Abstract
We investigated the effect of green tea extract PEGylated gold nanoparticles (P-AuNPs) making use of its targeted and sustained drug delivery against cyclophosphamide (CYP)-induced cystitis. AuNPs were synthesized by reduction reaction of gold salts with green tea extract following the concept of green synthesis. Mostly spherical-shaped P-AuNPs were synthesized with an average size of 14.3 ± 3.3 nm. Pre-treatment with P-AuNPs (1, 10 mg/kg, i.p.) before CYP (150 mg/kg, i.p.) challenge suggested its uroprotective properties. P-AuNPs significantly reversed all pain-like behaviours and toxicities produced by CYP resulting in a decreased aspartate aminotransferase, alanine aminotransferase, C-reactive protein, and creatinine level. P-AuNPs increased anti-oxidant system by increasing the level of reduced glutathione, glutathione-S-transferase, catalase and superoxide dismutase, and reduced nitric oxide production in bladder tissue. Additionally, it attenuated hypokalaemia and hyponatremia, along with a decrease in Evans blue content in bladder tissue and peritoneal cavity. CYP-induced bladder tissue damage observed by macroscopic and histological findings were remarkably attenuated by P-AuNPs, along with reduced fibrosis of collagen fibre in bladder smooth muscles shown by Masson’s trichrome staining. Additionally, alterations in hematological parameters and clinical scoring were also prevented by P-AuNPs suggesting its uroprotective effect.
Introduction
Cyclophosphamide (CYP) is a cytotoxic alkylating agent, belonging to oxazophorine group having reported efficacy in numerous human malignancies [Citation1]. Detrimental toxicities such as immunosuppression and haemorrhagic cystitis are associated with this anti-cancer agent reducing its clinical usefulness [Citation2,Citation3]. Numerous therapeutic agents have been evaluated experimentally and clinically against CYP-induced cystitis, but none has been proved to have complete protective effect. Clinically, sodium-2-mercaptoethane sulphonate (mesna) is used to detoxify acrolein; however, dose-dependent side-effects still occur with its use. Therefore, in order to enhance the effect of CYP and inhibition of cystitis along with reduction in bladder pain, there is a need for the development of a potential alternative therapy for cystitis. In the current study, gold nanoparticles (AuNPs) have been prepared owing to its diverse biomedical applications such as photothermal therapy, photodynamic therapy, drug delivery, X-ray imaging, and sensing [Citation4]. The synthesis of AuNPs is simple, its optical and physicochemical properties contribute to diverse nanotechnology applications. For the synthesis of AuNPs, green synthetic strategy using plant extract have attracted researchers’ attention due to sustainability initiatives [Citation5,Citation6].
CYP is a pro-drug metabolized from inactive cytostatic agent to its active metabolites phosphoramide mustard and acrolein mostly in the liver by CYP450. Acrolein is considered to be a major cause of CYP-induced cystitis, it has been proven to be a potent urothelial irritant causing edoema, haemorrhage, ulceration, and necrosis [Citation7]. CYP has been observed to increase the oxidative stress during the process of bio-activation by producing reactive oxygen species (ROS) that results in the modification of both normal and neoplastic cells leading to reduced anti-oxidant capacity [Citation8,Citation9]. The pathogenesis of cystitis induced by CYP consists of various free radicals i.e. ROS and reactive nitrogen species as by-products [Citation10]. Naturally present anti-oxidant defense system in humans and other organisms gets compromised in disease conditions resulting in the lack of protection against oxidative damage [Citation11,Citation12]. Inflammation plays a crucial role in the regulation of oxidative stress, leading to the generation of ROS [Citation13,Citation14]. Nitric oxide (NO) a free radical synthesized by inducible nitric oxide synthase is considered to be a key executor of toxicity in oxidative stress [Citation15,Citation16]. CYP dramatically increases bladder inflammation, induces abdominal mechanical hypersensitivity, an indicator of bladder pain and increased visceral pain behaviours indicated by piloerection, abnormal posture, and a reduced rate of respiration [Citation17–19].
Green tea is a famous beverage worldwide, made from the leaves of Camellia sinensis belonging to the family Theaceae [Citation20]. Throughout the world about 2.5 million tons of tea leaves are produced each year, about 20% as green tea. Although it is mainly consumed in Asia its production and manufacture has been spread to some parts of North Africa, Europe, and the United States [Citation20]. Green tea has been known to contain beneficial compounds to human health. Phenolic compounds are major constituent including (+)-catachin, (–)-epicatachin, (–)-epigallocatechin gallate. Besides phenolic compounds, tannins and alkaloids were also reported to be in green tea [Citation21,Citation22]. Green tea extract has been previously reported to have strong anti-inflammatory and anti-oxidant properties [Citation21]. It is found to be involved in the reduction of oxygen free radicals associated with various chronic diseases, such as neurodegenerative diseases, cancers, and bladder tumour inhibition in various animal models and clinical trials [Citation21, Citation23]. The present study is a continuation of our previously published work in which green tea extract was used to perform a reduction reaction of gold (Au) salts to AuNPs via a green strategy [Citation24]. The aim of the study was to evaluate the effect of P-AuNPs against CYP-induced cystitis characterized by mechanical sensitivity, visceral pain, and oxidative stress.
Materials and methods
Materials
Chloroauric acid trihydrate (HAuCl4·3H2O), sodium hydroxide and PEG-SH (6 kDa) were purchased from Sigma-Aldrich (St. Louis, MO, USA). CYP, Griess reagent, 1-chloro-2, 4-dinitrobenzene (CDNB), hydrogen peroxide (H2O2), and 5, 5′-dithiobis-2-nitrobenzoic acid (DTNB) were also purchased from Sigma-Aldrich. All other reagents along with normal saline were of analytical grade.
Instruments
Observation of surface plasmon resonance (SPR) was conducted by acquiring UV–visible spectra on a Shimadzu UV-2600 spectrophotometer in a quartz cuvette (Shimadzu Corporation, Kyoto, Japan). A Varian 640 IR was used to acquire Fourier transform infrared (FT-IR) spectra (Agilent Technologies, Santa Clara, CA, USA); the sample measured was prepared by the KBr disc method. Dispersion state, size, and morphology of nanoparticles were observed by obtaining high-resolution transmission electron microscopy (HR-TEM) images on JEM-3010 operated at 300 kV (JEOL, Tokyo, Japan). Samples were pipetted onto a carbon-coated copper grid (carbon type-B, 300 mesh, Ted Pella, Redding, CA, USA) and air-dried at ambient temperature for 24 h. For centrifugation, a Centrifuge 5424 R (Eppendorf AG, Hamburg, Germany) was used. For freeze-drying, an FD8518 (IlshinBioBase Co. Ltd, Gyeonggi, Republic of Korea) was utilized. For the measurement of Au concentration of P-AuNPs, inductively coupled atomic emission spectrometer (ICP-AES) was employed (ACTIVA-S, HORIBA Jobin-Yvon, Longjumeau, France). Hydrodynamic size, zeta potential, and polydispersity index were recorded with a NanoBrook 90 Plus Zeta analyser (Brookhaven Instruments Corporation, Holtsville, NY, USA).
Green synthesis of AuNPs using green tea extract
Green tea leaves were a kind gift from the Institute of Hadong Green Tea (Hadong, Gyeongnam, Republic of Korea). The green tea extract was carefully prepared according to our previous report [Citation24]. Green synthetic process of P-AuNPs was also described in our previous report [Citation24]. Briefly, the following three materials which were dissolved in deionized water were mixed in a glass vial; the extract (final concentration of 0.03%), chloroauric acid trihydrate (final concentration of 0.5 mM), and sodium hydroxide (final concentration of 1 mM) was added. The final volume was adjusted to 2 mL by adding deionized water. Then, the mixture was vortexed for 2 s and incubated in a dry oven for 2 h at 80 °C. UV–visible spectra were acquired in a range of 300–800 nm to investigate SPR of P-AuNPs. In order to obtain the information about the functional groups that contributed in the synthesis of P-AuNPs, FT-IR spectral analysis were performed. We compared the FT-IR spectra of P-AuNPs with the spectrum of the extract obtained from our previous study [Citation24].
