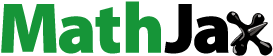
Abstract
As an autoimmune disease of the colon, the incidence of ulcerative colitis (UC) remains high. Carbon dots (CDs), a new type of nanomaterials, display excellent biological activity and are expected to inspire a new treatment for UC. A green method was used to carbonise rhei radix rhizoma (RRR) and extract CDs to study their anti-ulcer activity. The RRR-based carbon dots (RRR-CDs) were characterised by electron microscopy, optical techniques, and other techniques. The results indicated RRR-CDs have abundant chemical groups, excellent solubility and tiny size (1.374 nm–4.533 nm), which may be conducive to the exertion of inherent activity. Using a classic dextran sodium sulphate (DSS)-induced UC mouse model, for the first time, it was demonstrated RRR-CDs have significant anti-ulcerative activity in improving DAI score (from 2.8 to 1.6), colon length (4.15 to 6.08), and histopathology in mice. The underlying mechanisms of anti-ulcerative activity may be related to haemostatic, antioxidant, and anti-inflammatory activities to protect the mucosal barrier. RRR-CDs have symptomatic and potential mechanisms of treatment and are expected to become a candidate drug for the treatment of UC. This not only further expands the basis for the biological activity of CDs, but provides a potential treatment plan for solving thorny diseases in clinical practice.
Graphical Abstract
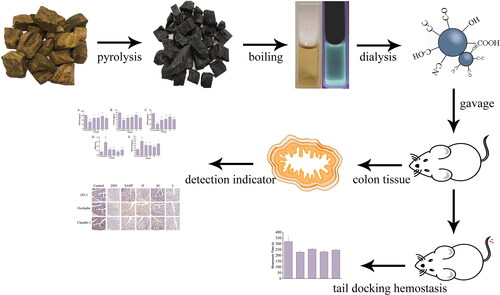
1. Introduction
Ulcerative colitis (UC) was first named by Samuel Wilks in 1859 [Citation1]. It is a chronic, relapsing disease that begins with inflammation of the rectal mucosa and continues to invade the submucosa while extending proximally until it involves all parts of the whole large intestine [Citation2]. According to incomplete statistics, there are currently 8.8–23.1 cases per 100,000 people in North America, 0.6–24.3 cases per 100,000 people in Europe, and China has steadily increased from 1.2 per 100,000 people. With the change in habits and increase in social pressure, the incidence of UC has risen rapidly and gradually evolves into a global intractable disease [Citation3]. Patients often suffer from clinical symptoms such as abdominal pain, pus, and blood in the stool, tenesmus, and weight loss. The pathogenesis of UC is still unclear, but it is often caused by a combination of several factors. Among them, oxidative stress, the inflammatory response, and intestinal mucosal barrier function play an important role in the occurrence and development of UC. Intestinal mucosal barrier function is jointly maintained by important membrane proteins, such as ZOs (zonula occludens), occludins and claudins, and the actin cytoskeleton, and is the first line of defense to protect the intestinal epithelium from harmful substances [Citation4]. However, increased oxidative stress and inflammatory responses can cause alterations in intestinal mucosal permeability by disrupting the structure of tight junctions (TJs) [Citation5]. Therefore, reducing oxidative stress and the inflammatory response and protecting mucosal integrity are of great significance for the treatment of UC.
The treatment of UC is difficult in clinical practice, so the disease is still a tricky problem that makes someone headache. At present, drug therapy is still the mainstream treatment method. Drugs such as aminosalicylic acid, corticosteroids, and immunosuppressants are frequently used clinically. The short-term efficacy of these drugs is acceptable, but the long-term efficacy is unsatisfactory and causes serious side effects, such as thromboembolism and easy recurrence after discontinuation [Citation6]. The state of persistent and refractory disease increases the risk of the inflammation-cancer transition and endangers the suffering of patients. Thus, finding a safe, long-term and effective therapeutic drug has become the common expectation and urgent demand of doctors and patients.
Carbon dots (CDs), known as carbon-based zero-dimensional fluorescent nanomaterials [Citation7,Citation8], have a particle size range of less than 10 nm, and they distribute a variety of organic functional groups on spherical surfaces [Citation9]. CDs are highly praised by analytical researchers for their superior performance, including fluorescent properties, biocompatibility, low toxicity, and ease of functionalization [Citation10–12]. CDs also show unexpected advantages in the field of biomedicine, such as targeted drug delivery, fluorescent probes, artificially simulated enzymes, photosensitizers, and antibacterial molecular nanocarriers [Citation13–18]. In addition, CDs have many biological activities, and these advantages suggest that they have the potential for some incurable diseases. For example, Phellodendri Chinensis Cortex-CDs showed significant anti-psoriasis activity, which was not present in Phellodendri Chinensis Cortex[Citation19].
The Rhei radix et rhizoma (RRR), named Da Huang in Chinese, first appeared in Shen Nong’s Herbal Classic more than 2,000 years ago. RRR with various components, include anthraquinones, possesses diarrhoea effects. However, looking through ancient books and documents, RRR charcoal (named RRRC) was processed to treat diarrhoea, blood in the stool, and other symptoms similar to UC. The previous research of our team [Citation19,Citation20] suggested that CDs in charcoal drugs are the active substances for it to act. This provides us with some scientific clues, that is, RRR-CDs have the effect of treating UC. Therefore, this study adopted a sustainable and green high-temperature pyrolysis method to prepare RRR-CDs, extract their CDs, and study their effect and mechanism in the treatment of UC. Charcoal traditional Chinese medicine (TCM) has a wide range of biological activities. RRR-CDs prepared with RRR as a precursor are an innovative biological source, and the research on the anti-ulcer biological activity of CDs is almost absent at present. This study not only further expands the basis for the biological activity of CDs but also provides a potential treatment plan for solving thorny diseases in clinical practice.
