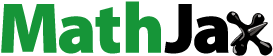
Abstract
Doxorubicin (DOX) is an effective chemotherapeutic agent widely used for cancer treatment. However, hypoxia in tumour tissue and obvious adverse effects particularly cardiotoxicity restricts the clinical usage of DOX. Our study is based on the co-administration of haemoglobin-based oxygen carriers (HBOCs) and DOX in a breast cancer model to investigate HBOCs’ ability to enhance chemotherapeutic effectiveness and its capabilities to alleviate the side effects induced by DOX. In an in-vitro study, the results suggested the cytotoxicity of DOX was significantly improved when combined with HBOCs in a hypoxic environment, and produced more γ-H2AX indicating higher DNA damage than free DOX did. Compared with administration of free DOX, combined therapy exhibited a stronger tumour suppressive effect in an in-vivo study. Further mechanism studies showed that the expression of various proteins such as hypoxia-inducible factor-1α (HIF-1α), CD31, CD34, and vascular endothelial growth factor (VEGF) in tumour tissues was also significantly reduced in the combined treatment group. In addition, HBOCs can significantly reduce the splenocardiac toxicity induced by DOX, according to the results of the haematoxylin and eosin (H&E) staining and histological investigation. This study suggested that PEG-conjugated bovine haemoglobin may not only reduce the hypoxia in tumours and increase the efficiency of chemotherapeutic agent DOX, but also alleviate the irreversible heart toxicity caused by DOX-inducted splenocardiac dysregulation.
Introduction
A major cause of morbidity and mortality in the world, cancer is defined as a condition characterized by the unchecked growth and spread of abnormal cells, which are brought on by both external and internal factors, such as an inadequate diet, inherited mutations from radiation and tobacco use, etc. [Citation1,Citation2]. Proliferating cells and the stroma, which comprise connective tissue and blood vessels, make up tumours [Citation3]. When a tumour becomes malignant, it can trigger angiogenesis, which enables it to develop a blood supply for feeding itself with vital nutrients and oxygen for more growth at the secondary sites [Citation4,Citation5]. The intra-tumour microenvironment becomes strikingly hypoxic due to the imbalance between the blood supply and the tumour’s rapid rate of growth, which reduces the oxygen content [Citation6].
Hypoxia is a common and important characteristic of solid tumours, which has been linked to the growth and spread of numerous cancers [Citation7]. The local microenvironment may become even more distorted as a result of the hypoxic microenvironment’s strong correlation with the advancement of cancer, enhanced aggressiveness, and intense spreading potential [Citation8]. Since many common cytotoxic anticancer therapies are inhibited by the aberrant microenvironment, such as resistance to radio- or chemotherapy, it also indicates a lesser chance of therapeutic success for a variety of tumour types [Citation9–11]. Doxorubicin (DOX), a kind of anthracycline, is a potent chemotherapeutic drug that has been the cornerstone of therapies for both early-stage and metastatic breast cancer and has been licenced by the FDA for clinical usage [Citation1,Citation12,Citation13]. As one of the anthracycline family of antibiotics, there are two confirmed mechanisms for DOX antitumor effect [Citation14]. The first mechanism is that interacts with the DNA and inhibits the progression of the enzyme topoisomerase II for transcription and topoisomerase II-mediated DNA repair, thereby further stopping the replication [Citation13]. The second mechanism involves the generation of reactive oxygen species (ROS), resulting in oxidative stress intracellular environment [Citation13,Citation15]. Both pathways depend on oxygen consumption, hence one of the factors limiting the therapeutic application of DOX is hypoxia in tumour tissue [Citation16]. One of the most crucial ways to boost the effectiveness of radiation, chemotherapy, and other oxygen-consuming methods is to increase tumour oxygenation, which could virtually assist cancer patients [Citation8].
To replace blood transfusions, haemoglobin-based oxygen carriers (HBOCs) demonstrate the remarkable capabilities of native haemoglobin in carrying and delivering oxygen (O2) as well as the potential to be employed as oxygen therapy to treat specific hypoxic ischaemic disorders [Citation17]. HBOCs have been tested to improve the effectiveness of radiotherapy and chemotherapy administration [Citation18]. According to Teicher et al. [Citation19], ultra-purified polymerized bovine haemoglobin solution can boost radiation and chemotherapy responsiveness while alleviating tumour hypoxia. According to Alfredo Lucas et al. PolyHb, a kind of HBOCs, may lower chemotherapy dosages of cisplatin and enhance tumour oxygenation and ROS production [Citation17,Citation20]. HBOCs and DOX may therefore be a good combination for chemotherapy and tumour oxygenation.
However, DOX is associated with clear side effects such as nausea, myelosuppression, baldness, stomatitis, and toxicity, especially cardiotoxicity, which is another significant restriction limiting the use of DOX [Citation12,Citation15,Citation21–24]. Cardiomyopathy is the most dangerous side effect induced by the cardiotoxicity of DOX, which could lead to congestive heart failure associated with mortality and morbidity in the worst-case scenario [Citation25]. Unfortunately, the precise mechanisms of DOX-induced cardiotoxicity is not explicitly studied [Citation26–28]. There are many factors and pathways related to the DOX-induced cardiotoxicity. Mitochondrial damage, iron accumulation, inflammatory pathways, oxidative stress, dysregulation of intracellular calcium, DNA breaks have fatal roles in the creation of DOX-induced cardiotoxicity [Citation25]. But a few medications were used to treat the DOX-induced cardiotoxicity in clinical settings [Citation29,Citation30]. Therefore, significant progress should be achieved in the development of novel therapeutic approaches that increase tumour cytotoxicity and minimize side effects [Citation8,Citation31,Citation32].
Current studies were mainly focused on the improvement of tumour hypoxic microenvironment and the enhancement of the chemotherapeutic efficacy via the oxygen-releasing of HBOCs, but most researchers have rarely paid any attention to the alleviation of side effects to the major organ induced by the DOX treatment when using the HBOCs to enhance chemotherapy. Herein, our focus has been not only on the improvement of tumour hypoxia and enhancement of DOX chemotherapeutic efficacy via PEG-conjugated bovine haemoglobin (PCBH), but also on the alleviation of splenocardiac dysregulation in the mice treated with different treatments.
