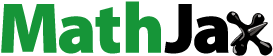
Abstract
Purpose
The present study was carried out to evaluate anti-inflammatory and antiangiogenic attributes of simvastatin and its nanofilms containing silver nanoparticles.
Methods
Silver nanoparticles and simvastatin-loaded nanocomposite (SNSN) films were formulated by using polymeric solution (pectin + sericin) through casting solution method. Different in vitro and in vivo anti-inflammatory assays were performed. In addition, chick chorioallantoic membrane assay (CAM) was also employed for angiogenesis activity.
Results
FTIR spectra of the film depicted the presence of intact simvastatin. Differential scanning calorimetry exhibited no endothermic expression in F9 film thermogram. The simvastatin release from all films exhibited a burst effect. Cotton-pellet induced granuloma model study showed that high dose of simvastatin and indomethacin produced comparable (p < 0.05) anti-inflammatory effect. Noteworthy, RT-PCR showed dose-dependent, anti-oedematous effect of simvastatin through downregulation of serum TNF-α and interleukin-1ß levels. While results of CAM assay exhibited remarkable anti-angiogenic potential of SNSN films showing dissolved blood vessels network macroscopically.
Conclusion
To reiterate, simvastatin and its SNSN films can add significant contribution to the field of biomedicines due to their promising anti-inflammatory and antiangiogenic properties, however, clinical studies are required to validate their commercial use.
Introduction
Inflammation is a natural response of body towards internal and external stimuli. However, an untreated inflammation causes cellular damage resulting in the development of various chronic diseases such as obesity, neurodegenerative diseases, atherosclerosis and cancer [Citation1]. Reportedly, 60% mortality rate in whole world is associated with chronic inflammation [Citation2]. Pathologically, there are few cytokines that promote or inhibit inflammatory process. For example, proinflammatory mediators that promote inflammation include IL-6, IL-13 [Citation3]. On the other hand, anti-inflammatory cytokines that can repress acute and chronic inflammation include interleukins (IL-1Rα, IL-4, IL-10, IL-11) and nuclear factor kappa-β (NF-κβ) [Citation4]. Inflammation plays a vital role in development of cardiovascular diseases such as atherogenesis and atherosclerosis. Few clinical investigations has demonstrated primary role of provocative markers (e.g. TNF-α, interleukin 6, NF-κβ and phospholipase A2) with intracellular lipids concentration [Citation5]. The solid reliance of atherosclerotic process on “acute inflammation” and “lipid abnormalities” allow researchers to explore relationship among hyperlipidemia-related inflammation [Citation6].
In addition, angiogenesis also potentiates inflammation by upregulating expression of angiogenic factors that are considered to be sensitive to inflammogens (). Neovascularization associated inflammation may contribute to tissue growth, abnormal ossification and aggravate response towards pathological insult [Citation7]. Several studies have evidenced that condition of synovitis is inhibited with use of antiangiogenic drugs including paclitaxel, methoxyestradiol and P-38 MAPKI (mitogen-activated protein kinase inhibitors) [Citation8]. Undoubtedly, inflammation and angiogenesis are independent processes, however their coexistence may lead to severe and persistent infections [Citation9]. Recently, there is an expanding number of pre-clinical and clinical studies endeavouring to develop more efficacious and safe agents for treatment of “chronic inflammation reduction trials”. Different strategies are being adopted to discover effective cytokine and leukotrienes inhibitors, chemotaxis preventing agents, mast cells blockers and novel vaccine approaches to boost humoral immunity in body [Citation10].
From therapeutic point of view, main clinically used anti-inflammatory drugs are NSAIDs, salicylates, pyrazoles, pyrazolidines and quinolone derivatives [Citation11]. Noteworthy, from previous two decades, systematic research has explored wide importance of series of synthetic drugs (sulfonamides, statins, monoamine oxidase inhibitors (MAOIs), proteolytic enzyme and cytostatics) in chronic inflammatory conditions, however further studies are required to warrant their use. Although, they are not regarded as anti-inflammatory agents, but they impart supportive action by exerting inhibitory action on cyclooxygenases and prostaglandins synthesis involved in inflammation [Citation12]. Statins “3-hydroxy-3-methyl-glutaryl-CoA inhibitors” are extensively used for management of cardiovascular diseases. Additionally, statins have antioxidant, anti-inflammatory, antitumor, and neuroprotective (pleiotropic effects) properties which suggest that they can target various signalling pathways to exert their great pharmacological effects [Citation13]. HMG-CoA reductase inhibitors impede “mevalonate pathway” resultantly decrease expression of proinflammatory cytokines (e.g. interleukin-6 and tumour necrosis-α) and reactive oxygen species (ROS) in body [Citation14]. While they attenuate angiogenesis by inhibiting endothelial growth factor associated cellular proliferation and migration. These mechanisms may make an important contribution towards statin’s clinical efficacy in inflammatory disorders [Citation15].