2. Materials and methods
2.1. Chemicals
The RRR material was purchased from Beijing Qiancao Herbal Pieces Co., Ltd. (Beijing, China), and the RRR-CDs were synthesised in our laboratory. Dialysis membranes (molecular weight cut-off was 1000 Da) were purchased from Beijing Ruida Henghui Technology Development Co., Ltd. (Beijing, China). Mouse SOD, MPO, MDA, GSH, and CAT kits were purchased from Nanjing Jiancheng Bioengineering Institute of China (Nanjing, China). Mouse IL-6, IL-10, and TNF-α enzyme-linked immunosorbent assay (ELISA) kits were purchased from Cloud-Clone Crop. (Wuhan, China). The cell counting kit-8 (CCK-8) was purchased from Dojindo Molecular Technologies, Inc. (Kumamoto, Japan). All other chemicals and reagents were of analytical grade. Deionised water was employed throughout this study.
2.2. Animals
All research complied with the Guide for the Care and Use of Laboratory Animals and approved the Ethics Review Committee for Animal Experimentation at the Beijing University of Chinese Medicine. Male BALB/C mice (weighing 22.0 g ± 2.0 g) and male Kunming mice (weighing 30.0 g ± 2.0 g) were obtained from Beijing Vital River Laboratory Animal Technology Co., Ltd (Beijing, China). The animals were housed in a well-ventilated room temperature of 24.0 ± 1.0 °C with 55 – 65% relative humidity under a 12h light/dark cycle. Access to food and water was unlimited.
2.3. Preparation of RRR-CDs
The RRR-CDs were prepared in the muffle furnace (TL0612 muffle furnace; Beijing Zhong Ke Aobo Technology Co., Ltd; Beijing, China) by one-step pyrolysis. First, the RRR samples were placed in separate crucibles and covered with aluminium foil paper with sealed lids. Then, the RRR was carbonised in a preheated muffle furnace at 350 °C for 1h. After cooling to room temperature, the RRR-carbon was ground to a fine powder and boiled twice in a water bath at 100 °C for 1h each time. Thereafter, the decoction solution was filtered (0.22 μm microfiltration membrane), concentrated, and dialysed (1000 Daltons molecular weight cut-off dialysis membrane). The obtained RRR-CDs were stored at 4 °C until further use. The preparation flow chart of RRR-CDs is shown in .
2.4. Characterisation of RRR-CDs
The morphology, particle size distribution, and microstructure information of the RRR-CDs were characterised by transmission electron microscopy (TEM; Tecnai G220, FEI Company, Hillsboro, OR, USA) with an electron energy of 200 kV. Atomic lattice spacing and other internal details were examined using High-resolution transmission electron microscopy (HRTEM) with a JEN-1230 (Japan Electron Optics Laboratory; Japan). Through X-ray photoelectron spectrometer (XPS), the surface composition of the sample was analysed using an ESCALAB 250Xi XPS (Thermo Fisher Scientific, MA, USA) with a monochromatic AI Kα x-ray source. The spectral properties of the CDs were recorded by an ultraviolet (UV-vis) spectrophotometer (CECIL, Cambridge, UK) and a fluorescence spectrophotometer (F-4500, Hitachi, Tokyo, Japan). Additionally, RRR-CDs was characterised using Fourier transform infra-red (FTIR) spectroscopy with an FTIR spectrophotometer (spectral window between 400 and 4,000 cm-1, Thermo Fisher, CA, USA). X-ray diffraction (XRD, D8 Venture Plus X-ray Diffractometer, Bruker AXS, Karlsruhe, Germany) was used to characterise the crystalline RRR-CDs.
2.5. In vitro cytotoxicity
The cytotoxicity of RRR-CDs was detected by CCK-8 assay. RAW 264.7 cells were cultured in DMEM containing 20% foetal bovine serum in a humidified 5% CO2 atmosphere at 37 °C. First, the cells were seeded into 96-well plates at a density of 2 × 105 cells per well and incubated for 12 h. Following this, the original medium was abandoned in each well, and RRR-CDs with different concentrations (1,000 μg/mL, 500 μg/mL, 250 μg/mL, 125 μg/mL, 62.5 μg/mL, 31.25 μg/mL, 15.625 μg/mL) were added for further incubation for 24 h. After 24 h, the cells were washed with phosphate-buffered saline (PBS) to remove all the culture medium in the 96-well plates, and then 10 μL/well CCK-8 solution and 100 μL/well medium was added and incubated for an additional 2 h. Pure DMEM was used as a blank, and the absorbance of each well was detected by a microplate reader (Biotek, VT, USA) at 450 nm wavelength. Untreated cells were used as the control. The cell viability calculation formula is as follows.
Ae, Ab and Ac correspond to the absorbance of the experimental group, blank group and control group, respectively.
2.6. Haemostatic test of RRR-CDs
A mouse tail docking experimental model was established[Citation21,Citation22]. Thirty Kunming mice were randomly divided into 5 groups: control group (normal saline [NS], ip), positive group (hemocoagulase [HC], 0.60 kU/kg−1, ip), and RRR-CDs high-, medium- and low-dose groups (0.23 mg/kg; 0.12 mg/kg; 0.06 mg/kg; ip). Mice were anaesthetised two hours after dosing. We used a sterile scalpel to cut the tail at a distance of 10 mm from the tail tip, immediately placed the tail on filter paper, and used another filter paper to dip the blood in the tail every 30 s until the filter paper had no blood, which was regarded as the stop of bleeding. During this process, the tail bleeding time of each mouse was recorded.
2.7. Model of UC and drug treatment
4% DSS water solution was allowed to be freely drink for 7 days to establish an acute UC model[Citation23,Citation24]. BALB/C male mice (7 weeks old, n = 36) were randomly divided into six groups: the control group (normal saline [NS] 10 ml/kg), model group ([NS] 10 ml/kg), positive group (Sulfasalazine [SASP] 500 mg/kg), high-dose group (RRR-CDs 0.23 mg/kg), medium-dose group (RRR-CDs 0.12 mg/kg) and low-dose group (RRR-CDs 0.06 mg/kg). Only the control group drank pure water freely per day, and all the other groups drank 4% DSS aqueous solution freely.
The mice were sacrificed on the second day after the modelling was completed. Blood and colon tissues were collected to access the macroscopic and histopathological changes.