PEGylation of AuNPs and measurement of Au amount in P-AuNPs by using ICP-AES
The stock solution of PEG-SH was prepared at a concentration of 20 mM with deionized water. As prepared AuNPs (1 mL) in the previous section was mixed with PEG-SH (26 μL). Then, the mixture was stirred in the dark with 750 rpm at ambient temperature. After the completion of a 30 min reaction, the reaction mixture was stored in a refrigerator (4 °C) for 24 h. Next, centrifugation of the reaction mixture was performed (14,000g, 20 °C, 35 min) to remove unreacted PEG-SH. The pellet was recovered and freeze-dried, and this sample was used for in vivo experiment. Measurement of Au amount is important for in vivo applications. Thus, the supernatant was submitted to ICP-AES analysis in order to measure Au amount of P-AuNPs. Colloidal stability of P-AuNPs was assessed by dispersing nanoparticles in PBS buffer (pH 7.4). Then UV-visible spectra were acquired in a range of 300–800 nm.
Experimental animals
Albino male mice (BALB/c) weighing 28–30 g, 8-10 weeks of age were purchased from National Institute of Health (NIH), Islamabad. Animals were housed in the animal facility of Pharmacy Department, Quaid-i-Azam University (QAU), Islamabad. They were kept at 25 ± 2 °C temperature and a relative humidity of 60 ± 10% with a 12-h light/dark cycle. Bedding was changed every day. All mice had access to standard chow and water ad libitum. All the experiments were performed in a pathogen-free environment between 8 a.m. and 4 p.m. This study was approved by the Bioethical Committee of QAU, Islamabad with an approval No. BEC-FBS-QAU ONL-2020-002.
Experimental groups and treatment
To establish acute cystitis, mice were induced intraperitoneally (i.p.) with CYP at a final dose of 150 mg/kg, as described in a previous study [Citation25]. Ibuprofen (100 mg/kg; p.o.) given prior to CYP-induction served as a positive control group [Citation25–27]. Separate group of mice were treated with P-AuNPs (1 and 10 mg/kg, i.p.) dissolved in normal saline, administered prior to CYP treatment.
The animals were randomly divided into five separate groups (n = 14 mice per group);
Group I: Vehicle-control group (normal saline, i.p.)
Group II: CYP-treated group (150 mg/kg CYP, i.p.)
Group III: Ibuprofen-treated group (100 mg/kg ibuprofen, p.o.)
Group IV: Treatment I (1 mg/kg P-AuNPs, i.p.)
Group V: Treatment II (10 mg/kg P-AuNPs, i.p.)
Experimental design and sample collection
Experiments were performed in a well-illuminated and temperature controlled (20–22 °C) room. Behavioural analysis consisted of the assessment of pain parameters and clinical scoring at different time points as previously reported [Citation28]. For the evaluation of the intensity of the evoked cystitis biochemical and histological analysis were performed, mice were killed by cervical dislocation at 4 and 48 h of CYP administration. Blood was collected and bladder tissue was isolated immediately, wet-tissue weight was assessed as a measure of vascular and bladder wall permeability followed by the development of cystitis [Citation29]. Some of the bladder tissues were fixed in 4% paraformaldehyde for histological analysis, while others were stored at –80 °C for further biochemical assays. Urine samples were collected from the metabolic chamber for the last 24 h before killing the mice at 48 h ().
Behaviour parameters
Spontaneous pain
Immediately after the administration of CYP spontaneous pain was observed in mice by placing them in cages without saw dust bedding and were videotaped for 4 h [Citation30]. Mice were observed for 2 min after every 30 min up to 4 h, their behaviour was coded according to the following scale; 0= normal, 1= piloerection, 2= strong piloerection, 3= laboured breathing, 4= licking of the abdomen, and 5= contraction and stretching of the abdomen [Citation31]. If at one observation period more than one of these scores were noted, the sum of the corresponding point was assigned. An overall score was obtained by summing the scores assigned at each observed time point [Citation31,Citation32].
Mechanical nociceptive parameters
Bladder pain-like nociception and referred hyperalgesia in mice were determined as described previously, [Citation28,Citation33,Citation34] with minor modifications. Each animal was placed individually in a six-chambered plastic box with a metal grid floor and were left to adapt to the surrounding environment for a duration of 30 min for 2 days before starting the experiment. Nociceptive threshold was measured with von Frey fibres, whenever a positive response like licking and biting of skin of the lower abdomen close to the bladder were noted, the next smaller filament was applied. Otherwise, the next higher was used at different time points throughout the experiment.
Referred hyperalgesia was evaluated by using the response to von Frey filaments with increasing bending forces 1.4, 2, 4, 6, 8, and 10 g. The von Frey filaments were applied to the lower abdomen near the urinary bladder of the mice, each filament was applied three times for 1–2 s every 5 s apart in an ascending order of forces. In order to avoid learning or sensitization, care was taken not to stimulate the same location twice. Response to a particular stimulus was coded according to a scale from 0 to 3; 0= no reaction, 1= retraction of the abdomen, 2= change of position/or jump, 3= change of position/or jump along with licking of the area stimulated by filament/or vocalization [Citation25]. The nociceptive threshold and cumulative nociceptive scores were assessed at the basal point (0 h before any treatment) and at 2, 4, 6, 24, and 48 h after the induction of cystitis. Nociceptive scores were considered as the von Frey filament for which first score 1 was obtained. Whereas the mean cumulative nociceptive score was given as a sum of three applications of the same strength filaments.
Clinical scoring
Animals were assessed throughout the experiment to record clinical symptoms of distress. These symptoms were scored 0–3, by considering changes in a dull/ruffled coat, changes in temperament, and reluctance to move. For dull/ruffled coat (0= shiny coat, 1= coat raised around neck only, 2= coat raised around neck and belly, 3= coat raised around entire animal), change in temperament (0= normal, 1= agitated, 2= stress marks on eyes, nose and paws, 3= stress marks with hunching), and reluctance to movement (0= movement on the lift of lid, 1= movement when the hand is placed near mice, 2= movement only after picking up mice, 3= no movement which handling mice) scoring was performed according to previous study [Citation35,Citation36]. The total score for all criteria was averaged at each time point.
Biochemical parameters
Evaluation of vascular permeability in the bladder tissue
After the intravenous (i.v.) administration of 1% Evans blue (10 mL/kg, b.w.) dissolved in normal saline, the mice received i.p. CYP (150 mg/kg), vehicle or P-AuNPs (1 and 10 mg/kg) as described previously [Citation37]. The mice were sacrificed after 48 h of CYP induction by cervical dislocation and the bladders were removed. Normal saline (8 mL) was injected into the peritoneal cavity, and washing fluid was collected and allowed to stand overnight at 4 °C [Citation37]. The bladder tissues processed for the determination of Evans blue content were weighed and dried at 60 °C for 24 h, dried tissue was weighed again to estimate the lost water content considered as an indication of tissue edoema [Citation31]. The bladder tissue were incubated in formamide solution for 24 h at 70 °C. The absorbance of the supernatant was evaluated at 620 nm with a spectrophotometer (UV-1800 Shimadzu, Japan) [Citation37].
Measurement of reduced glutathione
Determination of glutathione (GSH) in bladder tissue homogenate was made by Ellman’s reaction according to the method adopted from Moron et al. [Citation38]. A portion of the bladder tissue homogenate was precipitated with 5% trichloroacetic acid, centrifuged for 10 min at 1000 rpm and supernatant was separated. Freshly prepared DTNB in 0.2 M sodium phosphate buffer (pH 8.0) reacted with the supernatant and produced yellow colour that was detected by spectrophotometer at 412 nm [Citation39–41]. The activity was expressed in percentage.
Measurement of anti-oxidant enzymes
The bladder tissue homogenate was centrifuged at 1000 rpm for 10 min at 4 °C, the supernatant obtained was used further for the assay of superoxide dismutase (SOD), glutathione-S-transferase (GST), and catalase (CAT) activity. The GST activity was measured according to the previously reported method by Warholm et al. [Citation42,Citation43]. Change in absorption was measured after the addition of CDNB in the reaction mixture containing glutathione as a co-substrate and the sample/supernatant recorded at 340 nm by spectrophotometer [Citation44,Citation45]. The results were expressed in percentage.