Materials
PEG-conjugated bovine haemoglobin (PCBH) was received from Zhong Bao Mu biotechnology Co. Ltd (Changchun, China). Doxorubicin (DOX) was purchased from Med Chem Express Co. Ltd (Shanghai, China). Bovine haemoglobin (bHb) was purified as in our previous studies [Citation33]. Dulbecco’s modified Eagle’s medium (DMEM), 1640 culture medium, Dulbecco’s phosphate-buffered saline (PBS), 0.25% trypsin-EDTA and 100 × penicillin antibiotic solution were purchased from Sigma-Aldrich Co. Ltd (St Louis, USA). Heat-inactivated foetal bovine serum (FBS) was procured from ExCell Bio Co. Ltd (Guangzhou, China). Cell Counting Kit-8 (CCK-8) and γ-H2AX assay were purchased from, Dojindo Co. Ltd (Tokyo, Japan). HIF-1α, P-gp, VEGF, CD31, CD34, and Ki-67 assay kits were purchased from Beyotime Biotechnology Co. Ltd (Shanghai, China). The chemicals were analytical grade and utilized without any additional purification. All the experiments involved the use of Milli-Q (Millipore Corporation, CA, USA) water.
Oxygen equilibrium curves
An Oxygenation-dissociation Analyzer (BLOODOX-2018 Analyzer, Softron Biotechnology, Beijing, China) was employed to obtain Oxygen equilibrium curves (OECs) to measure changes in the binding affinity of O2 in bHb and PCBH [Citation33].
Oxygen dissociation assay (ODA)
The Hb’s spectral fingerprints (Soret and Q bands, 400–450 and 500–600 nm, respectively) were used to gauge the amount of oxygenated Hb and PCBH produced during deoxygenation. In 96-well plates, purified bHb or PCBH (Hb = 0.75 mg/ml) was allowed to incubate for 1 h at ambient air temperature and 7.4 pH. Samples were deoxygenated with N2 for two or five hours at 37 °C and then spectral measurements (380–700 nm) were taken to measure the OxyHb level using a plate reader (SPECTROstar Nano, BMG Labtech NC, USA) in every 30 s. Results of the data analysis were represented as % OxyHb [Citation34].
The oxyhaemoglobin (OxyHb), deoxyhemoglobin (DeoxyHb), and Methaemoglobin (MetHb) concentrations were calculated using the following equations:
The changes in OxyHb percentage in the dynamic oxygen environment were calculated as follows:
Cell line and animals
MCF-7ADR human breast cancer cells and 4T1 mouse breast tumour cells were purchased from Anweisci Biotechnology Co. Ltd (Shanghai, China). Female BALB-c nude mice (6–8 weeks) were purchased from SPF Biotechnology Co. Ltd (Beijing, China). All animal experiments conformed to the Guide for the Care and Use of Laboratory Animals and approved by the Institutional Animal Care and Use Committee of the Academy of Military Medical Sciences.
Cell culture
MCF-7ADR cells and 4T1 cells were cultured in DMEM and 1640 culture medium containing 10% FBS and 1% antibiotic–antimycotic respectively under normoxic conditions (95% air, 5% CO2).
Cell viability and the γ-H2AX assay
Cell viability was detected by the CCK-8 assay. 1 × 105 4T1 cells per well of 96-well plates were cultured with 150 μL medium overnight under normoxic conditions (95% air, 5% CO2) and hypoxic conditions (1% O2, 5% CO2, and N2 balanced). Subsequently, the cells were treated with doxorubicin (0.15, 0.3, 0.6, 1.2 μg/well), bHb and PCBH (7.5, 15, 30 and 60 μg/well). Following the completion of the treatments for 6, 12, and 24 h, cell viability was evaluated using a microplate reader in accordance with the manufacturer’s recommendations.
The γ-H2AX in 4T1 cells was detected by the γ-H2AX assay. 1.2 × 106 4T1 cells per well of 6-well plates were cultured with 2.5 ml medium overnight under normoxic conditions (95% air, 5% CO2) and hypoxic conditions (1% O2, 5% CO2, and N2 balanced). Subsequently, the cells were treated with doxorubicin (8 μg/mL), bHb and PCBH (400 μg/mL). Following the completion of the treatments for 6 h, the γ-H2AX was evaluated using a fluorescence microscope (ECHO Revolve-100, ECHO, USA) in accordance with the manufacturer’s recommendations.
Animal studies
The murine 4T1breast cancer model
In a mouse model, 6 × 106 4T1 cells with 200 μL 1640 culture medium were injected subcutaneously into the right side of each female nude mouse. Tumour volume was calculated as:
When tumour size reached 50–100 mm3, 36 mice were randomised and injected with 150 μL saline (control), doxorubicin (3 mg/kg) via tail vein, and PCBH (150 mg/kg) subcutaneously every 3 days. After 15 days of treatment, the mice were sacrificed and dissected to obtain tissues.
Histological analysis and immunohistochemistry
The tissues were removed and fixed with 4% paraformaldehyde for 24 h. These tissues included the kidney, liver, heart, liver, and spleen. The tissue is then sealed inside a paraffin block and stained with haematoxylin and eosin (H&E). The following immunohistochemical stains were used: CD31, CD34, VEGF, HIF-1α, P-gp, and Ki-67 in accordance with the manufacturer’s recommendations.
Gene expression analysis
Following the manufacturer’s instructions, the total ribonucleic acid (RNA) was separated from the samples (200 mg) by homogenizing them in a Trizol reagent. Using a spectrophotometer to measure absorbance at 260 nm, the amount of RNA in each sample was calculated. An in vitro transcription kit was used to create complementary deoxyribonucleic acid (cDNA), the first strand. SYBR Green/Fluorescein PCR Premix Mix, forward and reverse primers, cDNA, and nuclease-free water made up the reaction mixture.
Primer sequences of the genes were as follows:
HIF-1α F: 5΄-GATTCAAGTGGTCTTCCTGCTTCAGC-3′΄
R: 5΄-GGGACTCATCCCAGGCGGG-3′΄
P-gp F: 5′-CCCTACATTGCAATAGCAGG-3′
R: 5′-GTTCAAACTTCTGCTCCTGA-3′
The gene of interest was normalized against the reference geneglyceraldehyde-3-phosphate dehydrogenase (GAPDH):
F: 5′-ACGGCACAGTCAAGGCCGAG-3′
R: 5′-ACCCTTCAAGTGGGCCCCGG-3′
Initial denaturation was performed at 50 °C for 2 min, followed by 10 min at 95 °C, 40 cycles of denaturation at 95 °C for 15 s, annealing at 55 °C for 30 s, and a melt curve of 30 s at 72 °C. PCR was carried out thrice. The expression level of each target gene was calculated as 2-△Ct.
Measurement of oxygen content
O2 sensor foils with a 6 mm size were slid against the tissue surface to remove air, and measurements were taken after waiting at least 2 min for the sensor and tumour surface to reach a stable state. The VisiSens imaging systems (PreSens, Regensburg Germany) were used to show Luminescence 2D imaging. Before measuring O2, three tumour-bearing mice in each group received subcutaneous injections of 150 μL saline (control) and 150 mg/kg PCBH. The mean values in each area of interest were determined after the image had been defined (ROI). The VisiSens Analytical Software (PreSens, Regensburg, Germany) analyses the data that is recorded and evaluated [Citation35].
