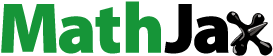
Abstract
This study aimed to synthesize the silver nanoparticles (SNPs) and loaded chitosan nanoparticles (LCNPs) using Euphorbia prostata based on their anticandidal activity. Antioxidant capacity and the total phenolic and total flavonoid content of plant samples and synthesized nanoparticles (NPs) were also evaluated. SNPs and LCNPs were prepared, respectively using chemical reduction of silver salt solution and ionotropic gelation method. The anticandidal activity was assessed by broth micro-dilution method and the antioxidant activity was determined using free-radical scavenging assays. The synthesized NPs after the optimization process were found to be spherical with sizes ranging from 12 to 100 nm. Spectroscopic analysis of NPs showed the appearance of peaks in prescribed wavelength ranging between 402 and 493 nm. The synthesized NPs showed potent anticandidal activity compared to the free extract. The SNPs formulations NpEPM 7.5 and NpEPMR 7.5, showed significantly low MIC values ranging between 2 and 128 µg/mL. In the case of LCNPs, NpEPM (4:1) and NpEPME (4:1) also showed lower MIC values ranging from 32 to 256 µg/mL. The plant samples as well as NPs showed antioxidant potential. In addition, plant extracts and NPs possess the potent biological potential and can be further investigated through in vivo experiments.
Introduction
The advent of nanotechnology, the biggest engineering innovation of recent times, has modernized the current medicines. The demand for nanotechnology-derived products is constantly increasing [Citation1]. Biosynthesis of green nanoparticles using plant extracts is an interesting area in the field of nanotechnology, which has economic and eco-friendly benefits over chemical and physical methods of synthesis. Chemical and physical methods of synthesis are potentially hazardous to the environment and involve the use of toxic and perilous chemicals [Citation2,Citation3]. Studies have shown that the size, morphology, stability, and properties (chemical and physical) of the NPs are strongly influenced by the experimental conditions, the kinetics of interaction of reagents, and the adsorption processes of a stabilizing agent with NPs [Citation4,Citation5]. Broadly speaking, there are two approaches to NPs production: top-down and bottom-up. Production of NPs requires an understanding of the fundamentals of nanoscale chemistry and physics [Citation5].
Human fungal infections pose serious medical issues. During the last decade, the incidence of superficial and systemic fungal infections has continued to increase explosively, due to the increasing number of immunocompromised persons, affecting millions of people worldwide [Citation6]. The use of synthetic chemicals for controlling these fungal infections is not the most secure method because of the reported toxic effects of these chemicals and the development of resistance against them [Citation7,Citation8]. Therefore, it is necessary to search for novel treatment options with improved features, and green synthesized NPs are an alternative. NPs are known to have extremely large surface areas which provide good contact and interaction with microbes, thus contributing to their good antimicrobial activity [Citation1]. Nanoscale materials have emerged as novel antifungal agents, owing to their high surface area to volume ratio and their unique chemical and physical properties [Citation9].
Silver and plant sample-loaded nanoparticles have been reported to be efficient for fighting against resistant microbes [Citation9–11]. Jabir et al. synthesized silver nanoparticles from Eriobotrya japonica extract and found them to have promising anticancer activity [Citation9]. Hawar et al. reported the antifungal role of silver nanoparticles prepared from Alhagi graecorum leaf extract [Citation10]. Another study revealed the enhanced autophagy-based anticancer effect of silver nanoparticles developed from Annona muricata Extract [Citation11]. Chitosan nanoparticle is a delivery system that offer high intracellular penetration and the potential for effective intracellular antimicrobial activity [Citation12], while silver nanoparticle can penetrate inside the cell membranes and cause damage that can lead to the death of the cell [Citation13]. This explains in part the growing interest in the synthesis of these two types of nanoparticles.
The objective of the present study was to synthesize the SNPs and LCNPs using crude extract and fractions of Euphorbia prostata based on their anticandidal activity. In addition, antioxidant activity, as well as total phenolic and total flavonoid content quantification of plant samples and synthesized NPs, was also performed.