SOD activity was determined by using the method of Marklund et al. [Citation46]. The reaction mixture was composed of 50 mM Tris-EDTA buffer (pH 8.5), 24 mM pyrogallol, and 10 µl of the supernatant/sample in a final volume of 0.2 mL. The enzyme activity was calculated at 420 nm and was expressed in percentage. Furthermore, CAT activity was obtained from the rate of H2O2 decomposition upon the addition of sample/supernatant at 240 nm according to the method explained by Aebi et al. [Citation47–49]. The enzyme activity was expressed in percentage.
NO content determination
NO content in the samples was measured by the colorimetric Griess method described by Miranda et al. [Citation50,Citation51]. NO level was measured in plasma and supernatant obtained from the bladder tissue homogenate. A total of 100 µL of the sample and 100 µL of the Griess reagent were incubated for 30 min at room temperature. The absorbance was obtained at 560 nm on a microplate reader and the quantification was measured from a standard curve obtained from different concentrations of sodium nitrite [Citation52–54].
Macroscopic bladder evaluation and wet weight determination
The mice were sacrificed at 4 and 48 h after CYP administration, the macroscopic examination of the bladder was done on the basis of previously established criteria by Gray et al. [Citation55]. All the harvested bladders were made free of connective tissue and were evaluated macroscopically by an examiner blind to the experimental groups. Each bladder was then evaluated for edoema, which was classified as 3= severe (when fluid was visible externally and internally in the walls of the bladder), 2= moderate (edoema confined to the internal mucosa), 1= mild (slight edematogenic signals were observed), and 0= absent (absence of fluid/normal bladder). Additionally, wet weight of the bladder was also observed and expressed as bladder weight/body weight (mg/g). Upon examination bladder was also evaluated for bleeding in the walls, haemorrhage was scored 0–3, 3= presence of intravesical clots, 2= mucosal haematomas, 1= dilation of the bladder vessels, 0= absent/normal bladder.
Histological examination
Bladder tissues were harvested immediately after the animals were sacrificed and fixed in 10% buffered formalin (pH 7.4) for haematoxylin and eosin (H&E) and Masson’s trichrome staining [Citation56]. The specimens from all groups were examined under light microscope and were photographed. Histological evaluation was done without any prior knowledge of treatment groups and were scored according to the following criteria: 0= normal epithelium/absence of inflammatory cell infiltration and ulceration, 1 = mild changes/diminished epithelial cells, flattening with submucosal edoema, few ulceration and mild haemorrhage, 2 = severe changes/mucosal erosions, fibrin formation, inflammatory cell infiltration, and multiple ulcerations [Citation3]. The Masson’s trichrome staining was performed to evaluate the fibrosis and collagen accumulation in bladder tissue followed by CYP administration [Citation57,Citation58].
Urinary potassium and sodium level
Urine samples of last 24 h were collected from metabolic cages and were processed for the determination of urinary sodium (Na+) and potassium (K+) level in mmol/L. The aliquots of urine were stored at –80 °C and thawed before analysis. The Na+ and K+ level was determined with automated chemical analyser (Audit Tecno 786, Ireland) using the standard method [Citation59].
Hematological parameters
The hematological analysis was done to assess the pharmacological effect of P-AuNPs on CYP-induced hematological changes [Citation55, Citation58]. Approximately 1 mL of blood was collected from each mice in heparin EDTA tube, from which a small drop was collected for the smear evaluation using Giemsa staining. Differential cell count (white blood cells [WBCs], platelets, neutrophils, lymphocytes, monocytes, and eosinophils) were determined under a 40× magnification, by counting 100 cells.
Liver and kidney profile
The liver and kidney profile were estimated by determining the concentration of serum enzymes like aspartate aminotransferase (AST), alanine aminotransferase (ALT), and creatinine respectively, along with C-reactive protein (CRP) as a marker of systemic inflammation. The blood was collected from all groups i.e. saline-treated group, CYP-challenged group, and P-AuNPs (1 and 10 mg/kg) treatment group [Citation60]. The blood samples were kept at room temperature for 2 h, centrifuged at 3000 rpm for 10 min at 4 °C to obtain serum. The ALT, AST and creatinine levels in the serum were quantified by using MERK kit with the help of semi-automated analyser (Microlab 300, Merck) [Citation61–64], while CRP level was determined by using CRP ELISA kit.
Statistical analysis
For the statistical analysis, one-way analysis of variance along with bonferroni post hoc test was performed. The results obtained were expressed as mean ± SD and (p < .05) served as criteria for significance from the negative control. For the analysis of data SigmaPlot version 12.5 was used.
Results
UV–visible spectra of P-AuNPs
In our previous report, spherical-shaped AuNPs that were green-synthesized with green tea extract showed their characteristic SPR at 532 nm [Citation24]. After PEGylation, P-AuNPs induced a bathochromic (or red) shift at 538 nm (, red line). Chitosan capping of AuNPs also induced a bathochromic shift at 537 nm [Citation24]. When these P-AuNPs were mixed with PBS buffer, there was a blue shift of 10 nm. Although the absorbance decreased, SPR was clearly observed at 528 nm.
Figure 2. (A) UV–visible spectra of P-AuNPs. (B) A size histogram of P-AuNPs. Two-hundred seventy-five nanoparticles from the images were randomly selected for measuring an average size. (C) FT-IR spectra of a PEGylated AuNP compared to the previously reported green tea extract used for synthesis [Citation24].
![Figure 2. (A) UV–visible spectra of P-AuNPs. (B) A size histogram of P-AuNPs. Two-hundred seventy-five nanoparticles from the images were randomly selected for measuring an average size. (C) FT-IR spectra of a PEGylated AuNP compared to the previously reported green tea extract used for synthesis [Citation24].](/cms/asset/0a550bae-852d-41b4-b63f-1f1943cf11f2/ianb_a_2078340_f0002_c.jpg)
FT-IR spectra of P-AuNPs
In our previously reported study, green tea extract showed their major functional group at 3255 cm−1 representing –OH stretching vibration, most likely involved in the reduction reaction of Au salts [Citation24]. As shown in , upon synthesis of the P-AuNPs, this peak was shifted to a higher wavenumber at 3407 cm−1 suggesting that –OH groups were involved in the synthesis. The appearance of C=O functional groups at 1716 cm−1 in P-AuNPs supported the oxidation of –OH groups during the synthetic process represented in . Furthermore, the peaks at 2882 cm−1 and 1100 cm−1 indicated CH2 stretching and C–O stretching vibrations of PEG. These results demonstrated that the PEGylation was successfully proceeded to produce P-AuNPs.
HR-TEM images and a size histogram of P-AuNPs
HR-TEM images were acquired upon re-dispersion of P-AuNPs after centrifugation (). Mostly spherical-shaped P-AuNPs showed excellent dispersion. It has been known that PEGylation increases stability of nanoparticles along with biocompatibility. Furthermore, from careful examination of Figure3(C) and (D), lattice fringes were obviously observed. HR-TEM images of P-AuNPs were compared with those of bare AuNPs (i.e. without PEGylation) published in our previous report [Citation24]. We were able to observe clear lattice fringes in both bare AuNPs and P-AuNPs. This result indicated that a crystalline nature of nanoparticles was retained after PEGylation. We randomly selected 275 nanoparticles from the images, and the average size was measured as 14.3 ± 3.30 nm. A size histogram was demonstrated in . The most abundant size was 10–15 nm (60.7%) followed by 15–20 nm (27.6%).
Hydrodynamic size, zeta potential, and polydispersity index of P-AuNPs
In our previous report, the hydrodynamic size and zeta potential of bare AuNPs (without PEGylation) were observed as 28.4 nm and −12.73 mV, respectively [Citation24]. After PEGylation, the hydrodynamic size, zeta potential, and polydispersity index of P-AuNPs were determined to be 147.4 nm, 0 mV, and 0.297, respectively. The increase of hydrodynamic size from 28.4 nm to 147.4 nm, and the decrease of zeta potential from −12.73 mV to 0 mV proved bare AuNPs were successfully coated with a PEG neutral layer to produce P-AuNPs.
Au concentration of P-AuNPs by ICP-AES analysis
In order to determine the amount of Au injected into each mouse, ICP-AES was performed to measure the Au concentration in P-AuNPs via an indirect method. After P-AuNPs preparation, the colloidal solution was centrifuged, the supernatant was recovered and submitted to ICP-AES analysis. The Au concentration was determined to be 19.4 μM (for 1mg/kg) and 194.0 μM (for 10 mg/kg). Furthermore, the average Au amount that was injected into each mouse was also calculated i.e. 1.15 μg Au (for 1 mg/kg) and 11.5 μg Au (for 10 mg/kg).