2.8. Disease activity index
Throughout the study, we observed the weight, activity, mental state, stool characteristics, and mortality of the mice daily and scored the disease activity index according to the weight, stool shape, and occult blood, as shown in [Citation25,Citation26].
Table 1. Disease activity indexTable Footnoteb.
2.9. Histopathological evaluation
After the colon tissue was observed and photographed, part of the colon tissue was quickly fixed in 4% paraformaldehyde solution, dehydrated, embedded in paraffin, and stained with haematoxylin and eosin (H&E). The stained sections were observed and recorded with a microscope at 200x and 400x.
2.10. Detection of relevant biochemical indicators and inflammatory factors in colon tissues
The remaining colon tissue was washed with precooled saline (0.9%), cut into small pieces and homogenised with precolded saline. Then, one part was directly applied to the suspension, and the other part was centrifuged at 3000 rpm for 10 min to obtain the supernatant, which was used to test the level of inflammatory factors (TNF-α, IL-6, IL-10) and oxidative stress (MOP, MDA, SOD, GSH, CAT). All procedures were carried out according to the instructions.
2.11. Detection of distribution of ZO-1, Occludin and Claudin1 in the intestinal epithelium
Paraffin sections of mouse colon tissue were taken, dewaxed, hydrated, antigen retrieved, sealed and then added dropwise with primary antibody at 37 °C. The samples were stored overnight in the refrigerator at 4 °C. The next day, the secondary antibody was added dropwise to incubate and washed with phosphate buffer, and DAB chromogenic solution was added dropwise to develop colour. Nuclei were counterstained with haematoxylin, washed with running water, dehydrated and observed under a microscope for scanning.
2.12. Statistical analysis
The results were analysed by using IBM SPSS Statistics (version 20, SPSS Inc., Chicago, IL, USA). For data with normal distribution and homogeneity of variance, the mean ± standard deviation is provided. One-way analysis of variance (ANOVA) was used to compare differences between groups. Nonnormally distributed data were analysed by nonparametric statistical analysis using the Kruskal–Wallis test. p < 0.05 and p < 0.01 were considered statistically significant differences.
3. Results
3.1. Characterisation of RRR-CDs
TEM and HRTEM images of RRR-CDs obtained at 350 °C for 1h were used to analyse the morphology and particle size distribution of RRR-CDs (). The TEM image shows that the RRR-CDs were nearly spherical, the particle size distribution was concentrated in the range of 1.4 nm−4.5 nm, and the distribution was uniform. HRTEM images of the RRR-CDs indicated that the lattice distance of RRR-CDs was 0.132 nm.
Figure 2. Characterization of Rhei radix et rhizoma-derived carbon dots. (A) Low-resolution transmission electron microscope image. Inset: Histogram of particle size distribution. (B) XRD pattern. (C) Ultraviolet visible specturm. (D) High-resolution transmission electron microscope image. (E) Excitation and emission fluorescence spectra. (F) Fourier transform infrared spectroscopy.
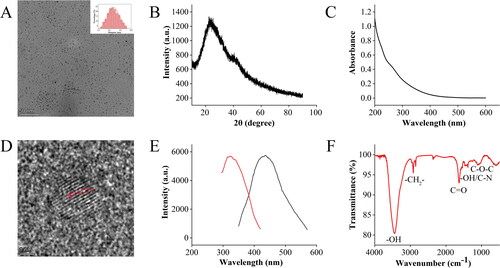
The UV-Vis absorption spectrum revealed that RRR-CDs had a broad spectrum without clear peaks (). RRR-CDs showed maximum fluorescence emission at 436 nm upon excitation at 321 nm ().
The surface chemical characteristics of CDs were characterised by FTIR spectroscopy (). The strong characteristic absorption peak at 3446.18 cm−1 may be the stretching vibration peak of -OH. The absorption peaks at 2918.51 cm−1 and 2850.34 cm−1 correspond to –CH2– stretching. RRR-CDs showed a C=O absorption peak at 1635.12 cm−1. At 1384.29 cm−1, the absorption peak may be the in-plane bending vibration peak of –OH or the stretching vibration peak of the C–N bond. The position of 1111.15 cm−1 was the absorption peak of C–O–C, which indicates the existence of the C–O–C bond.
The wide-angle XRD pattern in shows that there is a diffraction peak at approximately 2θ = 23.5°, which is in good agreement with the lattice parameters of RRR-CDs.
XPS was used to obtain the surface element composition and microchemical environment of the RRR-CDs. As shown in , the CDs contain three main peaks of C (67.99%), O (28.36%), and N (3.33%), which correspond to 284.9, 531.76, and 399.72 eV of the spectrum, respectively. In addition, there was a trace amount of S (0.01%). The C1s spectrum of RRR-CDs given in could be divided into three component peaks: 285.21 eV (C–N), 284.38 eV (C–C/C=C), and 287.96 eV (C–N/C–O) [Citation27,Citation28]. The high-resolution O1s spectrum () mainly contained two subpeaks corresponding to 531.28 eV (C-O) and 532.52 eV (C–OH) [Citation29,Citation30]. In addition, the two peaks 399.9 and 399.09 eV [Citation31,Citation32] in the N1s spectrum () confirmed the existence of C–N–C and N–H bonds.
3.2. Toxicity evaluation of RRR-CDs
The experimental results () showed that the RRR-CDs still did not show cytotoxicity at concentrations up to 1,000 μg/mL but instead had little effect on promoting cell proliferation.
3.3. Haemostatic evaluation of RRR-CDs
The results indicated that the tail bleeding time of mice in the RRR-CDs-treated groups were significantly shortened. As shown in , compared with the control group (318.00 ± 44.03 s), the positive group (227.33 ± 10.19 s) significantly shortened the tail haemorrhage time of mice, while the effect of RRR-CDs (H: 252.83 ± 12.23 s; M: 230.50 ± 12.55 s; L: 245.17 ± 11.48 s) intervention on the tail bleeding time was as good as that of the positive drug.