Materials and methods
Chemicals
Chitosan (viscosity=20–300 cP, molecular weight = 50–190 kDa, 86.6% deacetylated, from crab shells) was obtained from Sigma Aldrich. Tripolyphosphate (TPP) and Silver nitrate were also supplied by Sigma Aldrich. All other materials and reagents used in this study were of the analytical grade of purity.
Collection and identification of E. prostata
The Euphorbia prostate whole plant was collected in December 2015 in the Baham Subdivision and it was identified by Dr. Tchiengue Barthelemy, the botanist at the Cameroon National Herbarium (CNH).
Extraction and partitioning
Dried and ground plant material (100 g) was soaked in 600 ml of methanol for 48 h with intermittent shaking. The organic solvent was separated from plant material by the use of Whatman No. 1 filter paper and the filtrate evaporated under a vacuum at 40 °C. A part of the obtained crude extract was then partitioned with n-hexane, chloroform, and ethyl acetate according to the previously described method [Citation14].
Synthesis of SNPs
SNPs were synthesized using the chemical reduction of silver salt solution method (one-step method). To obtain SNPs with better anticandidal activity, the synthesis protocol has been optimized in two steps [Citation14].
First optimization step of SNPs synthesis
Different concentrations of 40 ml aqueous solution of silver nitrate, that is, 1, 5, and 10 mM were mixed in a bottle with 6 ml of methanolic solution of plant extract and fractions (5 mg/mL). The mixture was then stirred and incubated at room temperature in the dark for 24 h to allow the synthesis of the SNPs. The presence of the SNPs was detected visually by the rapid change in colour of the mixture. After the incubation period, the contents of each container were centrifuged for 15 min at 10,000 rpm to get NPs as pellets. Freeze-dried SNPs were stored at 4 °C for further biological and chemical analysis.
Second optimization step of SNPs synthesis
The concentrations of silver nitrate which showed the highest anticandidal activity obtained from the previous optimization step were kept constant and the concentration of extract varied from 7.5 and 10 mg/mL to determine the optimum value.
After optimization of silver nitrate and extract concentration, the potent formulation in terms of anticandidal activity was selected for further analysis
Synthesis of chitosan NPs
Chitosan NPs were prepared according to the ionotropic gelation process as described by Özdamar [Citation15]. As a consequence of mixing 0.5%, chitosan solution with 0.1% tripolyphosphate (TPP) solution (chitosan/TPP ratio = 5:1) with stirring at room temperature, the formation of chitosan NPs started spontaneously through the ionotropic gelation mechanism initiated by the TPP. The chitosan and TPP mixture were stirred for 1 h and then centrifuged at 12,000 rpm for 45 min. The NPs obtained were lyophilized and stored at 4 °C.
To obtain LCNPs with the highest anticandidal activity, the synthesis procedure was also optimized in two steps.
First optimization step of LCNPs synthesis
During the first step of optimization, 0.5% chitosan solution was first mixed with the methanolic solution of plant extract (5 mg/mL) in different ratios, that is (4:1), (3:2), and (1:1), and incubated with stirring for 30 min. Then after the incubation period, 0.1% aqueous solution of TPP was added to the chitosan-extract (chitosan-extract/TPP=5:1), stirring at room temperature. At the end of this synthesis step, the anticandidal activity of the freeze-dried LCNPs was determined.
Second optimization step of LCNPs synthesis
The chitosan/plant extract ratio which showed the best anticandidal activity was selected and different plant extract concentrations of 7.5 and 10 mg/mL were applied.
Once this synthesis optimization was completed, the LCNPs obtained were tested for anticandidal activity, and the most potent combinations were selected for further analysis.
