried out for 30 min. The membrane was blotted with primer antibody (dilution 1:500, mouse monoclonal 6X His-tag antibody, Abcam, United Kingdom) for 2 h. After incubation with primary antibody, the membranes were washed 3 times with TBST (50 mM Tris-HCl, 150 mM NaCl, 0.1% Tween 20, pH 7.4), blotted with secondary antibodies (dilution 1:5,000, gt anti-ms IgG (H + L) secondary antibody, HRP conjugate, Invitrogen, USA). After that, membranes were incubated with ECL reagent (Amersham™, United Kingdom) and expose X-ray film in the dark room. The expression level of foreign peptide followed by 6X His-tag was analysed by ELISA at 450 nm wavelength (Multiskan ski ELISA reader, Thermo scientific, USA).
The expression of foreign peptide on vacuolar membrane was also observed by confocal laser-scanning microscopy (LSM 880 with Airyscan, Carl Zeiss, Germany) installed in the Centre for University-Wide Research Facilities (CURF) at Jeonbuk National University. Vacuolar membrane was stained by FM4-64 that can selectively stain yeast vacuolar membrane with red fluorescence.
Transmission electron microscope (TEM)
After isolation of vacuoles, the morphology of vacuole was confirmed by transmission electron microscopy (TEM). Isolated vacuoles ware put on the copper grid with carbon film for 10 min. Vacuoles were negatively stained with 1% uranyl acetate for a few seconds. TEM images were analysed by H-7650 (Hitachi, Japan) installed in the Centre for University-wide Research Facilities (CURF) at Jeonbuk National University. Microscopy was operated at 100 kV, and images were acquired at magnification of ×100,000 and ×200,000.
Nanoparticle tracking analysis (NTA)
To confirm the size distribution of vacuoles, nanoparticles tracking analysis was performed. The vacuoles were diluted 1:20,000 and the diluted vacuoles solution were injected into the Zetaview® (Particle Metrix GmbH, Germany).
Encapsulation of daunorubicin into vacuoles
Daunorubicin chloride was encapsulated by its penetration properties into isolated vacuoles. Briefly, 80 μg of daunorubicin hydrochloride was mixed with isolated vacuoles that contained 100 μg of total protein in l mL P·S buffer (10 mM Pipes·KOH (pH 6.8), 200 mM Sorbitol) at 4 °C. After loading, all samples were centrifuged at 13,000 rpm for 30 min. Unloaded supernatant was removed and loaded lysosome was washed with PS buffer for more than eight times.
Cell culture
The HL-60 cells were maintained in RPMI-1640 medium (Gibco) with 10% (v/v) foetal bovine serum (FBS, Gibco), 1X antibiotic antimycotic (AA, Gibco). And RAW 264.7, and B16F10 and HeLa cells were maintained in Dulbecco’s modified eagle medium (DMEM, Gibco) with 10% (v/v) foetal bovine serum (FBS, Gibco), 1X antibiotic antimycotic (AA, Gibco) in humidity air with 5% CO2 at 37 °C. When 80% of cell confluence was obtained, growing cell was washed with pre-warmed RPMI-1640 (10% FBS, 1X AA) medium, and resuspended at each concentration in RPMI-1640 (10% FBS, 1X AA) medium. Cell count was determined by using haemocytometer and trypan blue dye exclusion.
Selective binding to AML
To confirm the targeting ability of peptide, selectivity assay of T2BP to HL-60 cells was checked, in comparison with other cancer cell line (HeLa, B16F10) and macrophage (RAW 264.7). The cells (3 × 105/mL) were washed in 0.5% BSA in DPBS, and aliquoted in 6-well plate. Each cell was stained with 1 µM of targeting peptide-FITC. After exposure, cells were washed 3 times with DPBS, and fluorescence intensity and images were measured by fluorometry (GloMax explorer, Promega, USA) and fluorescence microscopy (Axio Scope A1, Carl Zeiss, Germany).