3.4. RRR-CDs alleviated DSS-Induced UC in rats
The experimental time schedule of DSS-induced acute UC was established as shown in . DSS-induced colitis mainly manifests as obvious weight loss, diarrhoea, bloody stool, and other symptoms. In this study, it was observed that the mental state of the mice in the model group was gradually sluggish, the reaction was slow, the activity was poor, the grasping power was decreased, and the hair was dull. In contrast, everything including mental state, activity, hair gloss, etc., in the control group was fairly fine, and the mental state of the positive group and administration groups was good, which was greatly improved compared with the model group. The activity was increased, and the hair was slightly dull. Similarly, it was found that the model group (16.93 ± 0.91 g) experienced significant weight loss compared to the control group (22.1 ± 0.97 g), However, the RRR-CDs high-, medium-, and low-dose group modulated weight loss in mice during the disease (H:19.52 ± 2.27 g p < 0.01; M:20.77 ± 0.33 g p < 0.01; L:17.18 ± 1.92 g), as shown in . At the same time, the DAI score is the severity of colitis commonly used as an evaluation index. The study found that the DAI score of the model (2.83 ± 0.40) group was extremely increased after DSS intake, and RRR-CDs (H: 1.89 ± 1.06, p < 0.01; M:1.22 ± 0.17, p < 0.01; L: 1.94 ± 0.57, p < 0.01) dramatically decreased the DAI score of experimental colitis (). Colon shortening is often associated with this disease, and RRR-CDs have a sensibly inhibitory effect on colon shortening, as shown in .
Figure 6. Effects of Rhei radix et rhizoma-carbon dots on DSS-induced UC in mice. (A) Experimental schedule of the DSS-induced UC model in mice. (B) Body weight changes. (C) DAI score. (D) Colon length histogram. (E) Colon tissue photographs.Control: normal saline; SASP: Sulfasalazine; H, M, L: high-, medium-, and low-dose (0.23, 0.12, and 0.06 mg/kg) RRR-CDs-administered groups, respectively. Values are means ± SD, *p<0.05; **p<0.01 compared with the model group; ##p<0.01 compared with the control group.
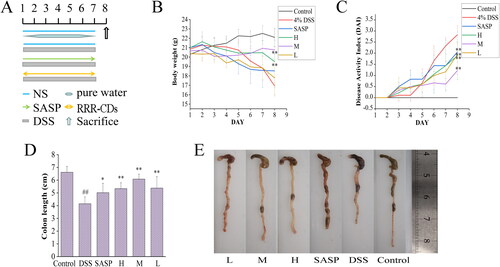
3.5. RRR-CDs ameliorated DSS-induced colitis histopathological damage
H&E staining was performed on different groups to explore the histopathological features of the colon, revealing DSS-induced damage and the protective biological activity of RRR-CDs, as shown in . The sections clearly showed that the colon tissue mucosa in the control group () was intact, the mucosal cells were neatly arranged, and there was no inflammatory cell infiltration. In contrast, in the DSS-induced model group (), the colon tissue mucosal structure was incomplete, the crypts partially disappeared, and the mucosal inflammatory cell infiltration was obvious. After RRR-CDs treatment, colon tissue in the RRR-CDs groups () was significantly protected. Among them, the mucosal structure of the RRR-CDs medium group () was close to normal, the cells were arranged neatly, and the infiltration of inflammatory cells was reduced.
Figure 7. Rhei radix et rhizoma-carbon dots ameliorate the histological changes of DSS-induced UC in mice. Histopathological sections of each group (A-F) were stained with H&E, scale bar = 50μm, 200×; 100μm, 400×. (A) Control group [normal saline]. (B) Model group [DSS]. (C) Positive group [Sulfasalazine]. (D) RRR-CDs High-does group. (E) RRR-CDs Mediun-dose group. (F) RRR-CDs Low-dose group.
![Figure 7. Rhei radix et rhizoma-carbon dots ameliorate the histological changes of DSS-induced UC in mice. Histopathological sections of each group (A-F) were stained with H&E, scale bar = 50μm, 200×; 100μm, 400×. (A) Control group [normal saline]. (B) Model group [DSS]. (C) Positive group [Sulfasalazine]. (D) RRR-CDs High-does group. (E) RRR-CDs Mediun-dose group. (F) RRR-CDs Low-dose group.](/cms/asset/ecc71148-cd78-46af-bc0e-04bcde3b8426/ianb_a_2197947_f0007_c.jpg)
3.6. Effect of RRR-CDs on the levels of inflammatory cytokines in colon tissue
The effect of RRR-CDs on the levels of inflammatory cytokines in the colon was evaluated by measuring the levels of TNF-α, IL-6, and IL-10 in colon tissue, as shown in . Compared with that in the control group (6.83 ± 1.17 ng/mL), the content of TNF-α in the colon tissue of the model group (12.25 ± 2.10 ng/mL) was increased (p < 0.01). Compared with the model group, the content of TNF-α in the colon tissue of mice in the SASP (9.93 ± 2.76 ng/mL) and the RRR-CDs (H: 9.86 ± 1.28 ng/mL; M: 7.95 ± 2.43 ng/mL; L: 9.21 ± 3.32 ng/mL) groups showed a decreasing trend, and the difference between the RRR-CDs medium and low groups was statistically significant (M: p < 0.01; L: p < 0.05).
Figure 8. Effects of Rhei radix et rhizoma-carbon dots on the level of TNF-α, IL-6, and IL-10 in colon tissue. Mice were treated with NS, SASP, and different concentrations of Rhei radix et rhizoma carbonisata-derived carbon dots (H: 0.23; M: 0.12, L: 0.06 mg/kg). ##p<0.01 compared with the control group; *p<0.05; **p<0.01 compared with the model group. Values are means ± SD. Control: normal saline; SASP: Sulfasalazine; H: RRR-CDs high dose; M: RRR-CDs medium dose; L: RRR-CDs low dose.