Nanoparticles (NPs) are small size materials that can be classified as metallic NPs and polymeric NPs and so on [Citation16]. Generally, the reactivity and mechanical properties of NPs make them suitable candidature for commercial, medical and diagnostic applications. In modern era, use of drug loaded metallic nanoparticles is increasing rapidly in every field of science such as healthcare, optics, chemical industries, sensors and imaging [Citation17]. The nano-drug delivery systems provide a comprehensive scrutiny for discovery and practical application of “nanomaterials” in promoting the efficacy of both traditional and novel drugs [Citation18,Citation19]. In biomedical field, silver nanoparticles (Ag-NPs) based pharmaceutical system is more dominant among all nanocomposites due to their good chemical stability, conductivity, antibacterial and antifungal activities [Citation20]. Multiple studies have been reported antimicrobial action of silver-nanoparticles which may help to mount a defensive response at site of injury through immunomodulatory action. One in vitro study has shown anticancer potential of graphene oxide-silver nanoparticles against A549 and LN229 cell lines by inducing nuclear damage but did not affect nearby healthy cells [Citation21]. Furthermore, Omidi et al. confirmed promising role of silver composite fibres in wound dressings and cosmetic products as antiseptic agent through disruption of enzymatic activities [Citation22].
Simvastatin is an antihyperlipidemic drug and found to be effective as anti-inflammatory agent in various randomised clinical trials [Citation22]. The present research work has additionally attempted to foster alternative use of already clinically approved drugs. Therefore, the current study was designed to provide comprehensive information about therapeutic potential of simvastatin against inflammation. To the best of our information, no study has been carried out yet to explore anti-inflammatory and anti-angiogenic effect of simvastatin nanocomposite films. Over the past few years, polymers-based drug loaded metallic nanocomposites have gained a wide importance due to their ever-growing applications in anti-cancer therapy, gene therapy and antibiotics delivery. As per conventional approach to manage pain and inflammatory disorders, NSAIDs are used twice or thrice in a day which results in poor patient compliance. On the other side, metallic nanoparticles loaded nanocomposites possess certain unique characteristics (e.g. size, shape, high surface-volume ratio and high lipid permeability) which help to deliver medication directly at target site and are easy to use [Citation16,Citation23]. Moreover, polymer-based drug delivery system have attained great success due to modified drug release profile, conductivity and broad pharmacokinetics.
By considering review of literature, the present study was conducted to prepare silver nanoparticles-containing simvastatin-loaded nanofilms (SNSN) by using sericin-pectin polymeric solution. Moreover, anti-oedemtous and antiangiogenic attributes of SNSN films were also investigated through in vivo and ex-vivo studies. Additionally, special focus was paid on drug release behaviour and kinetics of polymers, due to their direct link with drug’s performance and therapeutic outcomes.
Materials and methods
Materials
All chemicals used in this study such as pectin and sericin (Sigma Aldrich®, USA), silver nanoparticles (10 nm (TEM); 0.02 mg/mL in aqueous buffer contains sodium citrate as steriliser; stored 2–8 °C; Lot No. Mkcj6205; Sigma Aldrich®, USA) and glycerol (Sigma Aldrich®) were purchased through local vendors. All chemicals were of analytical grades. Simvastatin drug was gifted by Venus Pharmaceuticals, Lahore, Pakistan.
Preparation of silver nanoparticles containing simvastatin loaded films
Simvastatin-loaded nanocomposites were prepared by using combination of polymeric solution (pectin and sericin) with continuous stirring and heating (). Film flexibility was enhanced with addition of glycerol [Citation24]. Then, silver nanoparticles and simvastatin were added in polymeric solution and poured in petri plates as thin film. Then plates were subjected to dryness in an oven (37 °C) for 5–7 days (). While films of control were also prepared with similar method. Finally, simvastatin loaded and control films were removed from petri plates and wrapped in aluminium foil for future use [Citation24].
Table 1. Formulation table showing the pharmaceutical composition of control and silver nanoparticles containing simvastatin loaded films.
Film thickness of SNSN films
The thickness of simvastatin films was measured individually from various points of each film with the help of vernier calliper. The average of all points was taken as the whole thickness of that specific film [Citation25].
Moisture content of SNSN films
The moisture content of simvastatin films was determined by using an already well-established method with slight modifications [Citation24]. First of all, uniform size pieces of film were excised out and placed in a dried air oven at 105 °C temperature. After 24 h, the weight of film segments was measured again and MC was obtained by using the following equation:
where Wd = initial weight of film segment, Wf = Final film weight.
FTIR testing of SNSN films
The interaction of simvastatin with pectin and sericin was tested by analysing the fabricated film samples in a range of 500–4000 cm−1 using FTIR spectrophotometer (Tensor 25 series FTIR, Germany) [Citation24].
