Abstract
Low-intensity pulsed ultrasound (LIPUS) is a kind of therapeutic ultrasound. It can help improve bone fracture repair and soft tissue healing. Our previous study found that LIPUS treatment could halt the chronic kidney disease (CKD) progression in mice; unexpectedly, we observed the improvement of CKD-reduced muscle weights by LIPUS treatment. Here, we further tested the protective potential of LIPUS on CKD-associated muscle wasting/sarcopenia using the CKD mouse models. Mouse models of both unilateral renal ischemia/reperfusion injury (IRI) with nephrectomy and adenine administration were used to induce CKD. LIPUS with condition of 3 MHz, 100 mW/cm2, 20 min/day was applied to the kidney of CKD mice. LIPUS treatment significantly reversed the increased serum BUN/creatinine levels in CKD mice. LIPUS effectively prevented the decrease in grip strength, muscle weight (soleus, tibialis anterior, and gastrocnemius muscles), cross-section areas of muscle fibres, and muscular phosphorylated Akt protein expression by immunohistochemistry, and the increase in muscular atrogenes Atrogin1 and MuRF1 protein expression by immunohistochemistry in CKD mice. These results indicated that LIPUS could help improve weak muscle strength, muscle mass loss, muscle atrophy-related protein expression, and Akt inactivation. LIPUS application may be an alternative non-invasive therapeutic intervention on the management of CKD-associated muscle wasting.
Introduction
Chronic kidney disease (CKD) is considered one of the leading causes of death in the twenty-first century; it affects more than 10% of the world’s total population, totalling more than 800 million people [Citation1]. In the U.S. adult individuals, the prevalence of CKD is estimated to be 14.0%, based on the National Health and Nutrition Examination Survey (NHANES) data between 2017 and March 2020 [Citation2]. The prevalence of cachexia in adults with CKD requiring maintenance dialysis ranges from 28 to 80% [Citation3]. Cianciaruso and colleagues have observed that 51.0% of continuous ambulatory peritoneal dialysis patients and 38.6% of maintenance haemodialysis patients have mild to severe muscle wasting [Citation4]. Bataille and colleagues reported that 31.5% of haemodialysis patients had sarcopenia [Citation5]. Sarcopenia has also been found to be highly prevalent in elderly end-stage renal disease (ESRD) patients with 37.0% in men and 29.3% in women [Citation6]. A previous study has suggested that the maintenance of physical performance is an essential factor for improving the CKD prognosis [Citation7]. Aerobic exercise has recently been observed to alleviate uraemic cachexia and muscle wasting in a CKD animal model [Citation8]. Testosterone and growth hormone replacement are suggested to be the treatment strategies for muscle wasting [Citation7]. However, the current scientific approach to effective prevention of CKD-related cachexia/muscle wasting is still insufficient. CKD/ESRD patients with cachexia/muscle wasting are in urgent need of reliable approaches or new treatment strategies, especially in the elderly, frail, sedentary, and exercise-hesitant patient groups [Citation7].
Therapeutic ultrasound is considered safe and non-invasive. A low intensity pulsed ultrasound (LIPUS) device has been approved by the US FDA for fresh fracture therapy in 1994; in 2000, the US FDA approved LIPUS for the treatment of established non-unions [Citation9]. LIPUS treatment was also found to improve chronically pressure-overload-induced contractile dysfunction in hearts by enhancing myocardial angiogenesis and attenuating perivascular fibrosis [Citation10]. It has been found that the type 1 diabetes-related skeletal muscle atrophy in rats could be prevented by LIPUS [Citation11]. However, the therapeutic potential on CKD-associated muscle wasting by LIPUS treatment needs further evaluation.
Our previous findings showed that treatment with LIPUS in CKD mice can improve CKD progression and prevent kidney disease cachexia by inhibiting renal fibrosis and slowing down renal senescence [Citation12]. Unexpectedly, we observed that LIPUS treatment could also protect against CKD-reduced muscle weights. We therefore hypothesized that LIPUS application may possess the therapeutic potential for improving CKD-associated muscle wasting/sarcopenia. To test this hypothesis, in this study, we investigated the efficacy of LIPUS on CKD-induced muscle wasting/sarcopenia using two CKD mouse models. LIPUS with condition of 3 MHz, 100 mW/cm2, 20 min/day was applied to the kidney in both ischemia/reperfusion injury (IRI) and adenine administration-induced CKD mouse models mimicking our previous study [Citation12].