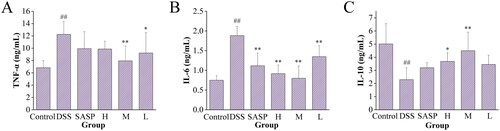
The content of IL-6 in the tissue was detected by ELISA (). IL-6 was significantly activated in the model (1.88 ± 0.23 ng/mL) group that drank DSS compared to the control (0.75 ± 0.11 ng/mL) group that drank pure water (p < 0.01). However, the level of IL-6 decreased in the RRR-CDs (H: 0.91 ± 0.21 ng/mL, p < 0.01; M: 0.80 ± 0.31 ng/mL, p < 0.01; L: 1.35 ± 0.27 ng/mL, p < 0.01) treatment group and SASP (1.11 ± 0.32 ng/mL) group, and there were significant differences.
In addition, presents the concentration of IL-10 in mouse colon tissue. Compared with the control (5.01 ± 1.54 ng/mL) group, the model (2.30 ± 0.89 ng/mL) group showed a significant decrease (p < 0.01), and the SASP (3.20 ± 0.4 ng/mL) group and the RRR-CDs (H: 3.68 ± 0.65 ng/mL; M: 4.50 ± 1.51 ng/mL; L: 3.45 ± 1.70 ng/mL) group showed an upwards trend, of which the high-dose (p < 0.05) group and the middle-dose (p < 0.01) group increased significantly, while the SASP group and the low-dose group increased IL-10 concentrations, but the difference was not significant (p > 0.05).
3.7. Effect of RRR-CDs on the levels of oxidative stress in colon tissue
To further verify the protective effect of RRR-CDs on UC, the antioxidant capacity was measured by the content of enzyme antioxidant (CAT, SOD) and nonenzyme antioxidant (GSH) in intestinal tissues (). Intestinal tissue damage caused by oxidative stress was assessed by the levels of MDA and MPO (). After DSS intake, MPO and MDA contents in the intestinal canal of mice in the model (MPO: 0.29 ± 0.10 U/g, p < 0.01; MDA: 29.83 ± 5.75 U/mg prot, p < 0.01) group increased sharply compared with the control (MPO: 0.07 ± 0.03 U/g; MDA: 12.16 ± 2.19 U/mg prot) group. In contrast, the RRR-CDs (MPO: H: 0.12 ± 0.03 U/g, p < 0.01; M: 0.08 ± 0.01 U/g, p < 0.01; L: 0.12 ± 0.03 U/g, p < 0.01; MDA: H: 20.33 ± 5.47 U/mg prot, p < 0.01; M: 17.16 ± 1.95 U/mg prot, p < 0.01; L: 24.00 ± 4.93 U/mg prot, p < 0.05) administration group largely inhibited intestinal damage caused by peroxide.
Figure 9. Effects of Rhei radix et rhizoma-carbon dots on the level of SOD, CAT, GSH, MPO, and MDA in colon tissue. Mice were treated with NS, SASP, and different concentrations of Rhei radix et rhizoma carbonisata-derived carbon dots (H:0.23; M:0.12, L:0.06 mg/kg). ##p<0.01 compared with the control group; *p<0.05; **p<0.01 compared with the model group. Values are means ± SD. Control: normal saline; SASP: Sulfasalazine; H: RRR-CDs high dose; M: RRR-CDs medium dose; L: RRR-CDs low dose.
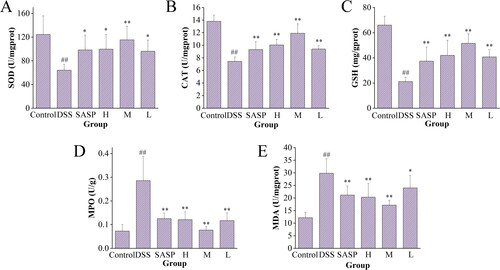
shows SOD activity values in each group. The contents of SOD in the colon tissue of mice in the model (64.23 ± 9.68 U/mg prot) group was significantly reduced (p < 0.01), while SOD activity in the positive (98.40 ± 25.09 U/mg prot, p < 0.05) group and RRR-CDs high- (99.81 ± 24.68 U/mg prot, p < 0.05), medium- (115.46 ± 23.00 U/mg prot, p < 0.01), and low- (96.20 ± 18.83 U/mg prot, p < 0.05) dose groups was increased to varying degrees, especially in the medium-dose group (p < 0.01).
Similarly, the content of CAT and GSH in colon tissue exhibited consistent results (). After ingesting DSS solution, the content of the model group decreased significantly (CAT: 7.45 ± 0.70 U/mg prot, p < 0.01; GSH: 21.16 ± 3.50 mg/g prot, p < 0.01) compared with control group (CAT: 13.80 ± 0.99 U/mg prot; GSH: 66.05 ± 7.03 mg/g prot). After treatment with positive drugs (CAT: 9.32 ± 1.26 U/mg prot, p < 0.01; GSH: 37.42 ± 11.21 mg/g prot) and RRR-CDs (CAT: H: 10.05 ± 0.89 U/mg prot, p < 0.01; M: 11.92 ± 1.50 U/mg prot, p < 0.01; L: 9.41 ± 0.53 U/mg prot, p < 0.01; GSH: H: 41.97 ± 12.06 mg/g prot, p < 0.01; M: 51.60 ± 7.44 mg/g prot, p < 0.01; L: 40.78 ± 5.79 mg/g prot, p < 0.01), the contents of CAT and GSH were significantly reversed.
3.8. Effects of RRR-CDs on the expression of tight junction protein
As shown in , the tight junction proteins ZO-1, occludin and claudin-1 were positively expressed in the control group, mostly with brownish-yellow staining; compared with the control group, ZO-1, occludin, and claudin-1 in the model group were decreased, and the expressions of these three proteins was increased in the positive group and each dose of RRR-CDs groups, among which the middle dose group had the best effect.
Figure 10. The expression of tight junction proteins in the intestinal tract of mice with UC. Antigen-antibody binding-stained sections. Scale bar = 20μm, 630×. Control: normal saline; SASP: Sulfasalazine; H, M, L: high-, medium-, and low-dose (0.23, 0.12, and 0.06 mg/kg) RRR-CDs-administered groups, respectively.