DSC testing of SNSN films
Thermal stability of the fabricated film samples (5 mg ± 1 mg) was tested by subjecting to DSC analysis using calibrated equipment (TGA Q 500 Focus Scientific Solutions, UK). The samples were placed in aluminium pan and their decomposition features were studied at a temperature range of 25–350 °C and heated at a rate of 5 °C per min under nitrogen gas atmosphere. Nitrogen gas purging was done at a rate of 40 ml/min. The change in the weight of sample was recorded with respect to heating temperature. Later, DSC thermograms were acquired by using TA universal analysis 2000 software [Citation26].
Drug content uniformity of SNSN films
Peripheral and central regions (50 mg) of the films were cut, immersed in 50 ml phosphate buffer, and stirred for 24 h. Then the filtered samples were tested using UV-Vis spectrophotometer at λmax = 238 [Citation27].
In-vitro drug release studies of SNSN films
Film samples (50 mg) were enclosed in dialysis bag (MWCO No. 3500–5000 Da) and studied for simvastatin release by using Type II dissolution apparatus (Pharma Labs PT-DT7). Phosphate buffer pH 6.8 (500 ml) at 37 ± 0.5 °C was used as dissolution medium. Dissolution sample (5 ml) was withdrawn and replaced with fresh medium for maintaining sink condition. The filtered samples were tested using UV-Vis spectrophotometer at λmax = 238. To analyse dissolution data, calibration curve was also constructed [Citation27]. Finally, dissolution data was processed through kinetic analysis using various models such as zero order, first order, Higuchi and Korsemeyer-Peppas models.
Anti-inflammatory activities (cotton pellet granuloma model)
Wistar albino rats (male and female) were caged in animal house of Riphah University Lahore, Pakistan, at standard room temperature 25 ± 2 °C under 12 h light/dark cycle. Both genders were used to increase the reproducibility of study outcomes and minimise the chances of clinical trials failure [Citation28]. In this study, all animals were safely administered with pellet diet and water. Rats were kept in an animals house for 1 week prior to the study for acclimatisation purpose. The experiments and protocols for this study were approved by the Ethical Committee of COMSATS University Islamabad (CUI) and further endorsed by Riphah University Lahore campus, Pakistan. Approval No. 879/CUI/PHM-2020 belongs to CUI. Albino rats were divided into five groups (n = 5) for evaluation of anti-inflammatory activities following the given scheme; Group 1: Negative control (received normal diet and water); Group 2: Disease control (received HFD and distilled water); Group 3: Treatment-1 (administered 10 mg/kg simvastatin orally); Group 4: Treatment-2 (administered 20 mg/kg simvastatin orally) and Group 5: Standard (received indomethacin 10 mg/kg orally). Animals were shaved and anaesthetised with injection of ketamine and xylazine (80:20). Then pre-weighed cotton pellets (30 mg) were implanted in axilla surface of rats and sealed the pocket with proline. All defined doses of simvastatin and indomethacin were administered for 10 days. On 11th day, animals were dissected and granuloma tissue containing pellets were excised out and incubated (60 °C) for one day [Citation29]. Percentage inhibition was calculated by using following formula:
Measurement of TNF-α and IL-Iβ levels through RT-PCR technique
Real-Time Polymerase Chain Reaction (QuantStudio3 Kits) technique was used to measure the levels of inflammatory biomarkers such as TNF-α and IL-Iβ. Samples were prepared according to manufacturer’s protocol provided on kits outlet and microplate was read on an automatic 96-well thermal cycler (Applied Biosystems Thermo Scientific®) at 450 nm [Citation30].
Chicken chorioallantoic membrane (CAM) assay
Twenty-four 3–4 days fertile white leghorn chicken eggs were purchased from Batt hatchery, Sui-Asal road, Lahore. After cleaning the eggs with ethanol all were incubated for one day at 37 °C temperature and 80% relative humidity. Groups 1–10 were labelled as simvastatin loaded silver nanoparticles films. While group 11 and 12 containing pure simvastatin (100 and 200 µg/mL dose) and 13 group was taken as control (ethanol). Then by using sterile scissor a round shaped window was generated on wide side of eggshell and discs of simvastatin films, pure compound (simvastatin) and control were placed in respective eggs with help of sterile forceps. The window was closed with paper tape and subjected to incubation for 48 h. and then reopened. Assay was performed in triplicate. Digitalised pictures were captured from all groups and effect of test drug on angiogenesis was analysed by calculating increase or decrease in blood vessels amount [Citation31].
Statistical analysis
Data is analysed through “One-way ANOVA” followed by Post-Hoc Tuckey’s test and results are presented as mean ± SEM (standard error of the mean) after statistical analysis through Graph-Pad Prism Software. “p” value less than .05 is significant.
Results
Physical analysis of films
The water content in silver nanoparticles containing simvastatin loaded films ranged between 12.5 ± 0.1 and 62.5 ± 0.1. There is a decrease in moisture content (%) with a decrease in pectin quantity in the prepared films. It could be due to the fact that the crystalline structure of matrices is increased with absorption of water; however, modulus and tensile strength are decreased (plasticization effect). Data in is shown order of moisture content (%) in ascending order as F1 > F9 > F2 > F5 > F8 > F7 > F3 > F4 > F6 > F10. While thickness (in millimeters) of all films was ranged from 0.99 ± 0.1 to 1.11 ± 0.1, respectively ().