Statistical analysis
All the data are evaluated as mean ± standard deviation (SD) based on at least three tests. ANOVA test was used to analyze the statistical discrepancy. Kruskal–Wallis one-way analysis was used when heterogeneity of variance. p-Values <0.05 were considered to indicate a statistically significant result.
Results and discussion
Oxygen-releasing capacity
HBOCs’ principal purpose is to deliver oxygen (O2) to tissues by binding gases to haemoglobin (Hb). The alterations in P50 that OECs show is what defines Hb-O2 affinity. A right shift of the OEC indicated a decrease in the affinity of Hb and O2 which demonstrated a subsequent increase in P50 [Citation34]. As shown in , there was a striking left shift in the OEC of PCBH compared to bHb, indicating that PCBH had a much stronger Hb-O2 affinity than bHb in buffer solution (pH = 7.4). The PCBH and bHb have P50 values () of 20.20 ± 1.03 mmHg and 27.81 ± 0.46 mmHg, respectively.
Figure 1. The Oxygen-releasing Capacity. (A) Oxygen equilibriumcurves (OEC) of bHb and PCBH. Data are expressed as mean ± SD (n = 3). (B) The P50 values of bHb and PCBH. Data are expressed as mean ± SD (n = 3). (C) The trend of oxyHb% of bHb and PCBH in hypoxic atmosphere (1% O2) for two hours. Data are expressed as mean ± SD (n = 3). (D) The trend of oxyHb% of bHb and PCBH in cyclically reduced atmospheric oxygen levels for 5h. Data are expressed as mean ± SD (n = 3). ** represented p < 0.01.
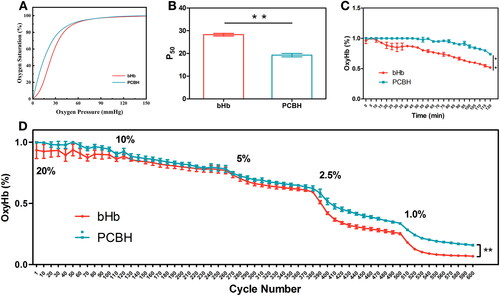
The higher Hb-O2 affinity of PCBH compared with bHb was further confirmed in the ODA, where oxygenated PCBH and bHb were deoxygenated in a hypoxia atmosphere (1% O2) for 2 h and the percentage of oxyHb (oxyHb%) were quantitated. After 2 h, oxyHb in bHb decreased from 100% to 51% (). However, 73% of Hb in PCBH remained in the oxygenated state which is significantly higher than bHb (p < 0.01). The atmosphere’s oxygen in the chamber of plate reader was reduced by 1/2 every hour to compare the oxygen-releasing capacities of bHb and PCBH, and the measurements were lasted for 6 h. As shown in , the substantial difference in their oxyHb% began to emerge at 5% oxygen saturation in the air of chamber, and even then, PCBH's oxyHb% has been significantly higher than bHb’s (p < 0.01). According to these findings, PCBH has a stronger O2 affinity than bHb and postpones O2 release in a hypoxic environment.
PEGylation of Hb was created to attach PEGs of different sizes to Hb, typically via amino groups or cysteine residues, and the modification of Hb’s Cys-93(β) affects Hb’s oxygen-binding characteristics [Citation36–38]. Human Hb’s P50 was reduced by PEGylation from 14 mmHg to 7 mmHg, as demonstrated by Hu et al. [Citation37]. Because of the four-amino groups of Hb being reductively alkylated, the lengthy PEG chains attached to the amino termini, or a combination of the two, the PEG-Hb exhibits significant oxygen-affinity [Citation38].
Evaluation of cell survival of 4T1 or MCF-7ADR after chemotherapy
Both MCF-7ADR and 4T1 cells were grown in normoxic or hypoxic (1% O2) air. Both bHb and PCBH demonstrated the ability to increase the cytotoxicity of DOX after 6h and 12h. As illustrated in , free DOX showed similarly minimal toxicity in MCF-7ADR cells and 4T1 cells in a hypoxic environment in 6h and 12h incubation, but the cytotoxicity of DOX was significantly improved in a normoxic environment. As showed in , the cell viability of free DOX in a normoxic environment was significantly higher than that of DOX combined with bHb or PCBH in high concentration (Hb: 30, 60 μg/well) in 6h and 12h incubation in MCF-7ADR cells, and in 6h incubation in 4T1 cells. It was demonstrated that DOX's oxygen-enhanced toxicity was dose-dependent. These findings demonstrated the cells’ decreased sensitivity to DOX's chemo-damage under hypoxia.
Figure 2. Cell survivals of 4T1 or MCF-7ADR after chemotherapy. Viability of MCF-7ADR cells after treatment with free DOX, bHb-DOX, or PCBH-DOX at different concentrations (1 μg/mL, 2 μg/mL, 4 μg/mL, 8 μg/mL) for 6h (A), (B) and 12h (C), (D) in hypoxia or normoxia. Data are expressed as mean ± SD (n = 3). Viability of 4T1 cells after treatment with free DOX, bHb-DOX, or PCBH-DOX at different concentrations (1 μg/mL, 2 μg/mL, 4 μg/mL, 8 μg/mL) for 6h (E), (F) and 12h (G), (H) in hypoxia or normoxia. Data are expressed as mean ± SD (n = 3). (I) Fluorescence images of γ-H2AX generation in 4T1 cells after treatment with different combination, scale bar = 50 μm. (J) The quantitative analysis of average fluorescent intensity of γ-H2AX in 4T1 cells. Data are expressed as mean ± SD (n = 3). * represented p < 0.05; ** represented p < 0.01.
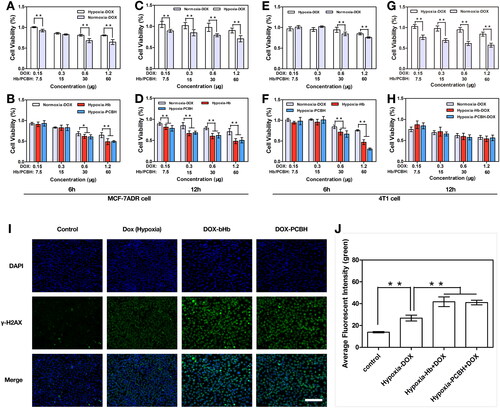
The DNA damage can comprise base damage, sugar damage, single-stranded breaks, or double-stranded breaks (DSBs), the latter being vital to cells. These fatal breaks in the DNA allow the prediction of toxicity in cells, and the γ-H2AX assay is a particularly precise method for detecting DSBs [Citation39]. Topoisomerase I and II inhibitors, particularly cytotoxic pharmaceuticals that produce DNA DSBs, are among the medications currently on the market that have been associated with the creation of γ-H2AX [Citation40]. By blocking topoisomerase I and II and intercalating into DNA to influence its uncoiling, DOX can directly damage DNA by preventing tumour cells or normal cells from proliferating further, ultimately leading to programmed cell death [Citation41]. To assess the DNA damage caused by free DOX and DOX coupled with bHb or PCBH in 4T1 cells under hypoxia, the γ-H2AX assay was used (). Compared to free DOX, DOX in combination with bHb or PCBH significantly increased DNA damage (p < 0.01) under hypoxia. These findings revealed that the oxygen supply provided by bHb or PCBH can increase the toxicity of DOX against tumours or increase the sensitivity of hypoxic tumour cells to DOX.