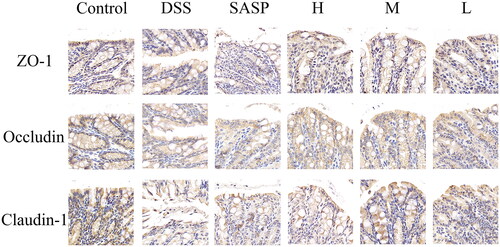
4. Discussion
Researchers are increasingly hot on nanomaterials because of their excellent photobleaching resistance [Citation32], photochemical stability [Citation33], low toxicity [Citation34], and other characteristics, and CDs have begun to become a new generation of nanoinnovative materials to occupy an important market. Existing research reports [Citation35] suggest that research on CDs is mostly from the perspective of nanocarriers and inherent biological activities. For example, doxorubicin-loaded polymer-coated carbon nanodots can pass through the blood-brain barrier and realise the pH-responsive sustained release of doxorubicin, enabling chemotherapeutic drug delivery into the brain [Citation36]. In addition, the anti-inflammatory activity of aspirin-based carbon dots has been demonstrated [Citation37]. It can be clearly seen that carbon dots, both as carriers and for their diverse inherent biological activities, provide new attempts and methods for the clinical treatment of thorny problems based on various advantages.
The superiority of CDs is closely related to their preparation methods. With the deepening of the research on CDs, the disadvantages of the preparation methods of CDs are gradually exposed due to their arc discharge, chemical oxidation, and hydrothermal properties [Citation38], which require strong acid and complex processes. The improved high-temperature pyrolysis method meets the preparation requirements of low cost, easy operation, low toxicity, and environmental friendliness. It is worth noting that the preparation process of this RRR is similar to that of CD [Citation39], which is conducive to the RRR's dominance.
In recent years, TCM has become increasingly active in the field of nanomaterials as part of the Chinese medical system. It has made outstanding contributions to the source and abundance of CDs, and some of the biological activities of CDs have been studied in the previous literature. For example, jujube carbon dots promote erythrocyte proliferation [Citation40], Glycyrrhizae Radix et Rhizoma (GRR) carbon dots with GRR as a precursor have been shown to have anti-gastric ulcer activity [Citation41], and Paeoniae Radix Alba-based CDs have liver-protecting activity [Citation42]. The RRR-CDs studied in this paper described a new type of carbon-based nanoparticles with a size distribution ranging from 1.374 nm to 4.533 nm, which was prepared from the natural product RRR as the carbon source.
Recently, there have been studies on the application of CDs in the treatment of UC. For example, carbon dots-SOD nanozymes are coated on metal-organic carriers, and their biomimetic nanocomplexes are delivered into the intestine to play a role [Citation43]. However, thus far, there is no research to prove that CDs have their own biological activity to treat UC induced by DSS. This study fills this gap and is the first to demonstrate protection against UC using the biological activity of RRR-CDs itself.
For UC, inflammation and oxidative stress have been most extensively studied as common cause. RRR-CDs have significant therapeutic effects, both symptomatic treatment and underlying mechanisms of treatment. The mouse tail tip bleeding experiment showed that RRR-CDs can effectively shorten the tail tip bleeding time in mice and achieve the purpose of hemostasis; at the same time, the nanoparticles have a physical adsorption effect [Citation42]. Studies have shown that polyacrylonitrile-based nanofibers obtain hydrophilicity by adding nanoparticles and simulate body fluids to analyse their water absorption capacity [Citation44]. Based on these studies, RRR-CDs can play an effective role in treating acute symptoms such as diarrhoea and blood in the stool to significantly reduce the DAI score and alleviate colon damage.
In addition, RRR-CDs protected the intestinal epithelial mucosal barrier by reducing the levels of oxidative stress and inflammation, which may be a potential mechanism by which RRR-CDs exert protective effects. Uninterrupted DSS solution stimulation can lead to overexpression of ROS, and sustained production of ROS may continuously deplete endogenous antioxidants, leading to lipid peroxidation and exacerbating colon epithelium damage [Citation45]. Inhibiting the production of oxidants such as lipid peroxides or increasing the levels of antioxidants can attenuate DSS-induced colon damage [Citation46]. The above detection levels of SOD, GSH, CAT, MPO, and MDA also confirmed the results of RRR-CDs alleviating oxidative stress levels.
Inflammatory response is an important factor in the occurrence, development and aggravation of UC. The mechanism by which DSS causes UC is still unclear, but as an irritant, it not only damages the physical barrier of the intestinal but also easily stimulates mucosal immunity, resulting in the overexpression of innate immune cells such as neutrophils, which continuously damage the intestinal tract, resulting in inflammation [Citation47]. Or drive M1 macrophages to produce inflammatory factors such as TNF-α and IL-6 [Citation19]. The persistence of these inflammations leads to a vicious cycle in the intestinal microenvironment, as evidenced by inflammation-related carcinogenesis models [Citation48]. Popivanova and colleagues found that TNF-α may play an important role in UC and that loss of TNF-α signalling in mice significantly ameliorated epithelial mucosal barrier damage and inflammatory cell infiltration [Citation49] to protect the colonic epithelium. The intervention of RRR-CDs in this study significantly reduced the levels of pro-inflammatory factors TNF-α and IL-6 and delayed the further development of colonic inflammation.
Under normal circumstances, the intestinal tract has a complete barrier function, which can effectively isolate the invasion of bacteria and pathogenic microorganisms in the intestinal contents and maintain the normal operation of the body. However, irritants stimulate the intestinal epithelial mucosa and damage tight junction proteins, resulting in various pathogenic microorganisms easily invading the body and further damaging the integrity of the intestinal epithelium and causing inflammation. In this paper, the distribution of ZO-1, Occludin and Claudin-1 proteins in the colon was detected to judge the integrity of the colonic mucosal barrier. The results showed that the expression of the tight junction proteins ZO-1, occludin, and claudin-1 in the model group was significantly reduced, proving that the intestinal barrier of mice was damaged. After intervention with RRR-CDs, the distribution and expression of tight junction proteins rebounded significantly, which was consistent with the detection results of oxidative stress and inflammatory factors in vivo.