Methods
Experimental animals and CKD models
As experimental animals, six-week-old adult male C57BL/6J mice were purchased from the Laboratory Animal Centre of the National Taiwan University School of Medicine, where the animal experiments were performed. Animals were housed in cages maintained at 22 ± 2 °C with a 12-h light/dark cycle. Animals were fed the standard rodent chow diet and water ad libitum. Animal experimental procedures, which were approved by the Institutional Animal Care and Use Committee of the National Taiwan University College of Medicine (IACUC Approval No.: 20180324), were performed in accordance with the Taiwan regulations and the National Institutes of Health (USA) guidelines.
Two CKD mouse models of IRI induction and adenine administration were performed as previously described [Citation12–14]. In brief, for IRI model, the mouse’s left renal artery was clamped by a non-traumatic artery clamp for 30 min before reperfusion to induce IRI. The nephrectomy for mouse’s right kidney was performed 24 h before euthanasia. After 14 days of IRI, animals were euthanized. All operations including euthanasia in mice were performed under anaesthesia. Moreover, for adenine model, mice were orally administered 50 mg/kg adenine in 0.5% CMC for 28 days until euthanasia. The blood samples were collected and skeletal muscles (soleus, tibialis anterior and gastrocnemius muscle) were isolated and weighed after animal euthanasia.
LIPUS application
The conditions for LIPUS application in mice were performed as previously described [Citation12,Citation15]. In brief, a therapeutic ultrasound device Sonicator-740 (Mettler Electronics, Anaheim, CA, USA) with a 3 MHz single-element focussed transducer (1 cm2 diameter) was used to produce LIPUS. The regions of left kidneys in both IRI mice and adenine-administered mice were exposed by LIPUS for 20 min/day through a transducer with a spatial-peak temporal-average intensity of 100 mW/cm2. For IRI model, mice were pre-treated with LIPUS 5 days before IRI operation and continuously treated 14 days after IRI. For adenine mouse model, LIPUS was pre-treated 5 days before adenine administration and continuously treated 28 days after adenine administration.
Detection of serum creatinine and blood urea nitrogen (BUN)
The serum creatinine and BUN levels were detected by a commercially available clinical chemistry analyser (Roche, Rotkreuz, Switzerland).
Grip strength test
The grip strength was determined by a grip strength device (DTG-2, Bio-Cando, Taoyuan, Taiwan). The device was placed horizontally and the mouse tail was held in which allowed to grasp the wire of the device. The grip strengths of both forelimb and hindlimb for both models of CKD were recorded prior to the sacrifice of the mice. The measurements of every mouse of groups were repeated five times.
Histology analysis
The haematoxylin and eosin staining, Masson’s trichrome staining, and immunohistochemistry were performed as previously described [Citation16]. The 4μm thick sections prepared from the paraffin-embedded skeletal muscles were used. For immunohistochemistry, the primary antibodies for Atrogin-1 (#ab198958), MuRF1 (#ab183094), and phosphorylated Akt (#ab81283) (abcam, Boston, MA, USA) were used. The samples were relabelled for blinded analysis before immunohistochemistry processing. The sections were counterstained with haematoxylin and the signal visualisation was detected with a SuperPicture horseradish peroxidase polymer conjugate kit (Invitrogen, Carlsbad, CA, USA). The image J 1.48 software (National Institutes of Health, Bethesda, MD, USA) was used to calculate the fibrotic areas and cross-section areas of myofibers and the relative optical density of immunohistochemical images in five random visual fields of each section.
Statistical analysis
Data are presented as the mean ± SEM. The statistical analysis was determined by a one-way analysis of variance (ANOVA) followed by the unpaired two-tailed Student’s t-test using GraphPad Prism 6 software. To analyse the distribution of myofiber sizes from muscles, the two-way ANOVA followed by the Sidak test was used. Statistically significant differences are indicated by p < 0.05.