In vivo antitumor study
To investigate how DOX coupled with PCBH enhanced the anticancer impact, a mouse model of the 4T1 tumour was created. The tumours () extracted on day 14 following the initial therapy and the matching tumour weights served as evidence of the therapeutic response (). Every day, changes in each mouse’s tumour volume were tracked (). The group treated with PCBH alone show the same performance in terms of tumour volume and weights as the blank control group with NS administration, which demonstrated no anticancer activity. The effect of the DOX injection involves the suppression of tumour growth. In contrast, when DOX and PCBH were administered to tumour-bearing mice, a larger anticancer impact was observed. Following treatment, the tumours were stained with haematoxylin and eosin (H&E) and the necrosis rate of the tumours was analyzed using Image J (). When compared with the blank control treated with NS, a large number of cells within tumour sections underwent structural deformation and showed signs of apoptosis in and around the necrotic foci in both the free DOX and DOX combined with PCBH treated groups. In comparison, the latter scenario more clearly demonstrated the effects of cell destruction, such as the substantially higher degree of cell differentiation, the extensive necrosis of tumour cells, the evident tumour pyknosis, and the significantly reduced blood supply. Several different forms of cancer are graded using the clinically significant proliferation marker Ki-67, which is abundantly expressed in cycling cells [Citation42]. The Level of Ki-67 in the DOX mixed with the PCBH group displayed a noticeably lower production than that of the other three groups, as seen in . These outcomes supported the hypothesis that PCBH could increase the anticancer activity of doxorubicin.
Figure 3. In vivo antitumor efficiency. (A) The picture of tumors excised frommice after 14 days of treatment in different groups. (B) Tumor growth curves measured for 14 days and (C) tumor weight after different treatments. Data are expressed as mean ± SD (n = 7) (D) Hematoxylin and eosin (H&E)-stained tumor slices after treatment in each group at day 14 and (E) necrosis rate of tumors in each group were obtained and analysed by two blinded pathologists under microscope. Data are expressed as mean ± SD (n = 7) (F) Ki-67 immunohistochemical staining images on day 14, scale bar = 50 μm. (G) Quantitative analysis of the relative density of Ki-67 in tumors after treatments. Data are expressed as mean ± SD (n = 7). * represented p < 0.05; ** represented p < 0.01.
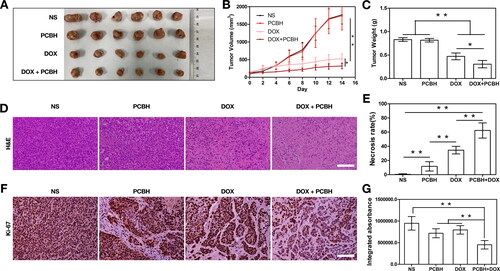
Based on the in vitro data, it is anticipated that PCBH could increase the DOX's effectiveness in eliminating tumours with severe hypoxia. The well-known primary bio-barrier for chemotherapy—the hypoxic tumour microenvironment (TME)—could upregulate HIF-1α in tumour cells, which is well-reported to cause the chemotherapeutic drugs’ induction of multidrug resistance (MDR) and is frequently used to confirm the hypoxia in tumours [Citation43]. To determine the reduction in tumour hypoxia by using PCBH's capacity for O2 delivery and transport, the changes in fluorescent intensity of O2 contained in tumours were measured in two distinct groups, NS (150 μl) and PCBH (150 mg/kg, 150 μl) using the VISISENS fluorescence imaging system. Further calculations of oxygen saturation were made throughout time. As demonstrated in , when NS was administered to a blank control, the oxygen saturation showed a brief increase; the peak occurred at 2 h, and it quickly returned to baseline. It was clear that oxygen saturation increased significantly and remained elevated from 2 h to 8 h, with a minor reduction. It is confirmed that PCBH could significantly and permanently reduce tumour hypoxia while raising the oxygen saturation of tumour tissue.
Figure 4. The alleviation of tumor hypoxia. (A) Oxygen content changes of the tumors with different treatments from 0 h to 8 h. (B) Oxygenation values of tumors from 0 h to 8 h. Error bars represent mean ± SD (n=4). (C) HIF-1α and (F) P-gp immuno histochemical staining images on day 14, scale bar = 50 μm. Quantitative analysis of the relative density of (D) HIF-1α and (G) P-gp from immuno histochemically stained tumor section after treatments, and qRT-PCR validation results of (E) HIF-1α and (H) Pgp after treatments. Data are expressed as mean ± SD (n = 7). * represented p < 0.05; ** represented p < 0.01.
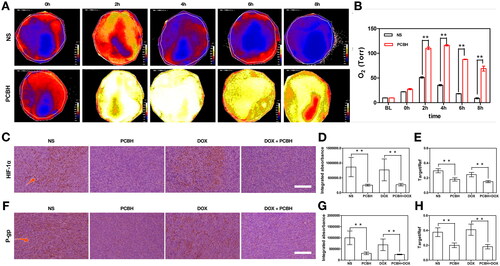
Then, immunohistochemical (IHC) staining analysis was carried out to assess the expression of HIF-1α in tumours under various treatments to determine the contribution of Hb still present in PCBH to the anticancer performance of DOX under hypoxia in vivo (). For the blank control with NS administration and DOX group with free DOX administration, the expression of HIF-1α presented a significantly higher level than that of the groups containing PCBH. The results were reconfirmed by the real-time quantitative PCR (qRT-PCR) of HIF-1α (), indicating that PCBH affected the tumorous hypoxia significantly. In agreement with the results of the previous study [Citation44], the injection of HBOCs could ameliorate the hypoxia in tumours and inhibit the expression of HIF-1α. It is widely known that the presence of hypoxia in tumours causes the MDR efflux pumps, such as P-glycoprotein (P-gp), which is a downstream target gene for HIF-1, to be increased. This has been found to have a significant role in the failure of clinical treatment and patient death [Citation45–47]. In another study, F. Rosso et al. using a murine xenograft showed that cross-linked hyaluronic acid nanoparticles can accumulate into the tumour cells by EPR effect and the CD44 receptor [Citation48]. PCBH might also accumulate into the tumour site through EPR effect to release O2 and inhibit the tumour hypoxia for a long period. demonstrated that consistent with the findings of qRT-PCR, the level of P-gp in the PCBH and DOX-PCBH groups had significantly lower production than that of the NS and free DOX groups (p < 0.01).