Table 2. Summary of primers used in RT-PCR.
Table 3. Moisture content of silver nanoparticles containing simvastatin loaded films.
FTIR testing of SNSN films
The FTIR spectra of simvastatin and the developed film are presented in . The main absorption peaks of all film spectra were resembling that of pure simvastatin. It suggested the loading of drug in intact form to the nanocomposite system. Simvastatin spectra depicts a broad band at 3546.40 cm−1 (free O–H stretching vibration), 2929.78 cm−1 (free C–H stretching vibration), 1694.98 cm−1, 1466.02 cm−1, 1267.11 cm−1 (stretching vibration of ester functional group), 1160.23 cm−1 (stretching vibration of lactone carbonyl functional group) and 1070.99 cm−1, which co-relate with the peaks of pure simvastatin.
DSC testing of SNSN films
The DSC thermograms of the composite films are presented in . DSC analysis was conducted to assess thermal stability of the fabricated films and to elaborate the changes occurred in their thermal characteristics upon the procedural activities. DSC thermogram of F8 film shows a melting endotherm at 117.25 °C. F9 film contains 400 mg simvastatin. The intensity of this endothermic curve is reduced in F10 film thermogram, likely since F10 film contains 300 mg simvastatin. There is no endothermic expression in F9 film thermogram. F9 film does not contain simvastatin. Moreover, the presence of silver nanoparticles (F5) did not affect this endotherm. These findings show that there is no chemical interaction of drug with other ingredients.
Drug content uniformity of SNSN films
Drug loading efficiency of all film samples varied from 99.10 + 0.15% to 99.43 + 0.21%. Moreover, there was uniform distribution of drug i.e. non-significant difference in drug loading efficiency in the centre and proximity of all film samples. These findings were in accordance with the previous findings [Citation14].
In-vitro drug release studies of SNSN films
Simvastatin release from all films exhibited a burst effect, i.e. 100% drug release was recorded within initial 5 min. This fast release of drug is likely due to hydrophilic nature of pectin and sericin, which absorb water immediately on meeting dissolution medium and allow the release of drug. Polymer chains undergo relaxation on coming in contact with dissolution medium resulting in the deprotonation of their amino groups, leading to film swelling, and thus faster liberation of drug molecules is achieved [Citation10]. Kinetic analysis revealed that dissolution data was best fit to first order model. The value of “n” was less than 0.45 that indicated the Fickian diffusion-based drug release.
Anti-inflammatory activity (cotton pellet induced granuloma assay)
data represented that percentage of inflammation inhibition of simvastatin (10 and 20 mg/kg) and indomethacin treatment groups was 54.8 ± 0.5, 82.9 ± 1.6 and 86.4 ± 1.7, respectively. By comparing simvastatin 20 mg/kg with standard, statistically non-significant (p > .05) difference was analysed. However, comparison of higher dose (20 mg/kg) of simvastatin with lower dose (10 mg/kg) showed significant (p < .001) reduction in granuloma formation. Hence, simvastatin exhibited dose dependent anti-granulomatous effect throughout the study (; and ).
Figure 6. Effects of simvastatin and indomethacin in cotton pellet induced granuloma model. Values shown are Mean ± SEM of percentage inhibition of granuloma formation (n = 4). Where *** = p ˂ .001 and ns = p > .05. Black colour star means comparison between different doses of simvastatin (10 vs 20 mg/kg). While red colour star means comparison of simvastatin 20 mg/kg with indomethacin 10 mg/kg respectively.
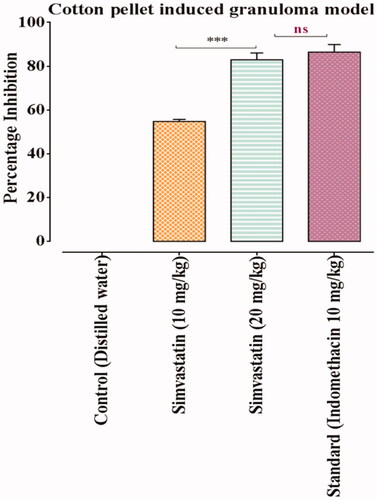
Table 4. Anti-inflammatory effects of simvastatin in cotton pellet induced granuloma model.
Measurement of TNF-α and IL-Iβ levels
Data in represented serum levels of TNF-α in control, simvastatin and standard treatment groups (10 and 20 mg/kg) as 65.1 ± 0.3, 49.4 ± 0.6, 35.0 ± 0.1 and 33.59 ± 0.1 respectively. While levels of IL-1β in control, simvastatin 10 and 20 mg/kg and indomethacin treated animals was estimated to be 48.2 ± 0.5, 44.3 ± 1.1, 35.8 ± 0.4 and 20.19 ± 2.0 in respective way. Thus, animals treated with simvastatin showed significant reduction in concentration of proinflammatory mediators (TNF-α and IL-1β) in dose dependent manner as compared to diseased control group. While 20 mg/kg dose of simvastatin showed comparable effects with indomethacin treatment group (standard) ().