Cell binding and cellular uptake
To investigate the cell penetration of T2BP, fluorescence image of HL-60 cell was confirmed after peptides exposure. The 3 ml of HL-60 cells (2 × 105/mL) were aliquoted in 6-well plate. The 1 µM of T2BP-FITC was exposed in each well for (1, 3, and 6) h, respectively. After exposure, HL-60 cells were washed by Dulbecco’s phosphate buffer saline (DPBS, Gibco, USA), and nucleus was stained with DAPI. Fluorescence image was confirmed by fluorescence microscopy (Axio Scope A1, Carl Zeiss, Germany).
After confirming the cellular uptake of T2BP, binding affinity of T2BP-expressed vacuole (targeting vacuole) to HL-60 was investigated. The 3 ml of HL-60 cells (2 × 105/mL) were aliquoted in 6-well plate. The 1 µg/mL of encapsulated daunorubicin into normal and targeting vacuoles was exposed in each well for (30, 60, and 120) min, respectively. After exposure, cells (2 × 105/mL) were washed in DPBS and drug delivery, and cellular uptake was monitored by confocal laser-scanning microscopy (LSM 880 with Airyscan, Carl Zeiss, Germany) installed at the Centre for University-Wide Research Facilities (CURF) at Jeonbuk National University.
Cell viability assay
To confirm the anticancer effect of encapsulated daunorubicin, the cell mortality was measured by cell counting. The encapsulated daunorubicin was quantified by comparing the fluorescence. The crude daunorubicin, normal vacuoles, and targeting vacuoles with encapsulated daunorubicin were treated with HL-60 cells. The 180 µL of HL-60 cells (2 × 105/mL) were aliquoted in 96-well plate. The 20 µL aliquots of daunorubicin of (0.1, 0.5, 1.0, 5.0, and 10.0) µg/mL were exposed in each well. The cells treated with medium only served as negative control group. Cells were incubated in the humidified 5% CO2 incubator at 37 °C for (3 and 6) h, respectively. Incubation was followed by centrifugation at 1,000 rpm for 5 min. Supernatant of culture medium was discarded. Pellet of cells was washed 2 times with 200 µL of DPBS and resuspended with 50 µL of DPBS. Cell count was determined by using haemocytometer and trypan blue dye exclusion. Relative cell viability (%) was calculated as percentage relative to the untreated control cells.
Statistical analysis
For all the experiments, the data were obtained from three independent samples measured simultaneously for error analysis, and the results are shown with the standard deviation and the correlation with cell mortality under several experimental conditions. A P-value <0.05 was statistically significant. The data was analysed by using Sigma Plot (SPS Chicago, IL, USA).
Results
Expression of T2BP on vacuolar outer membrane in S. cerevisiae
Vacuolar membrane ATPase 11 (VMA11) is part of vacuolar ATPase and localized in the vacuolar membrane [Citation6]. Permutation of polypeptide that makes up the VMA11 takes N- and C-terminal out of the vacuolar outer membrane [Citation5]. To express the TLR2 specific binding peptide (T2BP) followed by N-terminal of VMA11, T2BP was cloned with the GAL1 promoter, allowing gene expression in the presence of galactose pYES2, and attached to GFP, followed by the pYES2::SS::T2BP to confirm the expression of T2BP on the vacuolar outer membrane. The recombinant plasmid, pYES2::SS::T2BP::GFP::VMA11::His, was transformed into S. cerevisiae by Heat shock. T2BP and GFP were induced by the GAL1 promoter with SG medium (2% D-galactose) at 30 °C for 24 h. After culture and induction, the expression of T2BP was confirmed. To check the expression of modified VMA11, dot blot assay (Figure S1) was carried out using 6X His-tag, followed by C-terminal of modified VMA11. Mouse monoclonal 6X His-tag antibody (Abcam, United Kingdom) was used to check for protein presence (). We could see darker spots than those of the wild type strains. In addition, when the relative intensity was compared through ELISA, more than 5 times intensity was confirmed. Also, we observed the green fluorescence signals that responded in recombinant yeast (). The signal of GFP indicated that T2BP was expressed on the vacuolar outer membrane. In ., (a) is green fluorescence of EGFP, (b) is yeast vacuolar membrane stained by FM4-64, (c) is yeast cell, and (d) is merged image.