P-AuNPs reduces nociception in cystitis
Nociceptive responses were measured at different time points (0, 2, 4, 6, 24, and 48 h) by von Frey filaments after intraperitoneal CYP injection. CYP displayed a remarkable decrease in nociceptive threshold as compared to saline-treated vehicle-control group shown in . Whereas P-AuNPs (1 mg/kg) remarkably (p < .001) improved the nociceptive threshold as compared to the CYP-treated group. Referred hyperalgesia suggested increased cumulative nociceptive scores observed in CYP-treated animals, P-AuNPs (1 mg/kg) remarkably (p < .001) decreased the nociceptive score in response to increasing strength of von Frey filaments as compared to CYP-challenged group shown in . The pronociceptive effect of CYP was assessed from 2 h of its administration up to 48 h (), compared to vehicle-control group. Pre-treatment with P-AuNPs at a dose of 1 mg/kg remarkably prevented the rise in nociceptive responses due to CYP administration. This reversal was experienced throughout the experiment at different time points corresponding with an increase in mechanical threshold and a reduced cumulative nociceptive score (). While 10 mg/kg of P-AuNPs did not produce significant anti-nociceptive effect as compared to CYP-challenged group.
Figure 4. Effect of intraperitoneal injection of P-AuNPs on CYP-induced bladder pain (A) Nociceptive threshold (g), (B) Refered hyperalgesia (Mean cumulative nociceptive score) at different time points. (C–G) Effect of P-AuNPs on nociceptive scores in response to mechanical stimuli at different time points i.e. 2 h, 4 h, 6 h, 24 h, and 48 h, plotted as cumulative score against von Frey forces (g). Data represented mean ± SD. *p < 0.05 and ***p < 0.001 vs. CYP-treated group. (###) comparison between CYP-treated group and vehicle-control group.

P-AuNPs reduces macroscopic changes
CYP-induced cystitis was observed at 4 and 48 h following CYP administration indicating macroscopic appearance of severe edoema with a score of 2, 2.8 and extensive haemorrhage receiving a score of 2.6, 2.3 at 4 and 48 h respectively, remarkably (p < .001) distinct from the saline-control group with a score of 0 for edoema and haemorrhage (). Pre-treatment with P-AuNPs (1 mg/kg) significantly decreased edoema receiving a score of 0.3, 0.3 and haemorrhage with a score of 0.3, 0.6 at 4 and 48 h respectively, compared to the CYP-treated group shown in . However, P-AuNPs (10 mg/kg) showed no significant decrease in edoema and haemorrhage compared to the CYP-treated group.
Figure 5. (A) Effect of P-AuNPs on macroscopic changes induced after CYP administration. Representative images of (a) vehicle control, (b) CYP-treated group, (c) ibuprofen (100 mg/kg), (d) P-AuNPs (1 mg/kg), and (e) P-AuNPs (10 mg/kg) bladder tissue at 4 and 48 h time points. (B) Effect of P-AuNPs on CYP-induced spontaneous pain in mice. Time course of behavioural scores assigned at 30 min interval plotted as cumulative score over 4 h post-injection period. (C) Effect of P-AuNPs on clinical scoring in mice treated with CYP. Time course of behavioural scores assigned throughout the experiment. Data represented mean ± SD. **p < .01 and ***p < .001 vs CYP-treated group. (###) comparison between CYP-treated group and vehicle-control group.
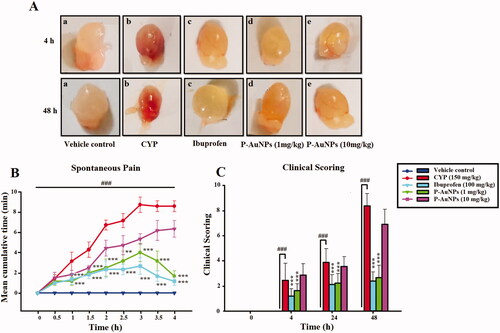
Table 1. Table representing weight (mg/g) and macroscopic scoring of bladder tissues at 4 and 48 h post CYP administration.
P-AuNPs attenuates spontaneous pain and improves clinical scoring
Assessment of spontaneous nociception showed a higher score in the CYP-treated group throughout the 4 h period. However, P-AuNPs (1 mg/kg) attenuated the spontaneous pain in comparison to the CYP-treated group as represented in . Similarly, ibuprofen used as a positive control group also reversed the spontaneous nociception compared to the negative control group. While P-AuNPs (10 mg/kg) was not effective against the spontaneous pain produced by the administration of CYP (). The clinical scoring was carried out for all the recruited groups. The saline-treated group exhibited no clinical symptom of distress with smooth fur, and no changes in movement shown in . However, CYP-treated animals showed highest clinical scores of distress as compared to vehicle control, they had decreased activity and hunched back along with stress marks. All these clinical symptoms were significantly (p < .001) improved by P-AuNPs (1 mg/kg) compared to the CYP-alone-treated group (). While P-AuNPs (10 mg/kg) showed signs of toxicity displaying decreased activity, hunched backs along with stress marks in CYP-treated mice as shown in .
P-AuNPs increases anti-oxidant status and reduces nitrite production
The bladder anti-oxidant status, such as GSH, GST, CAT, and SOD were significantly decreased in the CYP-treated group compared to the saline-control group at 4 and 48 h. The administration of P-AuNPs was found to significantly (p < .001) elevate the diminished activity of GSH (), GST (), CAT (), and SOD () resulting in scavenging of free radicals generated as a result of CYP-challenge at 4 and 48 h. CYP administration remarkably increased the NO production in bladder tissue homogenate and plasma compared to the saline-treated group. While P-AuNPs (1 mg/kg) significantly (p <.001) ameliorated the NO production in bladder tissue and plasma in comparison to the CYP-treated group. Additionally, the ibuprofen-treated group also reduced NO production as shown in .
Figure 6. Effect of pre-treatment of P-AuNPs on anti-oxidant proteins and enzymes in bladder tissue of mice after CYP treatment at 4 and 48 h. (A) GSH (%), (B) GST (%), (C) Catalase (%), and (D) SOD (%). (E) Effect of P-AuNPs on nitrite production in bladder tissues and plasma at 4 and 48 h after CYP administration. Data represented mean ± SD. **p < .01 and ***p < .001 vs. CYP-treated group. ###Comparison between CYP-treated group and vehicle-control group.
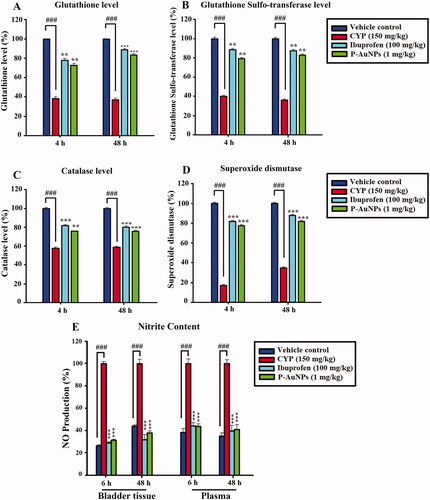
P-AuNPs reduces changes in vascular permeability in the bladder tissue
The CYP-induced increase in Evans blue extravasation was significantly (p < .001) decreased by P-AuNPs (1 mg/kg) indicated by a reduced Evans blue content in bladder tissue ()) and peritoneal cavity () compared to the CYP-treated group shown in . While treatment with P-AuNPs (10 mg/kg) showed no significant decrease in Evan blue content in bladder and peritoneal cavity compared to the CYP-treated group. Additionally, the wet weight of the bladder tissue was maximum for CYP (150 mg/kg), which was remarkably different from the weight of the P-AuNPs (1 mg/kg) and ibuprofen-treated groups as shown in , indicating reduced edoema and confirming the data obtained with Evans blue content.
Figure 7. Effect of P-AuNPs on Evans blue extravasation induced by CYP injection. (A) Photograph representing bladder tissue treated with Evans blue, (a) CYP-treated group, (b) ibuprofen (100 mg/kg), (c) P-AuNPs (1 mg/kg), and (d) P-AuNPs (10 mg/kg). Vascular permeability indicated as (B) Evans blue content µg/mg of bladder tissue and (C) Evans blue content µg/mL of peritoneal fluid. (D) Effect of P-AuNPs on bladder wet/dry weight (mg/g) in animals treated with CYP and Evans blue. Data represented mean ± SD. *p < .05 and ***p < .001 vs. CYP-treated group. ###Comparison between CYP-treated group and vehicle-control group.