Table 5. Serum TNF-α and IL-1ß levels in different treatment groups.
Antiangiogenic assay
Data in exhibit an inhibitory effect of SNSN films on angiogenesis process. Findings showed following values of percentage inhibition in SNSN-treated groups 52.4% (F1), 88.5% (F2), 100.0% (F3), 92.6% (F4), 100.0% (F5 and F6), 95.9% (F7), 86.1% (F8), 100.0% (F9 and F10) respectively. Moreover, F3, F5, F6, F9 and F10 demonstrated significant (p < .001) increase in percentage inhibition as compared to other formulations. While F1 film showed sudden decrease in percent inhibition of blood vessels network as compared to all others. In addition, pure drug of simvastatin showed 56.1% and 88.5% percentage inhibition in 100 and 200 µg/mL treatment groups respectively which is attributed its dose dependent antiangiogenic effect ( and ; ).
Figure 8. The results of CAM assay after treatment with two doses of simvastatin (100 and 200 µg/mL). In simvastatin treatment groups, dissolved blood vasculature showed its antiangiogenic effect. While ethanolic discs containing control group showed intact blood vessels network.
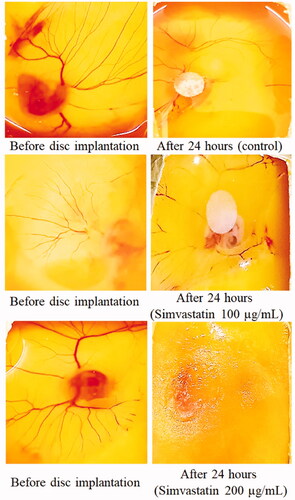
Table 6. Antiangiogenic effect of SNSN films on blood vasculature network.
Discussion
Statins or 3-hydroxy-methyl-glutaryl coenzyme-A (HMG CoA) reductase inhibitors possess “pleiotropic effect” in multiple signalling processes. To embark, the present research was carried out to validate the anti-inflammatory and antiangiogenic attributes of simvastatin. In recent times, nanotechnology is booming by leaps and bounds due to emergence of “nanoparticles” having unique functions as compared to their bulk [Citation32]. Furthermore, silver nanoparticles have gained more attention of researchers due to their high thermal and electrical conductivity, chemical stability, catalytic activity and kinetic behaviour. Therefore, this study also focussed on formulation of simvastatin loaded silver nanoparticles containing films and to analyse their pharmacological effects towards inflammation related angiogenesis. While additional glycerol makes formulation sweeten and provides support in the achievement of sufficient films thickness [Citation33].
Thin films can act both locally and systemically and are convenient to use because of their “easy handling, fast dissolvability and self-administrating” properties [Citation34]. The formation of thin film has become subject of extensive research to understand the physical characteristics of drug loaded films. As volume of films increases, distance between particles decreases which can reduce “interchain entanglement” [Citation35]. Studies have revealed toxicity of silver nanoparticles is linked with their size and shape. One study reported that particle size more than 15 nm may induce morphological alteration ultimately oedema, hyperplasia and focal inflammation. Furthermore, exposure of intracellular environment with Ag-NPs produces an increase in IL-1β, IL-6, IL-8 and TNF-α. Three basic mechanisms related to Ag-nanoparticles are DNA damage, cytokine induction and oxidative stress [Citation36,Citation37]. Most of the information about biosafety of Ag-nanoparticles come from in vitro studies and considerable attention should be directed towards fate of Ag-NPs through in vivo models [Citation38]. Moreover, silver nanoparticles possess strong surface functionalization that elicit their escalated and extensive use for therapeutic purposes [Citation39]. In the present study, silver nanoparticles were of 10 nm in size, attributed its safety for ex-vivo studies. Moisture contents indicates water contents present in the composite film and have a primary role in maintaining the integrity, texture, quality, and mechanical properties of nanocomposites. Our findings suggested significant variation among thickness of films which is suggested drug releasing behaviour, chemical and physical characteristics of nanofilms. Among all statins, “simvastatin” is eminent in clinical use. Moreover, apart from hypolipidemic effect of statins numerous studies have shown anti-inflammatory, antioxidant, antiproliferative, antiplatelet and immunomodulatory properties of this class. Statins regulate innate immune system by exerting effect on endothelial cells, macrophages, natural killer cells, and neutrophils [Citation40]. Furthermore, studies have shown that statins produce anti-inflammatory effect by decreasing protein prenylation ultimately reduce expression of transcription factors TNF-α and IL-1ß.