Determination of the loading capacity of both flavonoids and phenolic compounds by chitosan NPs
The procedure followed to determine the total flavonoid and phenolic contents of extracts were used to determine loading capacity with slight modification. Loading capacity (LC) of the flavonoids and phenolic compounds by chitosan NPs was calculated as follows:
C = TFC or TPC of the weight of the loaded chitosan NPs obtained
E = TFC or TPC of the weight of plant extract or fractions used for the synthesis of NPs
Characterization of SNPs and LCNPs
The presence and the formation of SNPs were first detected by visual observation after 24 h of incubation and then confirmed by a UV-Visible spectral analysis (UV-Visible spectrophotometer UV3000) in the wavelength range 200–700 nm [Citation15]. After the formation of SNPs and LCNPs, dried ones were subjected to Fourier transform infrared spectroscopy (FTIR) analysis. The spectra were measured in the wavelength range 600–4000 cm−1 at 1 cm−1 resolution [Citation16]. Hitachi S-4500 Scanning electron microscopy was used for SEM analysis [Citation17].
Anticandidal assay
The anticandidal activity of plant samples, SNPs and LCNPs was performed against two strains of yeast including Candida albicans ATCC9002, Candida parapsilosis ATCC22019, and two clinical isolates (Candida krusei and C. albicans). Minimum inhibitory concentrations (MICs) and minimum fungicidal concentrations (MFCs) were determined by the broth microdilution method as previously described by Tchuenguem et al. [Citation18]. Ketoconazole was used as positive control and all the experiments were carried out in triplicate.
Antioxidant activity
Antioxidant activity of plant samples, SNPs and LCNPs were determined using DPPH and reducing power assays. Total phenolic content (TPC) and total flavonoid content (TFC) were determined by using the previously described method [Citation18].
Result and discussion
Extraction and partitioning
Plant extraction yielded 20 g of crude extract of E. prostata which after partitioning produced 6.28, 1.2, 3.91, and 7.03 g of hexane, chloroform, ethyl acetate, and residual fraction, respectively.
Anticandidal activity of plant extract and fractions of E. prostata
The anticandidal activities of plant samples of E. prostata were investigated using the broth microdilution method. Their MICs and MFCs were also determined (). These samples showed variable anticandidal activity with MIC values ranging from 16 to 1024 µg/mL. The ethyl acetate fraction of E. prostate presented the highest antifungal activity with MIC values ranging from16 to 128 µg/mL. These results are correlated with previous reports about the anticandidal potential of Euphorbia genus [Citation19].
Table 1. MICs and MFCs of the plant extract and fractions were recorded (µg/mL).
Characterization of SNPs and LCNPs
UV-Vis spectroscopic analysis
The SNPs synthesis was confirmed by observing the change in colour of the reaction mixture. The yellow coloured reaction mixture was turned into a dark brown colour due to excitation of surface plasmon resonance indicating the biotransformation of ionic silver to reduced silver. The UV-Vis spectra of SNPs that showed the highest antifungal potential are presented in . It was observed that the maximum absorbance of the SNPs obtained after the optimization process occurred between 402 and 493 nm. According to Sileikaite et al., the absorption band in the visible light region (350–550 nm) is typical for SNPs [Citation20].
Infra-red spectroscopic analysis
The FTIR spectra of NPs which showed the highest antifungal potential in both SNPs and LCNPs are presented in . Their analysis shows stretching vibration of OH (alcohol and phenolic), C=O, C–O– (acid), CH, C≡C, C=C (aromatic system), C–O– (alcohol), C–N bonds as well as the functional group NO2 and C–Cl which might belong to phenolic and flavonoid compounds present at the surface of the most active NPs. The flavonoids and phenolic compounds present in the crude extracts of E. prostate and its fractions are powerful reducing agents which play a significant role in the formation of SNPs by reduction of silver ions [Citation20]. It has already been reported that flavonoids and phenolics compounds present in plant extracts can play the dual function of reducing and capping agents during SNPs formation [Citation21]. In addition, stretching vibration of bonds found in LCNPs might be one of the phenolic compounds found in these samples during the total phenolic content determination. Results are comparable with previous reports [Citation22].