Selective affinity and cellular uptake of decorated peptide on vacuolar membrane
Toll-like receptors (TLRs) are a family of pattern recognition receptors (PRRs) that sense a variety of pathogen-associated molecular patterns (PAMPs). TLR proteins play important roles in the host defense against pathogenic substrates. Normally, TLRs are responsible for the secretion of cytokines and signals that respond to the immune cells. In several reports, over expression or up-regulation of TLRs has been demonstrated in some tumours and cancer cell lines [Citation9,Citation10]. Indeed, enhanced expression of TL2 has been found in acute myeloid leukaemia. It is considered that selective therapeutics of TLR2 with targeting peptide are effective treatment for acute myeloid leukaemia [Citation8,Citation11].
To confirm the binding of T2BPs that were decorated on the vacuolar membrane, the position of T2BP-FITC in types of cancer and macrophage cells was investigated. The results show that weak signals were observed in the cells, except HL-60. HL-60 is related to acute myeloid leukaemia that revealed enhanced expression of TLR2 [Citation8], (). Higher signal of fluorescence was confirmed in HL-60 cell. Also, intensity of fluorescence was increased in HL-60 cell time dependently (). These results show that the targeting peptide is effective for specific cellular uptake to HL-60 cells.
Figure 3. Confirmation of TLR2 binding peptide (T2BP) on vacuolar membrane by confocal laser scanning microscopy. (a) GFP, (b) FM4-64, (c) yeast cells and (d) is merged images. Recombinant yeast (MBTL-WIC-1) with modified VMA11 protein followed by T2BP with GFP (green), Vacuolar membrane with FM4-64 (red), yeast cells and merged image.

Figure 4. Selectivity of T2BP was confirmed. (a) to (d) is representative fluorescence image of each cell (B16F10, HeLa, RAW 264.7, HL-60). (a) DIC, (b) Dapi, (c) T2BP-FITC, (d) is merged images (Scare bar, 5 µm).
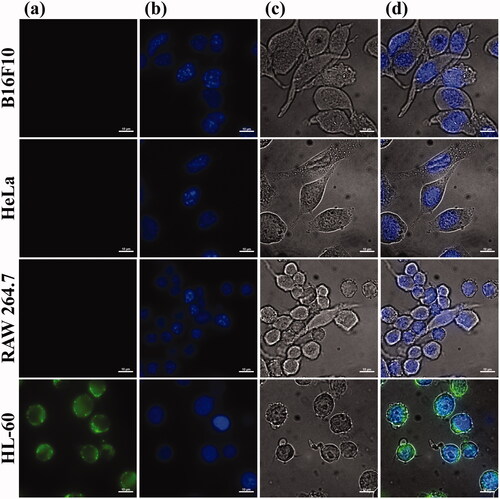
To confirm the cellular uptake of T2BP, fluorescence imagery was monitored (). After 1 h, it was confirmed that the T2BP-FITC was attached to the HL-60 cells. As the peptide exposure time increased, the number of fluorescent cells increased. According to these results, TLR2 specific binding peptide attached to HL-60 within an hour and was distributed in the cytoplasm.
Drug delivery and cellular uptake of targeting vacuole system to HL-60 cell
To enhance the drug delivery efficiency and specificity, T2BP was engineered on the vacuole drug delivery system. After confirming the selective affinity and cellular uptake of T2BP, drug delivery of targeting vacuole was monitored. Attachment of mock (a) and targeting (b) vacuole was confirmed in by CLSM. Also, in vitro cellular uptake was monitored according to time in . In , fluorescence of daunorubicin at little concentration could be observed, whereas in (b), the fluorescence of daunorubicin at higher signal was observed on the HL-60 cell surface. As a result of monitoring the cell adhesion of the targeting vacuole in , it was confirmed that the targeting vacuole was attached, uptake to the cell occurred, and daunorubicin was diffused into the cells.
Anticancer effect of targeting vacuoles system with encapsulated daunorubicin on HL-60 cells
After confirming the drug delivery of targeting vacuole system, the anti-cancer efficacy of the targeting vacuoles system with daunorubicin was confirmed.