Recently, clinical trials have been exhibited beneficial effects of statins towards inflammation through inhibition of leukocytes migration, MHC-2 downregulation and reducing cytokines expression. These mechanistic approaches allow statin to be used as therapeutic candidature for inflammatory diseases such as arthritis, sepsis, diabetes mellitus and neurodegenerative problems. Previous pre-clinical study confirmed safety profile of simvastatin in rats at dose level of 100 mg/kg thus the current study used 1/10th of this dose during in vivo experiment [Citation41,Citation42]. In present study, simvastatin showed dose dependent effect on granuloma formation in cotton pellet induced granuloma model by suppressing release of proinflammatory mediators as evidenced from earlier studies. Furthermore, inhibitory effect of simvastatin on TNF-α and interleukin-1ß (IL-1ß) signalling pathways was also evidenced from RT-PCR technique. Consequently, enhance our understanding about anti-inflammatory activity of simvastatin on “vascular pathology” beyond their lipid lowering effect. In addition, SNSN nanofilms suppressed the expression of TNF-α and IL-1ß through the transcription factor NF-kβ pathway [Citation43].
Multiple scientific reports have demonstrated strong link of angiogenesis with inflammatory diseases like rheumatoid arthritis, osteoarthritis, asthma, cancer and pulmonary fibrosis. Round about 25% of cancers possess chronic inflammation as major pathological cause due to secretion of inflammatory biomarkers and genetic mutations [Citation44,Citation45]. Moreover, tumour-associated macrophages (TAMs) secrete proangiogenic factors which ultimately lead to angiogenesis and oxygen deficit conditions. Metallo-proteinases secreted by macrophages cause angiogenesis and metastasis which lead to cancer development [Citation46]. Therefore, the prevention from long-lasting inflammation can impede abnormal neovascularization and turn aside tumorgenesis [Citation47].
Chorioallontoic membrane (CAM) assay is a common and economical test used to assess the effect of test drug on blood vessels growth [Citation29]. In the present research, CAM assay revealed significant inhibitory action of silver nanoparticles containing simvastatin loaded films and simvastatin (pure compound) on blood vessels growth which make it a potential candidate for treatment of inflammatory disorders. While ethanol disc of control group continued development of vessels plexus by increasing expression of vascular endothelial growth factor as described in earlier studies. Altogether, due to the repressive action of simvastatin on angiogenesis and inflammation it can be considered a good anti-cancerous agent in the future. However, SNSN film F9 exhibited very less inhibition towards angiogenesis as this formulation did not contain simvastatin. In addition, long clinical trials are still needed to confirm the antiangiogenic properties of simvastatin nanocomposites. Besides this, nanostructure films serve as “barrier materials” which provide an elegant plush feel to the carrier and allow the inclusion of wide variety of ingredients. Moreover, these “bioactive medical devices” inherently resist chronic pathologies and dramatically control the drug release profile and rheological properties of drugs. In current study polymer-based silver nanoparticles containing simvastatin loaded films were prepared to achieve the superb effect of simvastatin against chronic ailments by enhancing drug solubility, promoting therapeutic efficacy and reducing cytotoxicity. Owning to these all benefits, our study significantly enhances the potential utility of thin film system, however, a remaining challenge is to better understand the “biological response” of film-embedded bioactive agent e.g. cell sensitivity, surface linked polymers layer and bonding between nanofilms and bioactive moiety [Citation48].
Conclusion
To summarise, pure simvastatin has significant anti-inflammatory effect against granuloma formation. RT-PCR technique revealed inhibitory action of simvastatin on TNF-alpha and IL-1β signalling pathways. Moreover, CAM assay ensured antiangiogenic attributes of SNSN films and pure compound (simvastatin). The kinetic profile, ex-vivo and in vivo experiments altogether support anti-inflammatory and antiangiogenic attributes of pure simvastatin and SNSN films, Hence, controllable bioactivity of simvastatin and mechanical strength of Ag-NPs containing SNSN films offer great promise to interface material and biological world.
Ethical approval
The experiments and protocols for this animal study were approved by the Ethical Committee of COMSATS University Islamabad (CUI) with the approval No. 879/CUI/PHM-2020.
Authors’ contributions
Manal Buabeid, El-Shaimaa A. Arafa and Ghulam Murtaza had substantial contributions to the conception or design of the work and interpretation of data for the work; Hafiza Sidra Yaseen and Muhammad Ihtisham Umar were involved in the acquisition, analysis, or interpretation of data for the work; Manal Buabeid, Hafiza Sidra Yaseen and Muhammad Ihtisham Umar drafted the work and revised it critically for important intellectual content and finally approved the version to be published; Manal Buabeid and Ghulam Murtaza agrees to be accountable for all aspects of the work in ensuring that questions related to the accuracy or integrity of any part of the work are appropriately investigated and resolved.
Disclosure statement
No potential conflict of interest was reported by the author(s).
Data availability statement
The data that support the findings of this study are available from the corresponding author, [GM], upon reasonable request.
Correction Statement
This article has been republished with minor changes. These changes do not impact the academic content of the article.
Additional information
Funding
References
- Bilia AR, Bergonzi MC, Boulos JC, et al. Nanocarriers to enhance solubility, bioavailability, and efficacy of artemisinins. World J Tradit Chin Med. 2020;6(1):26–38.