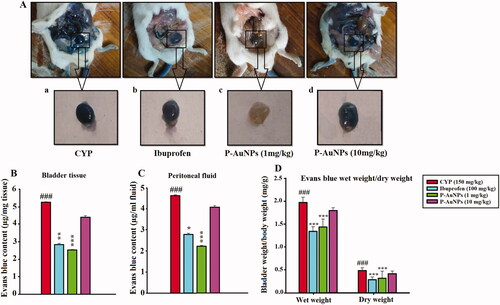
P-AuNPs attenuates decrease in urinary sodium and potassium level
The CYP-treated group presented a significant (p < .001) decrease in urinary Na+ and K+ level in comparison to the saline-treated group. However, pre-treatment with P-AuNPs remarkably attenuated the decrease in urinary electrolytes compared to the CYP-challenged group as shown in .
Table 2. Effect of P-AuNP and CYP on urinary Na+ (mmol/l) and K+ (mmol/l).
P-AuNPs reduces serum enzymes levels
CYP remarkably (p < .001) increased the level of serum enzymes such as ALT, AST, creatinine, and CRP compared to the saline-treated group shown in . However, pre-treatment with P-AuNPs (1 mg/kg) showed protection against CYP-induced toxicity by significantly (p < .001) reducing the level of serum enzymes and normalizing levels at 48 h of CYP administration indicating protective role of 1 mg/kg of P-AuNPs as compared to the CYP-treated group. However, pre-treatment with P-AuNPs (10 mg/kg) displayed marked alteration in ALT, AST, creatinine, and CRP level in the CYP-treated mice.
Table 3. Effect of P-AuNP and CYP on serum enzymes (IU/L).
P-AuNPs attenuates hematological changes
The hematological changes were evaluated in CYP-induced cystitis model, in order to evaluate the effect of P-AuNPs on the blood composition. The CYP-treated group showed remarkable (p < .001) alterations in the blood composition which included elevated level of WBCs, neutrophils, lymphocytes, monocytes, and eosinophils, while a decreased level of platelets in comparison to the saline-control group. However, P-AuNPs (1 mg/kg) treated group showed remarkable (p < .001) improvement in the blood composition as compared to the CYP-alone-treated group shown in .
Table 4. Effect of P-AuNPs pre-treatment on CYP-induced hematological changes.
P-AuNPs reduces histological changes examined by H&E and Masson’s trichrome staining
Histological markers typical for CYP-induced cystitis were absent in the saline-treated group (). In order to assess the severity of inflammation, an inflammation score was determined. P-AuNPs (1 mg/kg) significantly decreased the inflammatory infiltrate (p < .05), urethelial hyperalgesia (p < .01), haemorrhage (p < .001), and ulceration (p < .05) compared to the CYP-treated group presented in . Pre-treatment with P-AuNPs (1 mg/kg) significantly prevented the increase in collagen fibre accumulation compared to the CYP-treated group ().
Figure 8. Effect of P-AuNPs on microscopic changes induced with CYP administration (A) Representative microphotographs of (a) vehicle control, (b) CYP-treated group, (c) ibuprofen (100 mg/kg), and (d) P-AuNPs (1 mg/kg) shown by H&E-stained bladder tissue section observed at 100× magnification. (B) Representative photomicrographs of Masson’s trichrome stained bladder tissue in (a) vehicle control, (b) CYP-treated group, (c) ibuprofen (100 mg/kg), and (d) P-AuNPs (1 mg/kg) groups observed at 100× magnification. Black arrow represents haemorrhage, inflammation, and ulceration. Red arrow indicates blue-stained collagen and smooth muscles are red-counterstained.
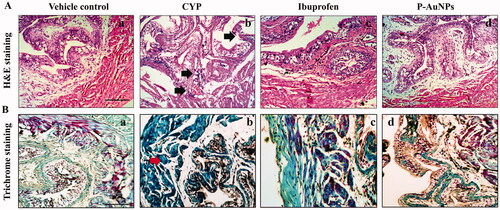
Table 5. Table represents histological evaluation of H&E stained bladder tissue section.
Discussion
CYP has limited therapeutic effectiveness due to its toxicity at several target organs such as urinary bladder, renal toxicity and less frequently, liver damage [Citation65]. Systemic administration of CYP in mice has been suggested to induce cystitis characterized by acute inflammation of the urinary bladder, visceral pain, and oxidative stress [Citation25, Citation66]. CYP-induced cystitis results from acrolein, a renally excreted active metabolite toxic to the bladder epithelium [Citation67]. Inflammatory disorders and oxidative stress develops with the cystitis overtime [Citation21]. The current study suggested the protective role of AuNPs synthesized by the reduction of aqueous gold metal ions in contact with green tea extract against the CYP-induced cystitis. The uroprotective effect of P-AuNPs were analysed by behavioural, biochemical, and histological data suggesting the contribution of hydroxyl group of phenolic compounds and tannins towards reduction reaction of Au salts to AuNPs [Citation22].
Green tea extract performed a reduction reaction of Au salts to AuNPs via a green strategy. Then, polyethylene glycol (PEG) was attached onto the surface of AuNPs i.e. PEGylation, to increase colloidal stability and biocompatibility. PEG has been known as a non-toxic polymer with hydrophilicity and has been approved by Food and Drug Administration for its use in drug delivery. In addition, for targeted and sustained drug delivery, PEGylation enhances nanoparticle diffusion by mucus [Citation68]. Green strategy, adopted in the preparation of AuNPs does not involve any toxic chemicals during the process, and in turn protects our global environment and public health [Citation22, Citation69]. Plant extract-based AuNPs include anti-microbial, anti-oxidant, as well as drug delivery and catalytic applications [Citation70,Citation71].
Systemic administration of CYP in mice has been proposed as a relevant preclinical model of acute bladder pain characterized by behaviour changes. Both the spontaneous and evoked pain behaviours were observed in the current model. The nociceptive responses consisting of allodynia and referred hyperalgesia are related to the inflammatory responses [Citation72,Citation73]. The current results suggested that both inflammation and nociception have to be considered in models of cystitis in order to define the best therapeutic treatment [Citation74,Citation75]. CYP-induced visceral hypersensitivity was characterized by a reduction in mechanical threshold in response to innocuous von Frey filaments and raised nociceptive scores due to increasing strength of filaments i.e. referred hyperalgesia. Quantifiable and reproducible alterations in nociceptive threshold (allodynia) and nociceptive intensity (referred hyperalgesia) were noted to be reversed significantly by P-AuNPs (1 mg/kg) as compared to the CYP-treated group. CYP treatment produced significant changes in spontaneous pain behaviour which included laboured breathing, piloerection, licking of the abdomen and rounded/limp body in comparison to the vehicle-control group. Pre-treatment with P-AuNPs (1 mg/kg) remarkably reduced the spontaneous pain behaviour compared to the CYP-treated group. While treatment with 10 mg/kg P-AuNPs showed no significant reduction in spontaneous pain and anti-nociceptive activity as compared to the CYP-treated group.
AuNPs are known to have anti-oxidant properties, which may be due to the ability of AuNPs to donate electrons or hydrogen ions to neutralize free radicals [Citation76]. The free radical scavenging ability of green synthesized AuNPs has been observed to be elevated compared to the extract of the same plant without nanoparticles [Citation76,Citation77]. This could be due to the absorption of plant extract anti-oxidants like polyphenols onto the surface of AuNPs, increasing the ability to scavenge free radicals and NO radicals [Citation78–80]. CYP administration increases production of ROS and decreases anti-oxidant enzymes and proteins [Citation60, Citation81]. Our results showed a similar decrease in the anti-oxidant level in the CYP-treated group, while pre-treatment with P-AuNPs (1 mg/kg) prevented CYP-induced oxidative stress that may possibly be associated with the free radical scavenging activity of P-AuNPs. The anti-oxidants like SOD, GSH, GST, and CAT are well known for their protection against damage and injuries through their anti-oxidant mechanisms. Similarly, the increased NO production responsible for the enhanced detrimental effects of CYP on bladder was reduced both in bladder tissue and plasma by pre-treatment with P-AuNPs (1 mg/kg) [Citation82,Citation83]. Since free radical-induced hepatotoxicity and renal toxicity occur with CYP, elevated levels of serum enzymes like ALT, AST, creatinine, and CRP were observed compared to the saline-control group [Citation60, Citation84]. Treatment with P-AuNPs (10 mg/kg) showed no significant alteration in ALT, AST, creatinine, and CRP compared to CYP-challenged mice. However, pre-treatment with P-AuNPs (1 mg/kg) showed protection against CYP-induced toxicity represented by reduced level of serum enzymes, maybe due to the presence of high anti-oxidants (polyphenols) on the surface of P-AuNPs at the time of synthesis.