Scanning electron microscopic analysis
The surface morphology of the dried chitosan nanoparticles loaded with methanolic extract (EPM) and ethyl acetate fraction (EPME) of E. prostata was studied using a scanning electron microscope (SEM). The suspension of chitosan nanoparticles was prepared in deionized water and sonicated for homogeneous dispersion. One drop of nanoparticles suspension was placed on a glass plate and dried at room temperature. Gold coating of these dried nanoparticles was performed under a high vacuum. Images were taken at an excitation of the voltage of 10 kV and at 100,000× magnification. These nanoparticles were also found active in biological studies. SEM analysis indicated the presence of morphologically smooth and spherical particles with sizes ranging between 12 and 100 nm (). The chitosan-loaded extract is considered a useful method that avoided the use of organic solvents as well as prevents the damage of active compounds that exist in the extract. However biological potential depends upon loading efficiency and the nature of extract [Citation23].
The size and shape of silver nanoparticles of selected AgNO3 (10 mM) and plant extract (7.5 mg/ml) combination were determined by SEM analysis [Citation24]. The samples were prepared by drop-casting an aliquot of AgNPs solution onto a carbon-coated copper grid, which was placed on a piece of paper to get rid of excess solvent. Photographs were taken at an excitation of the voltage of 10 kV and at 60,000× magnification. These nanoparticles were also found active in biological studies. SEM analysis indicated the presence of morphologically less smooth and with size ranging between 86 and 100 nm. These samples also showed some aggregation during analysis. In the case of SNPs, experimental conditions might have affected the nucleation and growth of the crystal nuclei, causing the smaller size of NPs. These results are comparable with the results of many previous researchers [Citation22,Citation25]. Kamalakannan et al. studied AgNPs synthesized from the plant, Argemone mexicana with the help of SEM [Citation23,Citation26]. Another study by Zia et al. determined the size and morphology of nanoparticles through SEM [Citation24,Citation27].
Anticandidal activity of nanoparticles
In the case of the first step, SNPs were synthesized by using three concentrations of silver nitrate (1, 5, and 10 mM) and the optimum concentration of silver nitrate for every fraction was selected (). All the SNPs show a good result when synthesized from 10 mM silver nitrate. After selecting the optimum concentration of silver nitrate at 10 mM, in the next step, it was kept constant, and two concentrations (7.5 and 10 mg/mL) of extract and fractions were used for each case. Synthesized SNPs were tested against fungal strains to get the optimum combination of silver and plant concentration (). It was observed that SNPs synthesized from plant samples with a concentration of 7.5 mg/mL and 10 mM silver nitrate were the optimum combination in terms of anticandidal potential.
Table 2. MICs and MFCs of SNPs were synthesized during the first (a) and the second (b) step of the optimization procedure (µg/mL).
In the case of synthesis of LCNPs initially, three different ratios (4:1, 3:2, and 1:1) of chitosan and plant samples were used. The results of anticandidal activity showed that the 4:1 combination was best (). The optimum chitosan/plant extract ratio (4:1) was maintained constant and two concentrations of 7.5 and 10 mg/mL of extracts were used for each case to see the effect of extract concentration. The results of antifungal activity for this step show that there is no significant effect on the anticandidal potential of NPs ().
Table 3. MICs and MFCs of LCNPs were synthesized during the first (a) and the second (b) step of the optimization procedure (µg/mL).
It is observed from the optimization procedure results that for SNPs, the formulation NpEPM 7.5 and NpEPMR 7.5 obtained from the combination of 10 mM silver nitrate with respectively EPM and EPMR at 7.5 mg/mL, presented the highest anticandidal potential with MICs ranging from 2 to 128 µg/mL.
The formulation NpEPM 4:1 and NpEPME 4:1, for LCNPs with 5 mg/mL plant extract concentration, presented the highest anticandidal activity with MICs ranging from 32 to 256 µg/mL, respectively.