- Osimo EF, Baxter LJ, Lewis G, et al. Prevalence of low-grade inflammation in depression: a systematic review and meta-analysis of CRP levels. Psychol Med. 2019;49(12):1958–1970.
- Mantovani A, Dinarello CA, Molgora M, et al. Interleukin-1 and related cytokines in the regulation of inflammation and immunity. Immunity. 2019;50(4):778–795.
- Ferrara M, Chialli G, Ferreira LM, et al. Oxidation of HMGB1 is a dynamically regulated process in physiological and pathological conditions. Front Immunol. 2020;11:1122.
- Montag C, Wagner J, Gruska I, et al. Human cytomegalovirus blocks tumor necrosis factor alpha-and interleukin-1β-mediated NF-κB signaling. J Virol. 2006;80(23):11686–11698.
- Koushki K, Shahbaz SK, Mashayekhi K, et al. Anti-inflammatory action of statins in cardiovascular disease: the role of inflammasome and toll-like receptor pathways. Clin Rev Allergy Immunol. 2021;60(2):175–199.
- Walsh DA, Pearson CI. Angiogenesis in the pathogenesis of inflammatory joint and lung diseases. Arthrit Res Ther. 2001;3(3):1–7.
- Taniguchi K, Karin M. NF-κB, inflammation, immunity and cancer: coming of age. Nat Rev Immunol. 2018;18(5):309–324.
- Wang C, Wang Q, Gao W, et al. Highly efficient local delivery of endothelial progenitor cells significantly potentiates angiogenesis and full-thickness wound healing. Acta Biomater. 2018;69:156–169.
- MacDonald IJ, Liu S-C, Su C-M, et al. Implications of angiogenesis involvement in arthritis. IJMS. 2018;19(7):2012.
- Sostres C, Gargallo CJ, Arroyo MT, et al. Adverse effects of non-steroidal anti-inflammatory drugs (NSAIDs, aspirin and coxibs) on upper gastrointestinal tract. Best Pract Res Clin Gastroenterol. 2010;24(2):121–132.
- S Antonopoulos A, Margaritis M, Lee R, et al. Statins as anti-inflammatory agents in atherogenesis: molecular mechanisms and lessons from the recent clinical trials. Curr Pharm Des. 2012;18(11):1519–1530.
- Ashrafizadeh M, Ahmadi Z, Farkhondeh T, et al. Modulatory effects of statins on the autophagy: a therapeutic perspective. J Cell Physiol. 2020;235(4):3157–3168.
- Sparrow CP, Burton CA, Hernandez M, et al. Simvastatin has anti-inflammatory and antiatherosclerotic activities independent of plasma cholesterol lowering. ATVB. 2001;21(1):115–121.
- Gilbert R, Al-Janabi A, Tomkins-Netzer O, et al. Statins as anti-inflammatory agents: a potential therapeutic role in sight-threatening non-infectious uveitis. Porto Biomed J. 2017;2(2):33–39.
- Tariq A, Bhawani SA, Moheman A. Nanoparticles for drug delivery. In: Bhat A, Khan I, Jawaid M, Suliman F, Al-Lawati H, Al-Kindy S, editors. Nanomaterials for healthcare, energy and environment. Singapore: Springer; 2019. p. 175–197.
- Ahmed S, Ahmad M, Swami BL, et al. A review on plants extract mediated synthesis of silver nanoparticles for antimicrobial applications: a green expertise. J Adv Res. 2016;7(1):17–28.
- Patra JK, Das G, Fraceto LF, et al. Nano based drug delivery systems: recent developments and future prospects. J Nanobiotechnol. 2018;16(1):1–33.
- Jabir MS, Hussien AA, Sulaiman GM, et al. Green synthesis of silver nanoparticles from eriobotrya japonica extract: a promising approach against cancer cells proliferation, inflammation, allergic disorders and phagocytosis induction. Artif Cells Nanomed Biotechnol. 2021;49(1):48–60.
- Bhavyasree P, Xavier T. Green synthesis of copper oxide/carbon nanocomposites using the leaf extract of Adhatoda vasica Nees, their characterization and antimicrobial activity. Heliyon. 2020;6(2):e03323.
- Mariadoss AVA, Ramachandran V, Shalini V, et al. Green synthesis, characterization and antibacterial activity of silver nanoparticles by Malus domestica and its cytotoxic effect on (MCF-7) cell line. Microb Pathog. 2019;135:103609.
- Omidi S, Sedaghat S, Tahvildari K, et al. Biosynthesis of silver nanocomposite with tarragon leaf extract and assessment of antibacterial activity. J Nanostruct Chem. 2018;8(2):171–178.
- Raj S, Khurana S, Choudhari R, et al. Specific targeting cancer cells with nanoparticles and drug delivery in cancer therapy. Amsterdam: Elsevier; 2021. p. 166–177.