The anthracycline antibiotics, such as daunorubicin and doxorubicin, are widely used in the treatment of leukaemia and solid tumours. These drugs show their effects by intercalation in the DNA double helix, thereby inhibiting replication and transcription. However, long-term treatment of anthracyclines is restricted by their serious, dose-dependent cardiotoxicity [Citation12].
To prevent the side effect of crude chemotherapeutics, the drug delivery system based on recombinant vacuoles was evaluated. After decoration with HL-60 cell targeting peptide, cell mortality was compared with free daunorubicin (DNR), nontargeting DNR loaded vacuoles, and targeting DNR-loaded vacuoles in . The results show that the cell mortality of targeting vacuoles with encapsulated DNR is clearly more efficient than the crude daunorubicin and nontargeting vacuoles. Also, the results show the synergetic effects of targeting vacuoles and daunorubicin. According to these results, the value of lethal concentration 50 (LC50) can be compared. LC50 of free daunorubicin (LC50 free DNR) is about 1 µg/mL for 6 h exposure. In comparison, LC50 of targeting vacuoles (LC50 targeting vacuoles) is lower than half of LC50 free DNR. This means that the amount of drug needed to kill the same amount of cancer cells is reduced to less than half. Comparison of the LC50 of nontargeting vacuoles (LC50 nontargeting vacuoles) and LC50 targeting vacuoles shows that targeting peptide improved the efficacy of drug delivery.
Discussion
Here, we construct the vacuole drug delivery system for selective AML therapeutics. Typically, components of nano drug delivery system are comprised of therapeutic agents and components that assemble with the therapeutic entities, such as lipid, polymers to form nanoparticles. Such nanoparticles can have enhanced anti-cancer effects compared with crude chemotherapy. This is due to more specific targeting to cancer via improved pharmacokinetics and dynamics [Citation13]. With the development of nanomaterials, numerous different types of strategy for selective therapeutics are reported [Citation14]. Also, various approaches for effective AML treatment have been reported. For example, regulation of bone marrow renin-angiotensin system is known to induce AML apoptosis. But some genomic subtypes of AML need conventional chemotherapy [Citation15]. To induce the cancer apoptosis, nanomaterials were applied for selective and intensive drug delivery for AML cells, which is a complementary approach to treatment strategy. Since many studies have already reported biomarkers according to cancer types, the use of peptides that specifically respond to these biomarkers is effective strategy. To develop AML selective vacuole drug delivery system, cell penetration peptide specifically binding AML cell lines was chosen from already screened novel targeting peptides [Citation8], and it was expressed on vacuolar outer membrane in . Also, selectivity and drug delivery of targeting vacuole system was confirmed in and . According to these results, targeting and drug delivery ability of vacuoles system were confirmed on not only multicellular solid tumour but also suspended blood cancer [Citation3].
Figure 8. Monitoring the cellular uptake of mock and targeting vacuoles with exposure time (for 30, 60 and 120 min). Red colour indicated the daunorubicin fluorescence (Scare bar, 10 µm).
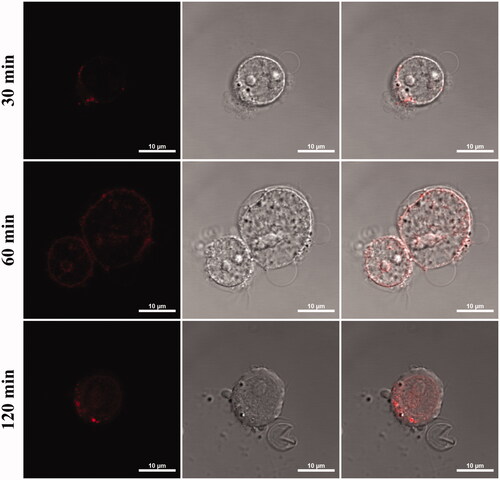
Figure 9. Cell mortality of HL-60 was investigated. HL-60 cells were treated with daunorubicin, non-targeting vacuoles and targeting vacuoles with encapsulated daunorubicin for 3(a) and 6(b) hours, respectively.