The degree of vascular extravasation was measured by bladder wet/dry weight and Evans blue content significantly correlating with the severity of visceral pain-related behavioural reactions [Citation31]. The CYP-induced increase in Evans blue extravasation was significantly decreased by P-AuNPs (1 mg/kg) indicated by a reduced Evans blue content in bladder tissue and peritoneal cavity compared to the negative control group. Additionally, weight analysis indicated decrease in tissue edoema in P-AuNPs-treated group compared to CYP-challenged bladder. Furthermore, P-AuNPs (1 mg/kg) also decreased the bladder’s microscopic and macroscopic changes caused by CYP-treatment. In the present study, CYP-induced cystitis has been well characterized morphologically and biochemically. It develops mild inflammatory response in bladder tissue by submucosal edoema, inflammatory infiltrate, tissue haemorrhages, urothelium loss, and mucosal ulcerations consistent with the previous studies [Citation85,Citation86]. Macroscopic analysis made according to Gray et al. [55] defined a score evaluation of bladder wall edoema, haemorrhage, and weight. P-AuNPs (1 mg/kg) significantly decreased the scores for macroscopic evaluation compared to the CYP-treated group. Bladder tissue stained with Masson’s trichrome indicated increase in fibrosis and collagen deposition in tissue in the CYP-treated group, postulated that the inflammation could account for this increase in collagen fibres deposition [Citation87]. Pre-treatment with P-AuNPs significantly prevented the increase in collagen fibre accumulation compared to the CYP-treated group.
CYP-induced urinary hypokalaemia and hyponatremia resulted from the electrolyte alterations due to increased permeability of bladder epithelial membrane [Citation59]. It could be due to the direct effect of acrolein on bladder epithelium resulting in free flux of urinary cations like Na+ and K+ across the membrane, resulting in the alteration in concentration on both sides of epithelium [Citation59]. Thus, the normally high concentration of urinary Na+ and K+ was lower in CYP-treated animals compared to control mice [Citation59]. Pre-treatment with P-AuNPs remarkably attenuated the decrease in urinary electrolytes compared to the CYP-challenged group. Similarly, increase in transepithelial permeability resulted in enhanced penetration of toxins, leading to the infiltration of inflammatory cells such as monocytes, lymphocytes, neutrophils and platelets, producing more ROS [Citation63, Citation88]. Investigation of blood revealed increased number of these inflammatory cells in the CYP-treated group, which were significantly prevented by pre-treatment with P-AuNPs. Additionally, the green synthesis of P-AuNPs (1 mg/kg) presented no distress symptoms indicating no change in movement and temperament of the mice compared to the CYP-treated group.
Conclusion
The present study demonstrated the uroprotective effect of P-AuNPs prepared by green synthesis on reduction with green tea extract against CYP-induced cystitis in mice (). P-AuNPs prevented bladder edoema and reversed bladder pain-like behaviours such as spontaneous and mechanical nociception. Alterations in urinary electrolytes, hematological parameters, and serum enzymes were remarkably prevented by P-AuNPs. Additionally, results obtained from macroscopic and histological analysis further confirmed the protective effect of P-AuNPs on bladder tissue.
Consent for publication
All authors have provided consent for the manuscript to be published.
Author contributions
Bushra Shal: Conceptualization, Methodology, Investigation, Visualization, Data curation, Writing – original draft. Safa Amanat: Investigation and Visualization. Ashraf Ullah Khan: Investigation, Data curation, and Writing – review editing. You Jeong Lee: Synthesis and Characterization of P-AuNPs. Fakhar Ud-Din: Resources and Validation. Hussain Ali: Validation, Data curation, and Writing – review editing. Youmie Park: Conceptualization, Resources, Synthesis, Characterization of P-AuNPs and Writing – original draft. Salman Khan: Conceptualization, Visualization, Validation, Data curation, Writing – review editing, Project administration, and Supervision.
Acknowledgments
The authors thank Ms Gayeon Lee at Inje University for her experimental assistance.
Disclosure statement
The authors declare that they have no known competing financial interests or personal relationships that could have appeared to influence the work reported in this paper.
Data availability statement
All data generated or analysed during this study are included in this published article.
Additional information
Funding
References
- Ip EJ, Lee-Ma A, Troxell LS, et al. Low-dose filgrastim in patients with breast cancer treated with docetaxel, doxorubicin, and cyclophosphamide. Am J Health Syst Pharm. 2008;65(16):130–1555.
- Inamoto Y, Suzuki R, Kuwatsuka Y, et al. Long-term outcome after bone marrow transplantation for aplastic anemia using cyclophosphamide and total lymphoid irradiation as conditioning regimen. Biol Blood Marrow Transplant. 2008;14(1):43–49.
- Batista C, Brito GAC, Souza MLP, et al. A model of hemorrhagic cystitis induced with acrolein in mice. Braz J Med Biol Res. 2006;39(11):1475–1481.
- Elahi N, Kamali M, Baghersad MH. Recent biomedical applications of gold nanoparticles: a review. Talanta. 2018;184:537–556.
- Sood R, Chopra DS. Metal-plant frameworks in nanotechnology: an overview. Phytomedicine. 2018;50:148–156.
- Yadi M, Mostafavi E, Saleh B, et al. Current developments in green synthesis of metallic nanoparticles using plant extracts: a review. Artif Cells Nanomed Biotechnol. 2018;46(suppl 3):S336–S343.
- Cox PJ. Cyclophosphamide cystitis–identification of acrolein as the causative agent. Biochem Pharmacol. 1979;28(13):2045–2049.
- Stankiewicz A, Skrzydlewska E, Makiela M. Effects of amifostine on liver oxidative stress caused by cyclophosphamide administration to rats. Drug Metabol Drug Interact. 2002;19(2):67–82.
- Shehzad A, Khan S, Sup Lee Y. Curcumin molecular targets in obesity and obesity-related cancers. Future Oncol. 2012;8(2):179–190.
- Halliwell B, Cross CE. Oxygen-derived species: their relation to human disease and environmental stress. Environ Health Perspect. 1994;102(Suppl 10):5–12.
- Ajith TA, Nivitha V, Usha S. Zingiber officinale roscoe alone and in combination with alpha-tocopherol protect the kidney against cisplatin-induced acute renal failure. Food Chem Toxicol. 2007;45(6):921–927.
- Shehzad A, Khan S, Shehzad O, et al. Curcumin therapeutic promises and bioavailability in colorectal cancer. Drugs Today. 2010;46(7):523–532.
- Reuter S, Gupta SC, Chaturvedi MM, et al. Oxidative stress, inflammation, and cancer: how are they linked? Free Radic Biol Med. 2010;49(11):1603–1616.
- Choi RJ, Chun J, Khan S, et al. Desoxyrhapontigenin, a potent anti-inflammatory phytochemical, inhibits LPS-induced inflammatory responses via suppressing Nf-κb and MAPK pathways in raw 264.7 cells. Int Immunopharmacol. 2014;18(1):182–190.
- Gilad E, Wong HR, Zingarelli B, et al. Melatonin inhibits expression of the inducible isoform of nitric oxide synthase in murine macrophages: role of inhibition of Nf-kappab activation. FASEB J. 1998;12(9):685–693.
- Khan SM, Choi RJ, Lee DU, et al. Sesquiterpene derivatives isolated from Cyperus rotundus L. inhibit inflammatory signaling mediated by Nf-κb. Nat Prod Sci. 2011;17(3):250–255.
- Bon K, Lichtensteiger CA, Wilson SG, et al. Characterization of cyclophosphamide cystitis, a model of visceral and referred pain, in the mouse: species and strain differences. J Urol. 2003;170(3):1008–1012.