These results showed that these SNPs and LCNPs have good antifungal activity and are significantly correlated with previous reports about the anticandidal potential of SNPs [Citation19]. In addition, the results of anticandidal assays of SNPs and LCNPs when compared with the results of crude extract and fractions showed a drastic increase in potential.
The anticandidal effect could be explained by the smaller size of SNPs and LCNPs with the extremely large surface area which provides good contact and interaction with fungi [Citation1]. It has been reported that silver ions released from silver NPs may penetrate inside the cell membranes damaging nucleic acids, altering cell walls, and producing reactive oxygenated species (ROS) which destroyed metabolic enzymes resulting in the loss of cell viability and ultimately cell death [Citation25,Citation26,Citation28,Citation29].
Antioxidant activity, total phenolic, and total flavonoid content of plant samples, SNPs and LCNPs as well as loading capacity of LCNPs
Different methods, such as DPPH free radical scavenging assay and reducing power are widely used to evaluate the antioxidant capacity of various samples, including green synthesized NPs [Citation27,Citation30]. The antioxidant capacity expressed as IC50 value and total phenolic and the total flavonoid content of plant samples and all the NPs are shown in . In the DPPH assay, the IC50 values were in the range of 1.62–177.70 µg/mL for plant samples, 33.06–508.98 µg/mL for SNPs while it remained higher than 1000 µg/mL in the case of LCNPs. These results indicate that plant samples and SNPs can be used to solve the oxidative stress problems and its associated consequences at the infection site [Citation28,Citation31].
Table 4. Antioxidant activity, total flavonoid and total phenolic content (TFC and TPC) of plant samples, SNPs and LCNPs as well as the loading capacity of LCNPs.
The total phenolic content of plant samples was found in the range of 66.64–624.45 mg GAE/g while the one of LCNPs was in the range of 15.74–47.97 mg GAE/g. The total flavonoid content of plant samples was found in the range of 2.92–51.40 mg EQ/g but the flavonoid content of LCNPs was found negligible. The presence of phenolic and flavonoid compounds in both plant samples, as well as the presence of phenolics compounds in LCNPs, is in agreement with the finding of Tchuenguem et al., who have previously reported the presence of biologically active secondary metabolites like phenols, flavonoids in methanolic extract of E. prostata. Recently many scientists reported that phytochemicals of plants (phenolics and flavonoids compounds) encapsulated in chitosan showed significant biological activities [Citation29,Citation30,Citation32,Citation33].
The loading capacity of phenolic compounds in chitosan NPs was in the range of 2.23–40.30%, while no flavonoids were loaded on chitosan NPs. The LCNPs which showed the best antifungal activity were those with the highest proportions of loaded phenolic compounds, that is NpEPM (4: 1) [40.30%] and NpEPME (4: 1) [3.68%]. Munin and Edwards-Lévy have reported that the amount and quality of phenolic compounds LCNPs might influence their biological activity [Citation31,Citation34].
Conclusion
In the present investigation, a novel approach for bioguided synthesis of SNPs and LCNPs from methanolic extract of E. prostata and its fractions was adopted. The synthesized NPs were spherical in shape with sizes ranging from around 12 to 100 nm as observed in SEM analysis. FTIR analysis revealed the presence of stretching vibration of bonds in the NPs which could be those of the flavonoids and the phenolic compounds present in the plant samples. The synthesized NPs showed significantly high anticandidal activity when compared to the free plant extract. The anticandidal activity of LCNPs was proportional to the loading capacity of phenolic compounds. The plant samples and SNPs showed the highest antioxidant capacity. In short, these results showed that plant extracts and nanoparticles possess the potent biological potential and can be further investigated through in vivo experiments.
Author contributions
Jean P. Dzoyem, Roland T. Tchuenguem, Jamshed Iqbal, Nighat Fatima, and Ghulam Murtaza participated in the research design, while Jean P. Dzoyem, Roland T. Tchuenguem, Jamshed Iqbal, Muhammad Arfat Yameen, Abdul Mannan, and Nighat Fatima worked on data collection and data analysis. Jean P. Dzoyem, Roland T. Tchuenguem, Abdul Mannan, Irum Shahzadi, Tariq Ismail, Nighat Fatima, and Ghulam Murtaza wrote the manuscript. All authors read and approved the final manuscript. In addition, I confirm that all the authors meet the criteria for authorship as per the ICMJE criteria.