This study verified the protective effect of RRR-CDs on DSS-induced UC from both symptomatic treatment, such as hemostasis, and potential mechanism treatment and explained that the underlying mechanism was related to inflammatory factors, oxidative stress, and intestinal mucosa. This research provides nanomedicine (such as CDs) treatment ideas for tricky clinical problems. In addition, deeper mechanisms need to be examined and explored.
5. Conclusions
In summary, this study uses the natural product RRR as the carbon source to synthesise and identify RRR-CDs, which have excellent biological activity, using a one-step pyrolysis method. For the first time, it was demonstrated that RRR-CDs have protective effects on the mucosal barrier of UC from the aspects of hemostasis, anti-inflammation and antioxidation. The potential mechanism of action of RRR-CDs is related to the downregulation of downstream proinflammatory factors, upregulation of anti-inflammatory factors, inhibition of lipid peroxidation, enhancement of oxygen free radical scavenging ability and protection of intestinal epithelial tight junction proteins ZO-1, Occludin and Claudin-1. This research provides nanomedicine (such as CDs) treatment ideas for addressing complex clinical problems and further expands the scope of CDs biological activity.
Ethical approval
Research experiments conducted in this article with animals were approved by the Ethical Committee of Animal Experimentation of Beijing University of Chinese Medicine following all guidelines, regulations, legal, and ethical standards as required for animals.
Author contributions
Yan Zhao, Huihua Qu, Hui Kong, Yifan Zhang and Jie Zhao participated in the concept and design of this study; Yifan Zhang, Xue Bai, Yue Zhang and Yumin Chen obtained of data for the work; Jie Zhao, Yuhan Liu and Yusheng Zhao analysed the data; Yifan Zhang, Jie Zhao, Yusheng Zhao and Yumin Chen gathered information and wrote the manuscript; Yan Zhao and Huihua Qu agreed to be accountable for all aspects of the work
Disclosure statement
No potential conflict of interest was reported by the author(s).
Data availability statement
All data generated or analysed during this study are included in this published article.
Additional information
Funding
References
- Wilks S. Morbid appearances in the intestine of miss bankes. London Med Times Gazette. 1859;2:264.
- Feuerstein JD, Moss AC, Farraye FA. Ulcerative colitis. Mayo Clin Proc. 2019;94(7):1357–1373.
- Hu Y, Ye Z, She Y, et al. Efficacy and safety of probiotics combined with traditional Chinese medicine for ulcerative colitis: a systematic review and meta-analysis. Front. Pharmacol. 2022;13:844961.
- Wang J, Zhang C, Guo C, et al. Chitosan ameliorates DSS-induced ulcerative colitis mice by enhancing intestinal barrier function and improving microflora. IJMS. 2019;20(22):5751.
- Guo G, Shi F, Zhu J, et al. Piperine, a functional food alkaloid, exhibits inhibitory potential against TNBS induced colitis via the inhibition of IκB-α/NF-κB and induces tight junction protein (claudin-1, occludin, and ZO-1) signaling pathway in experimental mice. Hum Exp Toxicol. 2020;39(4):477–491.
- Kucharzik T, Koletzko S, Kannengiesser K, et al. Ulcerative colitis-diagnostic and therapeutic algorithms. Dtsch Arztebl Int. 2020;117:564–574.
- Alaghmandfard A, Sedighi O, Tabatabae Rezaei N, et al. Recent advances in the modification of carbon-based quantum dots for biomedical applications. Mater Sci Eng C Mater Biol Appl. 2021;120:111756.
- Zhao DL, Chung TS. Applications of carbon quantum dots (CQDs) in membrane technologies: a review. Water Res. 2018;147:43–49.
- Kang Z, Lee ST. Carbon dots: advances in nanocarbon applications. Nanoscale. 2019;11(41):19214–19224.
- Cohen EN, Kondiah PPD, Choonara YE, et al. Carbon dots as nanotherapeutics for biomedical application. Curr Pharm Des. 2020;26(19):2207–2221.
- Miao H, Wang L, Zhuo Y, et al. Label-free fluorimetric detection of CEA using carbon dots derived from tomato juice. Biosens Bioelectron. 2016;86:83–89.
- Zhang X, Jiang M, Niu N, et al. Natural-product-derived carbon dots: from natural products to functional materials. ChemSusChem,. 2018;11(1):11–24.
- Nair A, Haponiuk JT, Thomas S, et al. Natural carbon-based quantum dots and their applications in drug delivery: a review. Biomed Pharmacother. 2020;132:110834.
- Purbia R, Paria S. A simple turn on fluorescent sensor for the selective detection of thiamine using coconut water derived luminescent carbon dots. Biosens Bioelectron. 2016;79:467–475.
- Devi P, Saini S, Kim KH. The advanced role of carbon quantum dots in nanomedical applications. Biosens Bioelectron. 2019;141:111158.
- Bharathi D, Siddlingeshwar B, Krishna RH, et al. Green and cost effective synthesis of fluorescent carbon quantum dots for dopamine detection. J Fluoresc. 2018;28(2):573–579.
- Zhou Y, Zhu F, Liu Y, et al. Blood-brain barrier–penetrating siRNA nanomedicine for Alzheimer’s disease therapy. Sci. Adv. 2020;6(41):7031.
- Pei J, Zhao R, Mu X, et al. Single-atom nanozymes for biological applications. Biomater Sci. 2020;8(23):6428–6441.
- Zhang M, Cheng J, Hu J, et al. Green phellodendri chinensis cortex-based carbon dots for ameliorating imiquimod-induced psoriasis-like inflammation in mice. J Nanobiotechnology. 2021;19(1):105.
- Zhao Y, Zhang Y, Kong H, et al. Protective effects of carbon dots derived from Armeniacae Semen Amarum carbonisata against acute lung injury induced by lipopolysaccharides in rats. Int J Nanomedicine. 2022;17(4):1–14.
- Liu X, Wang Y, Yan X, et al. Novel phellodendri cortex (Huang Bo)-derived carbon dots and their hemostatic effect. Nanomedicine. 2018;13(4):391–405.