New blood vessel formation (angiogenesis) is a fundamental event in the process of tumour growth and metastasis [Citation49]. However, abnormal vasculature is a hallmark of the tumour, especially adjacent to the tumour. Numerous tumour vessels boost the risk that tumour cells will enter systemic circulation [Citation50]. Pathologic angiogenesis is greatly aided by vascular endothelial growth factor (VEGF) and its receptors (VEGFR). Its overexpression has been connected to the development of tumours and a poor prognosis [Citation51,Citation52]. Some studies proposed that certain VEGF ligands promote tumour growth by acting directly through VEGFRs expressed by tumour cells as well as by causing angiogenesis [Citation53–55]. HIF-1α can be increased as a tumour grows, which encourages the synthesis of VEGF through the HIF-1α signaling pathway, which in turn increases the formation of blood vessels. However, because of the ineffective increased blood circulation, the deformed neovascular tissue is unable to rescue the oxygen supply. In numerous tumour systems, hypoxia has been recognized as a key regulator of VEGF expression. As a result of binding to the VEGF promoter in the nucleus under hypoxic conditions, the increased HIF-1α increases VEGF production. Other angiogenic markers, such as CD34 and CD31, which are employed as endothelial cell markers in tumour vessels to analyze microvessel density connected with prognosis in diverse malignancies, have also been researched in relation to cancer prognosis [Citation56]. Several studies demonstrated a clear relation between CD31 and prognosis [Citation10,Citation51,Citation52,Citation57,Citation58], which is a higher CD31 expression in tumours associated with a worse prognosis [Citation59,Citation60].
To investigate the tumorous angiogenesis and prognosis of tumour-bearing mice, the IHC staining analysis was carried out to assess the expression of VEGF, CD31 and CD34 in tumours under various treatments. The expression of VEGF, CD31 and CD34 was lower in the DOX-PCBH group than in the other three groups, as shown in , and those indices were also lower in the DOX group than in the NS and PCBH groups. Moreover, PCBH could downregulate the VEGF, CD31, and CD34 compared with the blank control. It is possible owing to the cytotoxicity of DOX which destroys the vasculature inducing apoptosis and necrosis of tumours, and the angiogenesis of tumours was further inhibited because of the anesis of hypoxia in the tumour microenvironment induced by the PCBH.
Figure 5. The angiogenesis of tumors with different treatments. (A) VEGF, CD31 and CD34 immunohistochemical staining image s on day 14, scale bar = 50 μm. Quantitative analysis of the relative density of (B) VEGF, (C) CD31 and (D) CD34 from immuno histochemically stained tumor section after treatments. Data are expressed as mean ± SD (n = 7), ** represented p < 0.01.
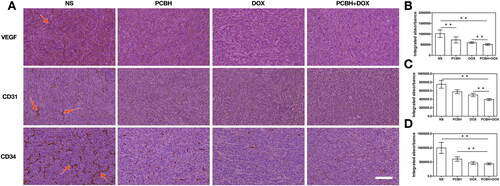
The ability to alleviate DOX-induced splenocardiac toxicity
DOX can bind to a variety of molecular targets, including topoisomerase enzymes I and II, causing DNA damage that activated the apoptosis pathways [Citation61] and intercalating the base pairs of both nuclear and mitochondrial DNA to prevent tumorous or normal cells from reproducing DNA and transcribing RNA [Citation62,Citation63]. Additional activities include the creation of free radicals, which increases DNA damage [Citation23], the suppression of the synthesis of macromolecules [Citation64], the separation or unwinding of DNA, and an increase in alkylation. DOX has demonstrated a strong anticancer potential, but it was constrained by the body’s toxicity to non-tumour cells [Citation41]. The most serious adverse impact brought on by DOX is cardiotoxicity [Citation22,Citation24,Citation65].
To evaluate the impact induced by the tumour and DOX upon major organs, the H&E staining assay and histopathological analyses of the major organ were carried out following the treatments (). For the blank control and free DOX groups (), partial myocardial tissue damage (myocardial cell necrosis) was found. There was interstitial non-specific inflammation and myocardial cell edoema and degeneration at the lesion site. The muscle space at the lesion site was widened, and fibrous tissue hyperplasia was found at the lesion site. The central ventricular wall and papillary muscles were both affected by the comparatively confined lesions. When the mice were given PCBH compared to the two groups above, there was modest interstitial inflammation, however, there was no cardiac tissue injury, local myocardial cell edoema, or local muscle space widening at the lesion site. also revealed an intriguing finding: while in PCBH groups, the structure of red and white pulp in splenic tissue was clear, lymphocyte density was normal, and the number and density of lymphocytes in splenic cord were not decreased, DOX could induce contraction of white pulp in the spleen and significantly reduce the density and number of lymphocytes.
Figure 6. The ability to alleviate DOX-induced splenocardiac toxicity. (A) H&E staining image of hearts and spleens (white pulp) dissected fromtumor-bearing mice after 14 days treatments, scale bar = 50 μm. The statistical analysis of myocardial injury (B) and relative atrophy of white pulp (C) obtained by two blinded pathologists under microscope. The necrotic myocardial cells and white pulp were pointed by arrows. Data are expressed as mean ± SD (n = 7), ** represented p < 0.01.
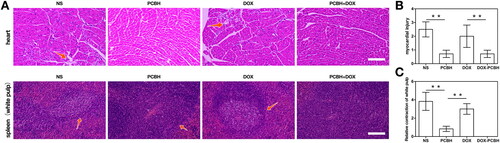
The present results indicated that DOX-induced cardiac toxicity could be ascribed to histological alteration in cardiac tissue, which is surfacing in fibrosis and apoptosis. The DOX-induced cardiotoxicity may involve diverse signaling mechanisms including apoptosis, oxidative stress, calcium overloading, and mitochondrial dysfunction [Citation66–69]. The incidence of DOX-induced cardiac toxicity increases with the elevation in the total accumulation of DOX during the treatment cycle [Citation25,Citation28]. The heart tissue is regarded as the target tissue for DOX because of the cardiolipin contained in myocardium, which is thought to have affinity to DOX, leading to the DOX accumulation in the myocytic mitochondria [Citation70–72]. Furthermore, our histological analysis showed that DOX decreased leukocytes and macrophages in the spleen. White pulp contraction caused by DOX, lymphocyte and macrophage depletion, and red and white pulp deformation were signs of host immunosuppression and DOX-toxicity damage to the defence system. According to the aforementioned findings, PCBH may be able to mitigate the cardiotoxicity and splenic hypoplasia induced by both tumours and DOX.