Acknowledgements
The authors gratefully acknowledge TWAS-CIIT and the Pharmacy Department of COMSAT Institute of Information Technology (CIIT) Abbottabad campus, Pakistan supporting this work.
Disclosure statement
No potential conflict of interest was reported by the author(s).
Data availability statement
The authors confirm that the data supporting the findings of this study are available within the article.
References
- Rudramurthy GR, Swamy MK, Sinniah UR, et al. Nanoparticles: alternatives against drug-resistant pathogenic microbes. Molecules. 2016;21(7):836–830.
- Suzan A, Mansor BA, Farideh N, et al. Green biosynthesis and characterization of zinc oxide nanoparticles using brown marine Macroalga sargassum muticum aqueous extract. Mater Lett. 2014;116:275–277.
- Ramaswamy U, Mukundan D, Sreekumar A, et al. Green synthesis and characterization of silver nanoparticles using aqueous whole plant extract of Vernonia cinerea L. and its biological activities. Mater Today Proc. 2015;2:4600–4608.
- Kaddour N. No laws in Nanoland: how to reverse the trend? The French example. J Environ Nanotechnol. 2013;30(2):486–522.
- Ghorbani HR. A review of methods for synthesis of Al nanoparticles. Orient J Chem. 2014;30(4):1941–1949.
- Bilia AR, Bergonzi MC, Boulos JC, et al. Nanocarriers to enhance solubility, bioavailability, and efficacy of Artemisinins. World J Tradit Chin Med. 2020;6(1):26–38.
- Davis SA, Vincent BM, Endo MM, et al. Nontoxic antimicrobials that evade drug resistance. Nat Chem Biol. 2015;11(7):481–487.
- Enani MA. Antimicrobial resistance: insights from the declaration of world alliance against antibiotic resistance. Saudi Med J. 2015;36(1):11–12.
- Jabir MS, Hussien AA, Sulaiman GM, et al. Green synthesis of silver nanoparticles from Eriobotrya japonica extract: a promising approach against cancer cells proliferation, inflammation, allergic disorders and phagocytosis induction. Artif Cells Nanomed Biotechnol. 2021;49(1):48–60.
- Hawar SN, Al-Shmgani HS, Al-Kubaisi ZA, et al. Green synthesis of silver nanoparticles from Alhagi graecorum leaf extract and evaluation of their cytotoxicity and antifungal activity. J Nanomater. 2022;2022:1058119.
- Jabir MS, Saleh YM, Sulaiman GM, et al. Green synthesis of silver nanoparticles using Annona muricata extract as an inducer of apoptosis in cancer cells and inhibitor for NLRP3 inflammasome via enhanced autophagy. Nanomaterials. 2021;11(2):384.
- Huh AJ, Kwon YJ. “Nanoantibiotics”: a new paradigm for treating infectious diseases using nanomaterials in the antibiotics resistant era. J Control Release. 2011;156(2):128–145.
- Reidy B, Haase A, Luch A, et al. Mechanisms of silver nanoparticle release, transformation and toxicity: a critical review of current knowledge and recommendations for future studies and applications. Materials. 2013;6(6):2295–2350.
- Amin M, Anwar F, Janjua M, et al. Green synthesis of silver nanoparticles through reduction with Solanum xanthocarpum L. berry extract: characterization, antimicrobial and urease inhibitory activities against Helicobacter pylori. IJMS. 2012;13(8):9923–9941.
- Özdamar B. Immobilization of olive leaf extract on chitosan nanoparticles and investigation of their effects on cancer cell lines [MS thesis]. İzmir Institute of Technology; 2014.