Results and discussion
The intensity for low-intensity therapeutic ultrasound is < 3 W/cm2, which the commonly used range applied in regular clinical therapy is 0.03–1 W/cm2 [Citation17]. The frequency range of ultrasound commonly used in therapeutic medicine is 0.7–3 MHz [Citation17]. A dosage of 20 min per day for LIPUS application is commonly used in experimental bone fracture models [Citation9] and kidney disease models [Citation12,Citation15]. Here, we tested the preventive effect of LIPUS, which condition included 3 MHz frequency, 0.1 W/cm2 intensity, and 20 min/day, on CKD-associated muscle wasting/sarcopenia in both IRI- and adenine-induced CKD mouse models.
Effects of LIPUS on body weight, renal function markers, grip strength, and muscle weight in two CKD mouse models
We first tested for changes in markers of renal function. The BUN and serum creatinine levels were significantly increased in these two CKD mouse models, which could also be significantly reversed by LIPUS treatment ( for IRI model, p < 0.05, n = 8; ) for adenine model, p < 0.05, n = 9). These results show that LIPUS has the ability to improve CKD as our previous research reported [Citation12].
Figure 1. Effects of LIPUS on the body weight, grip strength, and muscle weight in CKD mouse models. Two mouse models of unilateral IRI with contralateral nephrectomy (1) and adenine administration (2) were used. Body weight (A), grip strength for forelimb and hindlimb (B), and muscle weights for gastrocnemius, tibialis anterior, and soleus muscles (C) were shown. Data are presented as mean ± SEM (n = 8 for IRI model; n = 9 for adenine model). *p < 0.05, versus sham/control group; #p < 0.05, versus CKD alone group.
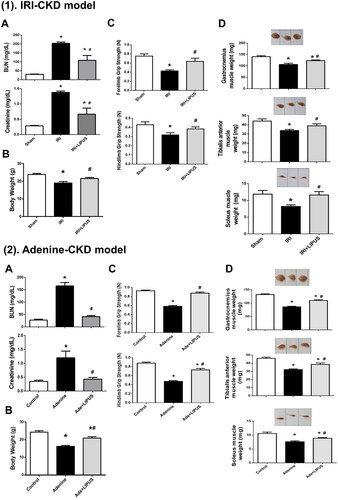
Loss of body weight and muscle mass is known as the one of characteristics of CKD cachexia [Citation3,Citation18]. Muscle wasting/sarcopenia is considered an important feature of the cachexia phenotype and may increase the risk of comorbidity [Citation3]. We next investigated the body weight, muscle strength, muscle weight, and muscle cross-section area in both IRI- and adenine-induced CKD mice with or without LIPUS treatment. The body weights were significantly decreased in both IRI- and adenine-induced CKD mice, which could be significantly reversed by LIPUS treatment () for IRI model, p < 0.05, n = 8; for adenine model, p < 0.05, n = 9). Moreover, LIPUS could also significantly improve the decrease in food intake in both IRI- and adenine-induced CKD mice (Supplementary Data-Figure 1).
Grip strength of forelimb and hindlimb in CKD mice was significantly decreased, which could be significantly reversed by LIPUS treatment ( for IRI model, p < 0.05, n = 8; for adenine model, p < 0.05, n = 9). Weights of the soleus, tibialis anterior, and gastrocnemius muscles were also significantly decreased in CKD mice, which could be effectively and significantly reversed by LIPUS treatment () for IRI model, p < 0.05, n = 8; ) for adenine model, p < 0.05, n = 9).
Figure 2. Effects of LIPUS on the cross-section areas of myofibers and fibrosis in soleus muscles of CKD mouse models. Two mouse models of unilateral IRI with contralateral nephrectomy (1) and adenine administration (2) were used. Cross-section areas (CSA) of myofibers (A-a), quantification of CSA (A-b), and distribution of myofibers (A-c) in soleus muscles were shown. Moreover, the collagen depositions in soleus muscles of IRI-CKD mice were stained by Masson’s Trichrome (B). The quantification was shown. Data are presented as mean ± SEM (n = 8 for IRI model; n = 9 for adenine model). *p < 0.05, versus sham group; #p < 0.05, versus CKD alone group.