The spleen functions as a reservoir for leukocytes and initiates immune response against injury, as well as regulates adaptive immune response [Citation73]. The immune defense and cardiac healing are defined by the splenic leukocyte reservoir [Citation74,Citation75]. Through the use of a non-cancer model, Jeevan et al. discovered unique DOX-induced malignant effects that created a thorough mechanism in the heart and spleen [Citation21]. In other words, the DOX-mediated heart damage was also accompanied by splenic mass contraction, leukocyte and macrophage depletion, and germinal centre compaction. Numerous studies have shown that disruption of the immune system’s metabolism characterizes the cardiac toxicity of DOX [Citation21]. Immune-sensitive enzymes including cyclooxygenase (COX) and lipoxygenases (LOX) are the principal drivers of immunometabolism. The spleen’s increased essential fatty acids are necessary for the manufacture of COXs and LOXs [Citation76–80]. To impede immunometabolism, DOX may cause dysregulation of LOXs and COXs in the spleen, toxicate macrophages in the splenic marginal zone, and inhibit leukocyte immunological function [Citation21]. In conclusion, after long-term exposure to DOX, DOX can induce dysregulation of immune-sensitive enzymes in heart and spleen cells, and then affect the host immune metabolism, thus further developing into irreversible splenocardiac cachexia.
In the current report, we ratiocinated that PCBH inhibited HIF-1 expression in tumour tissues by improving hypoxia in tumour tissues, and then inhibited DOX efflux mediated by P-glycoprotein, which allowed a large amount of DOX to be retained in tumour tissues and indirectly reduced DOX exposure in primary organs such as heart and spleen. Thus, PCBH treatment can reduce the effects of DOX-induced splenocardiac cachexia with impaired immunometabolism in cardiotoxicity while also improving the chemotherapeutic effectiveness of DOX. Because of the alleviated hypoxia in tumour tissues in the PCBH groups, our IHC study showed that P-gp was greatly reduced. This resulted from suppressed DOX efflux and much more DOX being limited to tumour tissues. The γ-H2AX assay in vitro investigation showed that more DOX was absorbed by cells to damage DNA as opposed to being excreted back into the extracellular environment.
Conclusion
In summary, this work investigated the competence of PCBH to improve the hypoxia in breast tumours and enhance the efficiency of the chemotherapeutic agent DOX, as well as study the capacity of PCBH to ameliorate DOX-induced splenocardiac cachexia. Here, we describe and demonstrate how PCBH inhibited HIF-1 expression in tumour tissues by enhancing hypoxia in tumour tissues. This was followed by an inhibition of DOX efflux mediated by P-glycoprotein, allowing a significant amount of DOX to be retained in tumour tissues and indirectly reducing DOX exposure in primary organs like the heart and spleen.
Author contributions
Conception of the idea: Bingting Li, Quan Wang; design of the work: Bingting Li, Quan Wang, Hong Zhou; acquisition, analysis and interpretation of data: Bingting Li, Jun Zhang, Ning Ma, Weidan Li, Guoxing You, Gan Chen, Lian Zhao; drafting the manuscript: Bingting Li; revising the manuscript: Bingting Li, Quan Wang, Gan Chen, Lian Zhao, Hong Zhou, Guoxing You; supervision: Quan Wang, Gan Chen, Lian Zhao, Hong Zhou; final approval of the manuscript: all; agreement to be accountable for all aspects of the work: all.
Acknowledgements
The authors acknowledge support from Zhong Bao Mu biotechnology Co. Ltd.
Disclosure statement
No potential conflict of interest was reported by the author(s).
Data availability statement
The data that support the findings of this study are available from the corresponding author QW upon reasonable request.
Additional information
Funding
References
- Rivankar S. An overview of doxorubicin formulations in cancer therapy. J Cancer Res Ther. 2014;10(4):853–858.
- Tacar O, Dass CR. Doxorubicin-induced death in tumour cells and cardiomyocytes: is autophagy the key to improving future clinical outcomes? J Pharm Pharmacol. 2013;65(11):1577–1589.
- Cardano M, Tribioli C, Prosperi E. Targeting proliferating cell nuclear antigen (PCNA) as an effective strategy to inhibit tumor cell proliferation. Curr Cancer Drug Targets. 2020;20(4):240–252.
- Plate KH, Scholz A, Dumont DJ. Tumor angiogenesis and anti-angiogenic therapy in malignant gliomas revisited. Acta Neuropathol. 2012;124(6):763–775.
- Wei X, Chen Y, Jiang X, et al. Mechanisms of vasculogenic mimicry in hypoxic tumor microenvironments. Mol Cancer. 2021;20(1):7.
- Jing X, Yang F, Shao C, et al. Role of hypoxia in cancer therapy by regulating the tumor microenvironment. Mol Cancer. 2019;18(1):157.
- Li Y, Zhao L, Li XF. Hypoxia and the tumor microenvironment. Technol Cancer Res Treat. 2021;20:15330338211036304.
- Graham K, Unger E. Overcoming tumor hypoxia as a barrier to radiotherapy, chemotherapy and immunotherapy in cancer treatment. Int J Nanomedicine. 2018;13:6049–6058.
- Vaupel P, Mayer A. Hypoxia in cancer: significance and impact on clinical outcome. Cancer Metastasis Rev. 2007;26(2):225–239.
- Evans SM, Koch CJ. Prognostic significance of tumor oxygenation in humans. Cancer Lett. 2003;195(1):1–16.
- Höckel M, Vaupel P. Tumor hypoxia: definitions and current clinical, biologic, and molecular aspects. J Natl Cancer Inst. 2001;93(4):266–276.
- Wu BB, Leung KT, Poon EN. Mitochondrial-Targeted therapy for doxorubicin-induced cardiotoxicity. Int J Mol Sci. 2022;23(3):1912. doi: 10.3390/ijms23031912. PMID: 35163838; PMCID: PMC8837080.
- Shafei A, El-Bakly W, Sobhy A, et al. A review on the efficacy and toxicity of different doxorubicin nanoparticles for targeted therapy in metastatic breast cancer. Biomed Pharmacother. 2017;95:1209–1218.
- Poljaková J, Eckschlager T, Hřebačková J, et al. The comparison of cytotoxicity of the anticancer drugs doxorubicin and ellipticine to human neuroblastoma cells. Interdiscip Toxicol. 2008;1(2):186–189.
- Baxter-Holland M, Dass CR. Doxorubicin, mesenchymal stem cell toxicity and antitumour activity: implications for clinical use. J Pharm Pharmacol. 2018;70(3):320–327.
- Bao MH, Wong CC. Hypoxia, metabolic reprogramming, and drug resistance in liver cancer. Cells. 2021;10(7):1715.
- Jansman MMT, Liu X, Kempen P, et al. Hemoglobin-Based oxygen carriers incorporating nanozymes for the depletion of reactive oxygen species. ACS Appl Mater Interfaces. 2020;12(45):50275–50286.