- Zuo TT, Luo FY, Jin HY, et al. Determination of the bioaccessibility of cadmium in golden thread by physiologically based extraction test digestion using the in vitro/Caco2 cell model and subsequent risk assessment. World J Tradit Chin Med. 2021;7:240–245.
- Zia F, Ghafoor N, Iqbal M, et al. Green synthesis and characterization of silver nanoparticles using Cydonia oblong seed extract. Appl Nanosci. 2016;6(7):1023–1029.
- Tchuenguem RT, Kechia FA, Kuiate JR, et al. Ethnopharmacological survey, antioxidant and antifungal activity of medicinal plants traditionally used in Baham locality (Cameroon) to treat fungal infections. Arch Med Biomed Res. 2017;3(2):91–103.
- Jenifer DS, Laveena DK, Priya S, et al. Antimicrobial screening of Euphorbia hirta L. and Pedalium murex L. – a comparative study. World J Pharm Pharm Sci. 2014;3(12):1221–1226.
- Sileikaite A, Prosycevas I, Puiso J, et al. Analysis of silver nanoparticles produced by chemical reduction of silver salt solution. Mater Sci. 2006;12(4):287–291.
- Liu JN, Tidwell T, Zhao HH, et al. Theoretical characteristics of Tibetan medicine. World J Tradit Chin Med. 2020;6(4):490.
- Bodnar M, Hartmann JF, Borbely J. Preparation and characterization of chitosan-based nanoparticles. Biomacromolecules. 2005;6(5):2521–2527.
- Atun S, Handayani S. Synthesis of nanoparticles produced by ethanol extract of Boesenbergia rotunda rhizome loaded with chitosan and alginic acid and its biological activity test. PJ. 2017;9(2):142–147.
- Arya G, Kumari RM, Gupta N, et al. Green synthesis of silver nanoparticles using Prosopis juliflora bark extract: reaction optimization, antimicrobial and catalytic activities. Artif Cells Nanomed Biotechnol. 2018;46(5):985–993.
- Sudha A, Jeyakanthan J, Srinivasan P. Green synthesis of silver nanoparticles using Lippia nodiflora aerial extract and evaluation of their antioxidant, antibacterial and cytotoxic effects. Resour Effic Technol. 2017;3(4):506–515.
- Gao L, Jia CH, Wang W. Recent advances in the study of ancient books on traditional Chinese medicine. World J Tradit Chin Med. 2020;6(1):61.
- Zia G, Sadia H, Nazir S, et al. In vitro studies on cytotoxic, DNA protecting, antibiofilm and antibacterial effects of biogenic silver nanoparticles prepared with Bergenia ciliata rhizome extract. Curr Pharm Biotechnol. 2018;19(1):68–78.
- Chen KX. Academician Kai-Xian Chen talks about the development of traditional Chinese medicine and global medicine. World J Tradit Chin Med. 2020;6(1):1.
- Haider A, Kang IK. Preparation of silver nanoparticles and their industrial and biomedical applications: a comprehensive review. Adv Mater Sci Eng. 2015;2015:1–16.
- Bhakya S, Muthukrishnan S, Sukumaran M, et al. Biogenic synthesis of silver nanoparticles and their antioxidant and antibacterial activity. Appl Nanosci. 2016;6(5):755–766.
- El-Rafie HM, Hamed MA. Antioxidant and anti-inflammatory activities of silver nanoparticles biosynthesized from aqueous leaves extracts of four Terminalia species. Adv Nat Sci Nanosci Nanotechnol. 2014;5:1–11.
- Da Silva SB, Amorim M, Fonte P, et al. Natural extracts into chitosan nanocarriers for rosmarinic acid drug delivery. Pharm Biol. 2015;53(5):642–652.
- Djoueudam FG. Evaluation de l’activité antisalmonelle et antioxydante in vitro et in vivo des feuilles de Solanum torvum Sw. [MS thesis]. Dschang University; 2015.
- Munin A, Edwards-Lévy F. Encapsulation of natural polyphenolic compounds; a review. Pharmaceutics. 2011;3(4):793–829.