- Boucher M, Meen M, Codron J-P, et al. Cyclophosphamide-induced cystitis in freely-moving conscious rats: behavioral approach to a new model of visceral pain. J Urol. 2000;164(1):203–208.
- Chun J, Choi RJ, Khan S, et al. Alantolactone suppresses inducible nitric oxide synthase and cyclooxygenase-2 expression by down-regulating Nf-κb, MAPK and Ap-1 via the Myd88 signaling pathway in LPS-activated raw 264.7 cells. Int Immunopharmacol. 2012;14(4):375–383.
- Chacko SM, Thambi PT, Kuttan R, et al. Beneficial effects of green tea: a literature review. Chin Med. 2010;5:13–13.
- Karim AJ, Dalai DR. Green tea: a review on its natural anti-oxidant therapy and cariostatic benefits. J Issues ISSN. 2014;2350:1588.
- Park Y, Hong YN, Weyers A, et al. Polysaccharides and phytochemicals: a natural reservoir for the green synthesis of gold and silver nanoparticles. IET Nanobiotechnol. 2011;5(3):69–78.
- Jigisha A, Nishant R, Navin K, et al. Green tea: a magical herb with miraculous outcomes. Int. Res. J. Pharm. 2012;3(5):139–148.
- Lee YJ, Ahn E-Y, Park Y. Shape-dependent cytotoxicity and cellular uptake of gold nanoparticles synthesized using green tea extract. Nanoscale Res Lett. 2019;14(1):129.
- Auge C, Chene G, Dubourdeau M, et al. Relevance of the cyclophosphamide-induced cystitis model for pharmacological studies targeting inflammation and pain of the bladder. Eur J Pharmacol. 2013;707(1–3):32–40.
- De Logu F, Li Puma S, Landini L, et al. The acyl-glucuronide metabolite of ibuprofen has analgesic and anti-inflammatory effects via the Trpa1 channel. Pharmacol Res. 2019;142:127–139.
- Nausch B, Pace S, Pein H, et al. The standardized herbal combination BNO 2103 contained in Canephron® N alleviates inflammatory pain in experimental cystitis and prostatitis. Phytomedicine. 2019;60:152987.
- Driver-Dunckley ED, Zhang N, Adler CH, et al. Brain Lewy-type synucleinopathy density is associated with a lower prevalence of atherosclerotic cardiovascular disease risk factors in patients with Parkinson’s disease. JPD. 2019;9(3):510–543.
- Miki T, Matsunami M, Nakamura S, et al. Ono-8130, a selective prostanoid Ep1 receptor antagonist, relieves bladder pain in mice with cyclophosphamide-induced cystitis. Pain. 2011;152(6):1373–1381.
- Laird JM, Souslova V, Wood JN, et al. Deficits in visceral pain and referred hyperalgesia in Nav1.8 (Sns/Pn3)-null mice. J Neurosci. 2002;22(19):8352–8356.
- Olivar T, Laird JM. Cyclophosphamide cystitis in mice: behavioural characterisation and correlation with bladder inflammation. Eur J Pain. 1999;3(2):141–149.
- Afridi R, Khan AU, Khalid S, et al. Anti-hyperalgesic properties of a flavanone derivative poncirin in acute and chronic inflammatory pain models in mice. BMC Pharmacol Toxicol. 2019;20(1):57.
- Khalid S, Khan A, Shal B, et al. Suppression of Trpv1 and P2y nociceptors by honokiol isolated from magnolia officinalis in 3rd degree burn mice by inhibiting inflammatory mediators. Biomed Pharmacother. 2019;114:108777.
- Khan D, Qindeel M, Ahmed N, et al. Development of novel pH-sensitive nanoparticle-based transdermal patch for management of rheumatoid arthritis. Nanomedicine. 2020;15(6):603–624.
- Atiq A, Shal B, Naveed M, et al. Diadzein ameliorates 5-fluorouracil-induced intestinal mucositis by suppressing oxidative stress and inflammatory mediators in rodents. Eur J Pharmacol. 2019;843:292–306.
- Amanat S, Shal B, Kyoung Seo E, et al. Icariin attenuates cyclophosphamide-induced cystitis via down-regulation of Nf-κb and up-regulation of Nrf-2/Ho-1 signaling pathways in mice model. Int Immunopharmacol. 2022;106:108604.
- Ullah MZ, Khan AU, Afridi R, et al. Attenuation of inflammatory pain by puerarin in animal model of inflammation through inhibition of pro-inflammatory mediators. Int Immunopharmacol. 2018;61:306–316.
- Moron MS, Depierre JW, Mannervik B. Levels of glutathione, glutathione reductase and glutathione S-transferase activities in rat lung and liver. Biochim Biophys Acta. 1979;582(1):67–78.
- Ali J, Khan AU, Shah FA, et al. Mucoprotective effects of saikosaponin-a in 5-fluorouracil-induced intestinal mucositis in mice model. Life Sci. 2019;239:116888.
- Khan A, Shal B, Naveed M, et al. Matrine ameliorates anxiety and depression-like behaviour by targeting hyperammonemia-induced neuroinflammation and oxidative stress in Ccl4 model of liver injury. Neurotoxicology. 2019;72:38–50.
- Ullah H, Khan A, Baig MW, et al. Poncirin attenuates Ccl4-induced liver injury through inhibition of oxidative stress and inflammatory cytokines in mice. BMC Complement Med Ther. 2020;20(1):115.
- Warholm M, Guthenberg C, von Bahr C, et al. Glutathione transferases from human liver. Methods Enzymol. 1985;113:499–504.
- Khan A, Khan A, Shal B, et al. N-(benzylidene)-2-((2-hydroxynaphthalen-1-Yl)diazenyl)benzohydrazides (1-2) (NCHDH and NTHDH) attenuate DMBA-induced breast cancer via Nrf2/Nf-κb/apoptosis signaling. Fundam Clin Pharmacol. 2022;1–19.
- Shal B, Khan A, Naveed M, et al. Neuroprotective effect of 25-Methoxyhispidol A against CCl4-induced behavioral alterations by targeting VEGF/BDNF and caspase-3 in mice. Life Sci. 2020;253:117684.
- Qindeel M, Khan D, Ahmed N, et al. Surfactant-free, self-assembled nanomicelles-based transdermal hydrogel for safe and targeted delivery of methotrexate against rheumatoid arthritis. ACS Nano. 2020;14(4):4662–4681.
- Marklund S, Marklund G. Involvement of the superoxide anion radical in the autoxidation of pyrogallol and a convenient assay for superoxide dismutase. Eur J Biochem. 1974;47(3):469–474.
- Hamsa TP, Kuttan G. Protective role of ipomoea obscura (L.) on cyclophosphamide-induced uro- and nephrotoxicities by modulating antioxidant status and pro-inflammatory cytokine levels. Inflammopharmacology. 2011;19(3):155–167.
- Shal B, Khan A, Naveed M, et al. Effect of 25-Methoxyhispidol A isolated from poncirus trifoliate against bacteria-induced anxiety and depression by targeting neuroinflammation, oxidative stress and apoptosis in mice. Biomed Pharmacother. 2019;111:209–223.
- Khan A, Shal B, Naveed M, et al. Matrine alleviates neurobehavioral alterations via modulation of JNK-mediated caspase-3 and BDNF/VEGF signaling in a mouse model of burn injury. Psychopharmacology. 2020;237(8):2327–2343.
- Miranda KM, Espey MG, Wink DA. A rapid, simple spectrophotometric method for simultaneous detection of nitrate and nitrite. Nitric Oxide. 2001;5(1):62–71.
- Shal B, Khan A, Khan AU, et al. Alleviation of memory deficit by bergenin via the regulation of reelin and Nrf-2/NF-κb pathway in transgenic mouse model. Int J Mol Sci. 2021;22(12):6603.
- Khan A, Khan S, Ali H, et al. Anomalin attenuates LPS-induced acute lungs injury through inhibition of Ap-1 signaling. Int Immunopharmacol. 2019;73:451–460.
- Naveed M, Khan SZ, Zeeshan S, et al. A new cationic palladium(Ii) dithiocarbamate exhibits anti-inflammatory, analgesic, and antipyretic activities through inhibition of inflammatory mediators in in vivo models. Naunyn Schmiedebergs Arch Pharmacol. 2019;392(8):961–977.