- Dai M, Yu M, Han J, et al. PEG-conjugated hemoglobin combination with cisplatin enforced the antiangiogeic effect in a cervical tumor xenograft model. Artif Cells Blood Substit Immob Biotechnol. 2008;36(6):487–497.
- Teicher BA. Hypoxia and drug resistance. Cancer Metastasis Rev. 1994;13(2):139–168.
- Lucas A, Belcher DA, Munoz C, et al. Polymerized human hemoglobin increases the effectiveness of cisplatin-based chemotherapy in non-small cell lung cancer. Oncotarget. 2020;11(42):3770–3781.
- Jadapalli JK, Wright GW, Kain V, et al. Doxorubicin triggers splenic contraction and irreversible dysregulation of COX and LOX that alters the inflammation-resolution program in the myocardium. Am J Physiol Heart Circulat Physiol. 2018;315(5):h1091–h1100.
- Songbo M, Lang H, Xinyong C, et al. Oxidative stress injury in doxorubicin-induced cardiotoxicity. Toxicol Lett. 2019;307:41–48.
- Bhagat A, Kleinerman ES. Anthracycline-Induced cardiotoxicity: causes, mechanisms, and prevention. Adv Exp Med Biol. 2020;1257:181–192.
- Rawat PS, Jaiswal A, Khurana A, et al. Doxorubicin-induced cardiotoxicity: an update on the molecular mechanism and novel therapeutic strategies for effective management. Biomed Pharmacother. 2021;139:111708.
- Sheibani M, Azizi Y, Shayan M, et al. Doxorubicin-Induced cardiotoxicity: an overview on pre-clinical therapeutic approaches. Cardiovasc Toxicol. 2022;22(4):292–310.
- Von Hoff DD, Layard MW, Basa P, Jr., et al. Risk factors for doxorubicin-induced congestive heart failure. Ann Intern Med. 1979;91(5):710–717.
- Ganz PA, Hussey MA, Moinpour CM, et al. Late cardiac effects of adjuvant chemotherapy in breast cancer survivors treated on southwest oncology group protocol s8897. J Clin Oncol. 2008;26(8):1223–1230.
- Avagimyan A, Mkrtchyan L, Abrahomovich O, et al. AC-Mode of chemotherapy as a trigger of cardiac syndrome X: a case study. Curr Probl Cardiol. 2022;47(9):100994.
- Ludke AR, Al-Shudiefat AA, Dhingra S, et al. A concise description of cardioprotective strategies in doxorubicin-induced cardiotoxicity. Can J Physiol Pharmacol. 2009;87(10):756–763.
- Abdulkareem Aljumaily SA, Demir M, Elbe H, et al. Antioxidant, anti-inflammatory, and anti-apoptotic effects of crocin against doxorubicin-induced myocardial toxicity in rats. Environ Sci Pollut Res Int. 2021;28(46):65802–65813.
- Traverso N, Ricciarelli R, Nitti M, et al. Role of glutathione in cancer progression and chemoresistance. Oxid Med Cell Longev. 2013;2013:972913.
- Xie G, Dong H, Liang Y, et al. CAR-NK cells: a promising cellular immunotherapy for cancer. EBioMedicine. 2020;59:102975.
- Chu Z, Wang Y, You G, et al. The P(50) value detected by the oxygenation-dissociation analyser and blood gas analyser. Artif Cells Nanomed Biotechnol. 2020;48(1):867–874.
- Oksenberg D, Dufu K, Patel MP, et al. GBT440 increases haemoglobin oxygen affinity, reduces sickling and prolongs RBC half-life in a murine model of sickle cell disease. Br J Haematol. 2016;175(1):141–153.
- Auerswald S, Schreml S, Meier R, et al. Wound monitoring of pH and oxygen in patients after radiation therapy. Radiat Oncol. 2019;14(1):199.
- Webster KD, Dahhan D, Otto AM, et al. “Inside-out" PEGylation of bovine β-cross-linked hemoglobin. Artif Organs. 2017;41(4):351–358.
- Wang Y, Wang L, Yu W, et al. A PEGylated bovine hemoglobin as a potent hemoglobin-based oxygen carrier. Biotechnol Prog. 2017;33(1):252–260.
- Hu T, Prabhakaran M, Acharya SA, et al. Influence of the chemistry of conjugation of poly(ethylene glycol) to Hb on the oxygen-binding and solution properties of the PEG-Hb conjugate. Biochem J. 2005;392(Pt 3):555–564.
- Kuo LJ, Yang LX. Gamma-H2AX - a novel biomarker for DNA double-strand breaks. In Vivo. 2008;22(3):305–309.
- Huang X, Traganos F, Darzynkiewicz Z. DNA damage induced by DNA topoisomerase I- and topoisomerase II-inhibitors detected by histone H2AX phosphorylation in relation to the cell cycle phase and apoptosis. Cell Cycle. 2003;2(6):613–618.
- Tacar O, Sriamornsak P, Dass CR. Doxorubicin: an update on anticancer molecular action, toxicity and novel drug delivery systems. J Pharm Pharmacol. 2013;65(2):157–170.
- Sun X, Kaufman PD. Ki-67: more than a proliferation marker. Chromosoma. 2018;127(2):175–186.
- Iyer AK, Singh A, Ganta S, et al. Role of integrated cancer nanomedicine in overcoming drug resistance. Adv Drug Deliv Rev. 2013;65(13–14):1784–1802.
- Wang P, Li X, Yao C, et al. Orthogonal near-infrared upconversion co-regulated site-specific O(2) delivery and photodynamic therapy for hypoxia tumor by using red blood cell microcarriers. Biomaterials. 2017;125:90–100.
- Yoh K, Ishii G, Yokose T, et al. Breast cancer resistance protein impacts clinical outcome in platinum-based chemotherapy for advanced non-small cell lung cancer. Clin Cancer Res. 2004;10(5):1691–1697.
- Baekelandt MM, Holm R, Nesland JM, et al. P-glycoprotein expression is a marker for chemotherapy resistance and prognosis in advanced ovarian cancer. Anticancer Res. 2000;20(2B):1061–1067.
- Lin JH, Yamazaki M. Role of P-glycoprotein in pharmacokinetics: clinical implications. Clin Pharmacokinet. 2003;42(1):59–98.
- Rosso F, Quagliariello V, Tortora C, et al. Cross-linked hyaluronic acid Sub-micron particles: in vitro and in vivo biodistribution study in cancer xenograft model, journal of materials science. J Mater Sci Mater Med. 2013;24(6):1473–1481.
- Hicklin DJ, Ellis LM. Role of the vascular endothelial growth factor pathway in tumor growth and angiogenesis. J Clin Oncol. 2005;23(5):1011–1027.