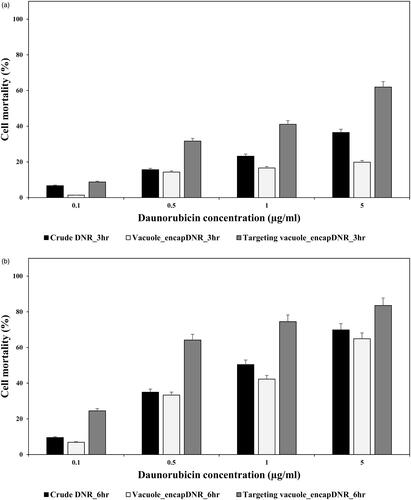
Yeast vacuoles correspond to lysosomes that is an intracellular organelle with acidic lumen. Release of hydrolytic enzymes of lysosomes to cytosol accompanies apoptosis signalling in several systems. It was confirmed that inducing the apoptosis of cancer cells by vacuolar enzymes derived from yeast in Figure S1. Enhanced apoptosis of cancer cell was confirmed by treatment of vacuole that overexpressed aminopeptidase Y, one of vacuolar enzyme [Citation16]. Lysosomotropic drugs such as doxorubicin, daunorubicin and other anthracycline drugs are lipophilic or amphiphilic compounds with a weakly basic pH and can be permeable and sequestered in vacuole [Citation17]. It was confirmed that the sequestered drugs were released out of vacuoles over time in previous study [Citation18]. Decreased apoptosis of HL-60 cells was confirmed with encapsulated daunorubicin in normal vacuoles in . It can be explained by release profile. Sequestered daunorubicin in acidic vacuole was non-ionized form and it diffuse back through lipid bilayer very slowly [Citation19]. But in targeting vacuole system, vacuoles can attach and release the drug directly to target cells. And also, these drug transfer into acidic compartments of cancer cell or tumour tissue. Such lysosomotropic behaviour was induced by targeting ability of vacuoles in . As a result, apoptosis of HL-60 cells was enhanced by not only lysosomotropic properties but also vacuolar enzymes. These synergies can be evaluated on economics of cancer drug. comparing the value of lethal concentration 50% (LC50), LC50 targeting vacuoles was lower than half of LC free DNR. This means that the amount of drug needed to kill the same amount of cancer cells is reduced by less than half. As a result, we conclude that the vacuole drug delivery system with disease selectivity offers potential application to cancer therapy.
Our studies demonstrate that yeast-derived vacuoles with targeting ability have the potential to be used as a bio-based drug delivery system. Also, this vacuole system provides potential strategies and a platform for disease selective treatment through simple manipulation. In this study, we evaluated the anticancer effect of vacuole drug delivery on acute myeloid leukaemia (AML). For a strategy to increase the selectivity of drug delivery, a representative biomarker of AML and peptide having a targeting ability against biomarker was investigated. After a literature survey, we constructed the vacuoles that contain targeting peptide, T2BP, on the vacuolar membrane. Daunorubicin, a kind of anthracycline, can be encapsulated in acidic vacuole lumen. To evaluate the efficacy of the vacuole drug delivery system, cellular uptake in vitro and cell mortality assay were conducted. Targeting vacuole engineered with T2BP that can be selectively attached to HL-60 cells showed a higher level of cellular uptake, compared to mock vacuole. HL-60 cells that were representative of AML were exposed to free daunorubicin (DNR), vacuoles with encapsulated DNR, and targeting-vacuoles with encapsulated DNR. The effect of selectivity could be confirmed through the difference in cell viability between the nontargeting vacuole and targeting vacuole. Through this study, drug delivery system using surface modified vacuoles might be suggested as selective therapeutics for AML treatment.
Supplemental Material
Download MS Word (583.1 KB)Supplemental Material
Download TIFF Image (2.5 MB)Supplemental Material
Download TIFF Image (6.6 MB)Supplemental Material
Download TIFF Image (19.8 MB)Supplemental Material
Download TIFF Image (6.3 MB)Supplemental Material
Download TIFF Image (6.3 MB)Supplemental Material
Download TIFF Image (6.3 MB)Supplemental Material
Download TIFF Image (6.3 MB)Disclosure statement
The authors declare no competing financial interest.