- Khan S, Shehzad O, Jin H-G, et al. Anti-inflammatory mechanism of 15,16-epoxy-3α-hydroxylabda-8,13(16),14-trien-7-one via inhibition of LPS-induced multicellular signaling pathways. J Nat Prod. 2012;75(1):67–71.
- Gray KJ, Engelmann UH, Johnson EH, et al. Evaluation of misoprostol cytoprotection of the bladder with cyclophosphamide (ucurbi) therapy. J Urol. 1986;136(2):497–500.
- Khan A, Shal B, Khan AU, et al. Withametelin, a novel phytosterol, alleviates neurological symptoms in EAE mouse model of multiple sclerosis via modulation of Nrf2/Ho-1 and Tlr4/NF-κb signaling. Neurochem Int. 2021;151:105211.
- Wu KC, Chiang BJ, Tsai WH, et al. I-Tiao-Gung extract through its active component daidzin improves cyclophosphamide-induced bladder dysfunction in rat model. Neurourol Urodyn. 2018;37(8):2560–2570.
- Khan AM, Khan AU, Ali H, et al. Continentalic acid exhibited nephroprotective activity against the LPS and E. coli-induced kidney injury through inhibition of the oxidative stress and inflammation. Int Immunopharmacol. 2020;80:106209.
- Parsons CL, Greene RA, Chung M, et al. Abnormal urinary potassium metabolism in patients with interstitial cystitis. J Urol. 2005;173(4):1182–1185.
- Nafees S, Rashid S, Ali N, et al. Rutin ameliorates cyclophosphamide induced oxidative stress and inflammation in Wistar rats: role of Nfκb/MAPK pathway. Chem Biol Interact. 2015;231:98–107.
- Sher YP, Tzeng TF, Kan SF, et al. Cancer targeted gene therapy of BIKDD inhibits orthotopic lung cancer growth and improves long-term survival. Oncogene. 2009;28(37):3286–3295.
- Lin G, Luo D, Liu J, et al. Hepatoprotective effect of polysaccharides isolated from dendrobium officinale against acetaminophen-induced liver injury in mice via regulation of the Nrf2-Keap1 signaling pathway. Oxid Med Cell Longev. 2018;2018:6962439.
- Khan S, Shehzad O, Chun J, et al. Anti-hyperalgesic and anti-allodynic activities of capillarisin via suppression of inflammatory signaling in animal model. J Ethnopharmacol. 2014;152(3):478–486.
- Singh S, Sachan P, Madeshiya A, et al. Quantitative analysis of serum uric acid and C-reactive protein level in North Indian pre-diabetic and diabetic subjects. Int J Biomed Res. 2013;4(11):596–602.
- Hutter AM, Bauman AW, Frank IN. Cyclophosphamide and severe hemorrhagic cystitis. NY State J Med. 1969;69(2):305–309.
- Khan S, Choi RJ, Shehzad O, et al. Molecular mechanism of capillarisin-mediated inhibition of Myd88/Tirap inflammatory signaling in in vitro and in vivo experimental models. J Ethnopharmacol. 2013;145(2):626–637.
- Brock N, Pohl J, Stekar J. Studies on the urotoxicity of oxazaphosphorine cytostatics and its prevention–I. Experimental studies on the urotoxicity of alkylating compounds. Eur J Cancer. 1981;17(6):595–607.
- Huckaby JT, Lai SK. Pegylation for enhancing nanoparticle diffusion in mucus. Adv Drug Deliv Rev. 2018;124:125–139.
- Park Y. A new paradigm shift for the green synthesis of antibacterial silver nanoparticles utilizing plant extracts. Toxicol Res. 2014;30(3):169–178.
- Khan T, Ullah N, Khan MA, et al. Plant-based gold nanoparticles; a comprehensive review of the decade-long research on synthesis, mechanistic aspects and diverse applications. Adv Colloid Interface Sci. 2019;272:102017.
- Zeeshan M, Ali H, Khan S, et al. Glycyrrhizic acid-loaded pH-sensitive poly-(lactic-co-glycolic acid) nanoparticles for the amelioration of inflammatory bowel disease. Nanomedicine. 2019;14(15):1945–1969.
- Khan S, Choi RJ, Lee J, et al. Attenuation of neuropathic pain and neuroinflammatory responses by a pyranocoumarin derivative, anomalin in animal and cellular models. Eur J Pharmacol. 2016;774:95–104.
- Khan A, Ullah MZ, Afridi R, et al. Antinociceptive properties of 25‐Methoxyhispidol A, a ucurbitacea isolated from poncirus trifoliata (rutaceae) through inhibition of Nf‐κb signalling in mice. Phytother Res. 2019;33(2):327–341.
- Khan A, Khan S, Kim YS. Insight into pain modulation: nociceptors sensitization and therapeutic targets. Curr Drug Targets. 2019;20(7):775–788.
- Khan S, Shehzad O, Chun J, et al. Mechanism underlying anti-hyperalgesic and anti-allodynic properties of anomalin in both acute and chronic inflammatory pain models in mice through inhibition of Nf-κb, MAPKS and CREB signaling cascades. Eur J Pharmacol. 2013;718(1–3):448–458.
- Patra JK, Baek K-H. Novel green synthesis of gold nanoparticles using ucurbita lanatus rind and investigation of proteasome inhibitory activity, antibacterial, and antioxidant potential. Int J Nanomedicine. 2015;10:7253–7264.
- Chitra G, Balasubramani G, Ramkumar R, et al. Mukia maderaspatana (ucurbitaceae) extract-mediated synthesis of silver nanoparticles to control Culex quinquefasciatus and Aedes aegypti (Diptera: Culicidae). Parasitol Res. 2015;114(4):1407–1415.
- Ganaie SU, Abbasi T, Abbasi SA. Utilization of the terrestrial weed a Ntigonon leptopus in the rapid and green synthesis of stable gold nanoparticles with shape/size control. Environ Prog Sustainable Energy. 2016;35(1):20–33.
- Ain Q, Zeeshan M, Khan S, et al. Biomimetic hydroxyapatite as potential polymeric nanocarrier for the treatment of rheumatoid arthritis. J Biomed Mater Res A. 2019;107(12):2595–2600.
- Khan S, Shehzad O, Cheng M-S, et al. Pharmacological mechanism underlying anti-inflammatory properties of two structurally divergent coumarins through the inhibition of pro-inflammatory enzymes and cytokines. J Inflamm. 2015;12(1):47.
- Soumaya K, Rouissi K, Hamrita B, et al. Therapeutic effects of aloe vera plant extract against cyclophosphamide and buthionine sulfoximine induced toxicities in the bladder. Biochem Pharmacol. 2012;1(101):2167–0501.1000101.
- Khan S, Shehzad O, Lee KJ, et al. Anti-inflammatory properties of samidin from Seseli resinosum through suppression of Nf-κb and Ap-1-mediated-genes in LPS-stimulated raw 264.7 cells. Arch Pharm Res. 2014;37(11):1496–1503.
- Khan S, Shin EM, Choi RJ, et al. Suppression of LPS-induced inflammatory and NF-κB responses by anomalin in RAW 264.7 macrophages. J Cell Biochem. 2011;112(8):2179–2188.
- Khalid S, Ullah MZ, Khan AU, et al. Antihyperalgesic properties of honokiol in inflammatory pain models by targeting of Nf-κb and Nrf2 signaling. Front Pharmacol. 2018;9:140.
- Chow YC, Yang S, Huang CJ, et al. Prophylactic intravesical instillation of epinephrine prevents cyclophosphamide-induced hemorrhagic cystitis in rats. Exp Biol Med. 2007;232(4):565–570.
- Matsuoka Y, Masuda H, Yokoyama M, et al. Protective effects of bilirubin against cyclophosphamide induced hemorrhagic cystitis in rats. J Urol. 2008;179(3):1160–1166.
- Taha NR, Amin HA, Sultan AA. The protective effect of moringa oleifera leaves against cyclophosphamide-induced urinary bladder toxicity in rats. Tissue Cell. 2015;47(1):94–104.
- Hotter G, Closa D, Prats N, et al. Free radical enhancement promotes leucocyte recruitment through a PAF and LTB4 dependent mechanism. Free Radic Biol Med. 1997;22(6):947–954.