- Matsuda Y, Hagio M, Ishiwata T. Nestin: a novel angiogenesis marker and possible target for tumor angiogenesis. World J Gastroenterol. 2013;19(1):42–48.
- Lee JC, Chow NH, Wang ST, et al. Prognostic value of vascular endothelial growth factor expression in colorectal cancer patients. European Journal of Cancer. 2000;36(6):748–753.
- Maeda K, Chung YS, Ogawa Y, et al. Prognostic value of vascular endothelial growth factor expression in gastric carcinoma. Cancer. 1996;77(5):858–863.
- Wehland M, Bauer J, Magnusson NE, et al. Biomarkers for anti-angiogenic therapy in cancer. Int J Mol Sci. 2013;14(5):9338–9364.
- Woollard DJ, Opeskin K, Coso S, et al. Differential expression of VEGF ligands and receptors in prostate cancer. Prostate. 2013;73(6):563–572.
- Ebos JM, Tran J, Master Z, et al. Imatinib mesylate (STI-571) reduces Bcr-Abl-mediated vascular endothelial growth factor secretion in chronic myelogenous leukemia. Mol Cancer Res. 2002;1(2):89–95.
- Hansen S, Grabau DA, Rose C, et al. Angiogenesis in breast cancer: a comparative study of the observer variability of methods for determining microvessel density. Lab Invest. 1998;78(12):1563–1573.
- Basilio-de-Oliveira RP, Pannain VL. Prognostic angiogenic markers (endoglin, VEGF, CD31) and tumor cell proliferation (Ki67) for gastrointestinal stromal tumors. World J Gastroenterol. 2015;21(22):6924–6930.
- Majchrzak K, Kaspera W, Szymaś J, et al. Markers of angiogenesis (CD31, CD34, rCBV) and their prognostic value in low-grade gliomas. Neurol Neurochir Pol. 2013;47(4):325–331.
- Saad RS, El-Gohary Y, Memari E, et al. Endoglin (CD105) and vascular endothelial growth factor as prognostic markers in esophageal adenocarcinoma. Hum Pathol. 2005;36(9):955–961.
- Saad RS, Lindner JL, Liu Y, et al. Lymphatic vessel density as prognostic marker in esophageal adenocarcinoma. Am J Clin Pathol. 2009;131(1):92–98.
- Swain SM, Whaley FS, Ewer MS. Congestive heart failure in patients treated with doxorubicin: a retrospective analysis of three trials. Cancer. 2003;97(11):2869–2879.
- Fornari FA, Randolph JK, Yalowich JC, et al. Interference by doxorubicin with DNA unwinding in MCF-7 breast tumor cells. Mol Pharmacol. 1994;45(4):649–656.
- Tardi PG, Boman NL, Cullis PR. Liposomal doxorubicin. J Drug Target. 1996;4(3):129–140.
- Seymour L, Bramwell V, Moran LA. Use of dexrazoxane as a cardioprotectant in patients receiving doxorubicin or epirubicin chemotherapy for the treatment of cancer. The provincial systemic treatment disease site group. Cancer Prev Control. 1999;3(2):145–159.
- Christidi E, Brunham LR. Regulated cell death pathways in doxorubicin-induced cardiotoxicity. Cell Death Dis. 2021;12(4):339.
- Gholami S, Hosseini MJ, Jafari L, et al. Mitochondria as a target for the cardioprotective effects of Cydonia oblonga mill. and Ficus carica L. in doxorubicin-Induced cardiotoxicity. Drug Res. 2017;67(6):358–365.
- Varga ZV, Ferdinandy P, Liaudet L, et al. Drug-induced mitochondrial dysfunction and cardiotoxicity, American journal of physiology. Am J Physiol Heart Circ Physiol. 2015;309(9):H1453–1467.
- Szenczi O, Kemecsei P, Holthuijsen MF, et al. Poly(ADP-ribose) polymerase regulates myocardial calcium handling in doxorubicin-induced heart failure. Biochem Pharmacol. 2005;69(5):725–732.
- Minotti G, Recalcati S, Menna P, et al. Doxorubicin cardiotoxicity and the control of iron metabolism: quinone-dependent and independent mechanisms. Methods Enzymol. 2004;378:340–361.
- De Beer EL, Bottone AE, Voest EE. Doxorubicin and mechanical performance of cardiac trabeculae after acute and chronic treatment: a review. Eur J Pharmacol. 2001;415(1):1–11.
- Ascensão A, Magalhães J, Soares JM, et al. Moderate endurance training prevents doxorubicin-induced in vivo mitochondriopathy and reduces the development of cardiac apoptosis. Am J Physiol Heart Circ Physiol. 2005;289(2):H722–731.
- Sun Z, Yan B, Yu WY, et al. Vitexin attenuates acute doxorubicin cardiotoxicity in rats via the suppression of oxidative stress, inflammation and apoptosis and the activation of FOXO3a. Exp Ther Med. 2016;12(3):1879–1884.
- Cesta MF. Normal structure, function, and histology of the spleen. Toxicol Pathol. 2006;34(5):455–465.
- Halade GV, Norris PC, Kain V, et al. Splenic leukocytes define the resolution of inflammation in heart failure. Sci Signaling. 2018;11(520):eaao1818. doi: 10.1126/scisignal.aao1818. PMID: 29511119; PMCID: PMC6145858.
- Perez OA, Yeung ST, Vera-Licona P, et al. CD169(+) macrophages orchestrate innate immune responses by regulating bacterial localization in the spleen. Sci Immunol. 2017;2(16):eaah5520. doi: 10.1126/sciimmunol.aah5520. PMID: 28986418; PMCID: PMC5969998.
- Kalivendi SV, Kotamraju S, Zhao H, et al. Doxorubicin-induced apoptosis is associated with increased transcription of endothelial nitric-oxide synthase. Effect of antiapoptotic antioxidants and calcium. J Biol Chem. 2001;276(50):47266–47276.
- Ding XZ, Hennig R, Adrian TE. Lipoxygenase and cyclooxygenase metabolism: new insights in treatment and chemoprevention of pancreatic cancer. Mol Cancer. 2003;2:10.
- Ahmed F, Urooj A. Cardioprotective activity of standardized extract of ficus racemosa stem bark against doxorubicin-induced toxicity. Pharm Biol. 2012;50(4):468–473.
- Ingle KA, Kain V, Goel M, et al. Cardiomyocyte-specific Bmal1 deletion in mice triggers diastolic dysfunction, extracellular matrix response, and impaired resolution of inflammation. Am J Physiol Heart Circulat Physiol. 2015;309:H1827–1836.
- Ahmad S, Panda BP, Kohli K, et al. Folic acid ameliorates celecoxib cardiotoxicity in a doxorubicin heart failure rat model. Pharm Biol. 2017;55:1295–1303.