Data availability statement
The authors confirm that the data supporting the findings of this study are available within the article and its supplementary materials.
Additional information
Funding
References
- Yoo J-W, Irvine DJ, Discher DE, et al. Bio-inspired, bioengineered and biomimetic drug delivery carriers. Nat Rev Drug Discov. 2011;10(7):147–535.
- Li Z, Tan S, Li S, et al. Cancer drug delivery in the nano era: an overview and perspectives (Review). Oncol Rep. 2017;38(2):611–624.
- Gujrati V, Lee M, Ko YJ, et al. Bioengineered yeast-derived vacuoles with enhanced tissue-penetrating ability for targeted cancer therapy. Proc Natl Acad Sci USA. 2016;113(3):710–715.
- Armstrong J. Yeast vacuoles: more than a model lysosome. Trends Cell Biol. 2010;20(10):580–585.
- Kim BN, Ahn JY, Song MS, et al. Development of odorous gases reduction agents using recombinant Yeast-Derived extract. Toxicol Environ Health Sci. 2019;11(4):271–277.
- Flannery AR, Graham LA, Stevens TH. Topological characterization of the c, c', and c" subunits of the vacuolar ATPase from the yeast Saccharomyces cerevisiae. J Biol Chem. 2004;279(38):39856–39862.
- Takeda K, Akira S. Toll-like receptors in innate immunity. Int Immunol. 2005;17(1):1–14.
- Li K, Lv XX, Hua F, et al. Targeting acute myeloid leukemia with a proapoptotic peptide conjugated to a Toll-like receptor 2-mediated cell-penetrating peptide. Int J Cancer. 2014;134(3):692–702.
- Chen GY, Brown NK, Wu W, et al. Broad and direct interaction between TLR and siglec families of pattern recognition receptors and its regulation by Neu1. Elife. 2014;3:e04066.
- Rybka J, Butrym A, Wróbel T, et al. The expression of toll-like receptors in patients with acute myeloid leukemia treated with induction chemotherapy. Leuk Res. 2015;39(3):318–322.
- Gilad Y, Firer M, Gellerman G. Recent innovations in peptide based targeted drug delivery to cancer cells. Biomedicines. 2016;4(2):11.
- Paul C, Liliemark J, Tidefelt U, et al. Pharmacokinetics of daunorubicin and doxorubicin in plasma and leukemic cells from patients with acute nonlymphoblastic leukemia. Ther Drug Monit. 1989;11(2):140–148.
- Davis ME, Chen Z, Shin DM. Nanoparticle therapeutics: an emerging treatment modality for cancer. Nat Rev Drug Discov. 2008;7(9):771–782.
- Ståhl S, Gräslund T, Karlström AE, et al. Affibody molecules in biotechnological and medical applications. Trends Biotechnol. 2017;35(8):691–712.
- Ghasemi M, Okay M, Turk S, et al. The impact of At1r inhibition via losartan on the anti-leukaemic effects of doxorubicin in acute myeloid leukaemia. J Renin Angiotensin Aldosterone Syst. 2019;20(2):1470320319851310.
- Yoon J, Sekhon SS, Kim YH, et al. Enhanced lysosomal activity by overexpressed aminopeptidase Y in Saccharomyces cerevisiae. Mol Cell Biochem. 2016;417(1-2):181–189.
- Giraldo AMV, Appelqvist H, Ederth T, et al. Lysosomotropic agents: impact on lysosomal membrane permeabilization and cell death. Biochem Soc Trans. 2014;42(5):1460–1464.
- Choi W, Heo MY, Kim SY, et al. Encapsulation of daunorubicin into saccharomyces cerevisiae-derived lysosome as drug delivery vehicles for acute myeloid leukemia (AML) treatment. J Biotechnol. 2020;308:118–123.
- Gong Y, Duvvuri M, Krise JP. Separate roles for the golgi apparatus and lysosomes in the sequestration of drugs in the multidrug-resistant human leukemic cell line HL-60. J Biol Chem. 2003;278(50):50234–50239.