- Shah A, Yameen MA, Fatima N, et al. Chemical synthesis of chitosan/silver nanocomposites films loaded with moxifloxacin: their characterization and potential antibacterial activity. Int J Pharm. 2019;561:19–34.
- Ullah K, Khan SA, Mannan A, et al. Enhancing the antibacterial activity of erythromycin with titanium dioxide nanoparticles against MRSA. Curr Pharm Biotechnol. 2020;21(10):948–954.
- Shah A, Hussain I, Murtaza G. Chemical synthesis and characterization of chitosan/silver nanocomposites films and their potential antibacterial activity. Int J Biol Macromol. 2018;116:520–529.
- Shah A, Ashames AA, Buabeid MA, et al. Synthesis, in vitro characterization and antibacterial efficacy of moxifloxacin-loaded chitosan-pullulan-silver-nanocomposite films. J Drug Delivery Sci Technol. 2020;55:101366.
- Lee SK. Sex as an important biological variable in biomedical research. BMB Rep. 2018;51(4):167–173.
- Yaseen HS, Asif M, Saadullah M, et al. Methanolic extract of ephedra ciliata promotes wound healing and arrests inflammatory Cascade in vivo through downregulation of TNF-α. Inflammopharmacology. 2020;28(6):1691–1704.
- Saleem M, Iftikhar A, Asif M, et al. Asphodelus tenuifolius extracts arrested inflammation and arthritis through modulation of TNF-α, NF-κB, ILs, and COX-2 activities in in vivo models. Inflammopharmacology. 2021;29(2):483–497.
- Saleem M, Asif M, Parveen A, et al. Investigation of in vivo anti-inflammatory and anti-angiogenic attributes of coumarin-rich ethanolic extract of melilotus indicus. Inflammopharmacology. 2021;29(1):281–293.
- Sarwar MS, Huang Q, Ghaffar A, et al. A smart drug delivery system based on biodegradable chitosan/poly (allylamine hydrochloride) blend films. Pharmaceutics. 2020;12(2):131.
- Yang YP, Tasneem S, Daniyal M, et al. Lanostane tetracyclic triterpenoids as important sources for anti-inflammatory drug discovery. World J Tradit Chin Med. 2020;6(3):229–238.
- Karki S, Kim H, Na S-J, et al. Thin films as an emerging platform for drug delivery. Asian J Pharm Sci. 2016;11(5):559–574.
- Gurmessa BJ. Buckling instabilities of nanoscale polymer films and colloidal particle layers. Fargo, ND: North Dakota State University; 2015.
- Stensberg MC, Wei Q, McLamore ES, et al. Toxicological studies on silver nanoparticles: challenges and opportunities in assessment, monitoring and imaging. Nanomedicine. 2011;6(5):879–898.
- Ferdous Z, Nemmar A. Health impact of silver nanoparticles: a review of the biodistribution and toxicity following various routes of exposure. IJMS. 2020;21(7):2375.
- Zhang X-F, Liu Z-G, Shen W, et al. Silver nanoparticles: synthesis, characterization, properties, applications, and therapeutic approaches. IJMS. 2016;17(9):1534.
- Allawadhi P, Singh V, Khurana A, et al. Silver nanoparticle based multifunctional approach for combating COVID-19. Sens Int. 2021;2:100101.
- Sparrow C, Burton C, Hernandez M, et al. Simvastatin has anti-inflammatory and anti-atherosclerotic activities independent of plasma cholesterol-lowering. Atherosclerosis. 2000;151(1):188.
- Wierzbicki AS, Mikhailidis DP. Dose–response effects of atorvastatin and simvastatin on high-density lipoprotein cholesterol in hypercholesterolaemic patients: a review of five comparative studies. Int J Cardiol. 2002;84(1):53–57.
- MacDonald JS, Halleck MM. The toxicology of HMG—CoA reductase inhibitors: prediction of human risk. Toxicol Pathol. 2004;32(2_suppl):26–41.
- Lee S-Y, Cho S-S, Li Y, et al. Anti-inflammatory effect of Curcuma longa and Allium hookeri co-treatment via NF-κB and COX-2 pathways. Sci Rep. 2020;10(1):1–11.
- Brücher BL, Jamall IS. Chronic inflammation evoked by pathogenic stimulus during carcinogenesis. 4open. 2019;2:8.
- Brezani V, Smejkal K, Hosek J, et al. Anti-inflammatory natural prenylated phenolic compounds-potential lead substances. Curr Med Chem. 2018;25(10):1094–1159.
- Keibel A, Singh V, Sharma MC. Inflammation, microenvironment, and the immune system in cancer progression. Curr Pharm Des. 2009;15(17):1949–1955.
- Murakami M, Hirano T. The molecular mechanisms of chronic inflammation development. Front Immunol. 2012;3:323.
- Jiang C, Wang T, Xu ZJ, et al. Investigation on the mode of action of the traditional Chinese medical prescription-Yiqihuoxue formula, an effective extravasation treatment for cerebral vascular microemboli in ApoE-/- mice. World J Tradit Chin Med. 2020;6(1):112–120.