- Wang Y, Kong H, Liu X, et al. Novel carbon dots derived from Cirsii Japonici Herba Ccarbonisata and their haemostatic effect. J Biomed Nanotechnol. 2018;14(9):1635–1644.
- Gao C, Zhou Y, Chen Z, et al. Turmeric-derived nanovesicles as novel nanobiologics for targeted therapy of ulcerative colitis. Theranostics. 2022;12(12):5596–5614.
- Zhang Q, Xu N, Hu X, et al. Anti-colitic effects of physalin B on dextran sodium sulfate-induced BALB/c mice by suppressing multiple inflammatory signaling pathways. J Ethnopharmacol. 2020;259:112956.
- Huang JQ, Wei SY, Cheng N, et al. Chimonanthus nitens oliv. Leaf granule ameliorates DSS-induced acute colitis through treg cell improvement, oxidative stress reduction, and gut microflora modulation. Front Cell Infect Microbiol. 2022;12:907813.
- Hidalgo-Garcia L, Molina-Tijeras JA, Huertas-Peña F, et al. Intestinal mesenchymal cells regulate immune responses and promote epithelial regeneration in vitro and in dextran sulfate sodium-induced experimental colitis in mice. Acta Physiol. 2021;233(2):e13699.
- Yue L, Li H, Liu Q, et al. Manganese-doped carbon quantum dots for fluorometric and magnetic resonance (dual mode) bioimaging and biosensing. Mikrochim Acta. 2019;186(5):315.
- Zhang M, Cheng J, Zhang Y, et al. Green synthesis of Zingiberis rhizoma-based carbon dots attenuates chemical and thermal stimulus pain in mice. Nanomedicine. 2020;15(9):851–869.
- Wang X, Zhang Y, Kong H, et al. Novel mulberry silkworm cocoon-derived carbon dots and their anti-inflammatory properties. Artif Cells Nanomed Biotechnol. 2020;48(1):68–76.
- Zhao Y, Zhang Y, Kong H, et al. Haemostatic nanoparticles-derived bioactivity of from Selaginella tamariscina Carbonisata. Molecules. 2020;25(3):446. pp.
- Kirbas Cilingir E, Seven ES, Zhou Y, et al. Metformin derived carbon dots: highly biocompatible fluorescent nanomaterials as mitochondrial targeting and blood-brain barrier penetrating biomarkers. J Colloid Interface Sci. 2021;592:485–497.
- Agrawal M, Prathyusha E, Ahmed H, et al. Biomaterials in treatment of Alzheimer’s disease. Neurochem Int. 2021;145:105008.
- Mondal S, Yucknovsky A, Akulov K, et al. Efficient photosensitizing capabilities and ultrafast carrier dynamics of doped carbon dots. J Am Chem Soc. 2019;141(38):15413–15422.
- Pardo J, Peng Z, Leblanc RM. Cancer targeting and drug delivery using Carbon-Based quantum dots and nanotubes. Molecules. 2018;23(2):378.
- Henna TK, Pramod K. Graphene quantum dots redefine nanobiomedicine. Mater Sci Eng C Mater Biol Appl. 2020;110:110651.
- Henna TK, Raphey VR, Sankar R, et al. Carbon nanostructures: the drug and the delivery system for brain disorders. Int J Pharm. 2020;587:119701.
- Xu X, Zhang K, Zhao L, et al. Aspirin-based carbon dots, a good biocompatibility of material applied for bioimaging and anti-inflammation. ACS Appl Mater Interfaces. 2016;8(48):32706–32716.
- Ge G, Li L, Wang D, et al. Carbon dots: synthesis, properties and biomedical applications. J Mater Chem B. 2021;9(33):6553–6575.
- Tejwan N, Saha SK, Das J. Multifaceted applications of green carbon dots synthesized from renewable sources. Adv Colloid Interface Sci. 2020;275:102046.
- Xu Y, Wang B, Zhang M, et al. Carbon dots as a potential therapeutic agent for the treatment of cancer-related anemia. Adv Mater. 2022;34(19):e2200905.
- Liu Y, Zhang M, Cheng J, et al. Novel carbon dots derived from Glycyrrhizae Radix et Rhizoma and their anti-gastric ulcer effect. Molecules. 2021;26(6):1512.
- Zhao Y, Zhang Y, Kong H, et al. Carbon dots from Paeoniae Radix Alba Carbonisata: hepatoprotective effect. Int J Nanomedicine. 2020;15:9049–9059.
- Ma Y, Gao W, Zhang Y, et al. Biomimetic MOF nanoparticles delivery of C-dot nanozyme and CRISPR/Cas9 system for site-specific treatment of ulcerative colitis. ACS Appl Mater Interfaces. 2022;14(5):6358–6369.
- Docter D, Distler U, Storck W, et al. Quantitative profiling of the protein coronas that form around nanoparticles. Nat Protoc. 2014;9(9):2030–2044.
- Amirshahrokhi K. Febuxostat attenuates ulcerative colitis by the inhibition of NF-κB, oinflammatory cytokines, and oxidative stress in mice. Int Immunopharmacol. 2019;76:105884.
- Boeing T, de Souza P, Bonomini TJ, et al. Antioxidant and anti-inflammatory effect of plumieride in dextran sulfate sodium-induced colitis in mice. Biomed Pharmacother. 2018;99:697–703.
- Ahluwalia B, Moraes L, Magnusson MK, et al. Immunopathogenesis of inflammatory bowel disease and mechanisms of biological therapies. Scand J Gastroenterol. 2018;53(4):379–389.
- Zhang S, Li J, Xie P, et al. STAT3/c-Myc axis-mediated metabolism alternations of inflammation-related glycolysis involve with colorectal carcinogenesis. Rejuvenation Res. 2019;22(2):138–145.
- Yao D, Dong M, Dai C, et al. Inflammation and inflammatory cytokine contribute to the initiation and development of ulcerative colitis and its associated cancer. Inflamm Bowel Dis. 2019;25(10):1595–1602.