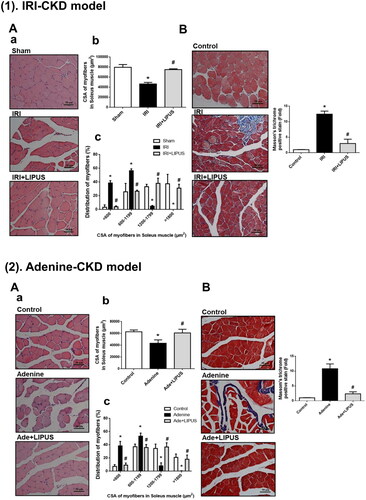
In our preliminary study, we designed 4 experimental groups for sham/control group, CKD group, CKD + LIPUS group, and sham/control + LIPUS group in both IRI- and adenine-CKD mouse models. The results showed that LIPUS alone (100 mW/cm2 intensity) did not affect the renal functional parameters and grip strength changes in sham control mice or normal control mice in both mouse models (Supplementary Data-Figure 2). Moreover, the mechanical index for LIPUS (1 MHz frequency) applied by Sonicator®-740 has been estimated to be 0.3 [Citation19] that is a safe condition for LIPUS exposure. Following the Animal Welfare Act and 3Rs principle for studies using animals, we did not proceed with the follow-up experiments testing effects of LIPUS alone on sham/control mice.
Effects of LIPUS on myofiber cross-section areas (CSA) and distribution and muscle collagen deposition in two CKD mouse models
CKD mice featured a marked decrease in average myofiber CSA in the soleus muscles ( for IRI model, p < 0.05, n = 8; for adenine model, p < 0.05, n = 9) with a leftward shift in the representative frequency distribution histogram of soleus myofiber CSA ( for IRI model, p < 0.05, n = 8; for adenine model, p < 0.05, n = 9) compared to the control mice. Treatment with LIPUS significantly ameliorated the changes of average myofiber CSA and distribution of myofiber CSA in CKD mice ( for IRI model, p < 0.05, n = 8; for adenine model, p < 0.05, n = 9). These results indicate that muscle wasting can be observed in these CKD mouse models, which was effectively prevented by LIPUS treatment.
Skeletal muscle fibrosis, which muscle collagen content and density are increased, has been demonstrated in rodent models and patients with CKD [Citation20,Citation21]. CKD-associated muscle fibrosis may injure physical function. We also examined whether LIPUS application reversed muscle fibrosis in CKD mice. We found that collagen deposition stained with Masson’s Trichrome in the soleus muscles of both IRI- and adenine-induced CKD mice was significantly increased; LIPUS treatment could significantly ameliorate the increased collagen deposition in the soleus muscles of CKD mice ( for IRI model, p < 0.05, n = 8; for adenine model, p < 0.05, n = 9). These findings suggest that LIPUS application is capable of improving the CKD-associated muscle fibrosis in mice.
Effects of LIPUS on muscle wasting/atrophy-related signalling molecules Akt and atrogens in two CKD mouse models
The protein wasting in muscles from CKD animal models has been demonstrated to be associated with the decreased protein synthesis and the increased proteolysis [Citation22]. The Akt signalling is known to play an important role in muscle protein synthesis and degradation [Citation23,Citation24]. We next investigated the muscle wasting/atrophy-related signalling molecules, especially Akt and atrogens (ubiquitin-ligases), in the soleus muscles. The immunohistochemistry data showed the increased staining for atrogens Atrogin-1 and MuRF1 proteins and the decreased staining for phosphorylated Akt protein in the soleus muscles of CKD mice ( for IRI model, p < 0.05, n = 8; for adenine model, p < 0.05, n = 9). LIPUS treatment could effectively and significantly reverse the changes of these signalling molecules expression in the soleus muscles of CKD mice ( for IRI model, p < 0.05, n = 8; for adenine model, p < 0.05, n = 9). The activation of Akt signal by growth factors-activated phosphoinositide 3-kinase can activate mTOR signal, which participates muscle protein synthesis, and inactivate FoxOs signal, which is involved in protein degradation [Citation23,Citation24]. A recent study showed that the skeletal muscle-specific Akt deficiency effectively induced sarcopenia in mice, which could be reversed by simultaneous activation and inhibition of mTORC1 and FoxO1, respectively [Citation25]. Therefore, our results suggest that LIPUS may alleviate CKD-induced muscle wasting by improving the regulation of muscle protein synthesis and degradation.
Figure 3. Effects of LIPUS on the immunointensity for phosphorylated Akt, Atrogin-1, and MuRF1 in soleus muscles of CKD mouse models. Two mouse models of unilateral IRI with contralateral nephrectomy (1) and adenine administration (2) were used. Immunohistochemistry for the protein expression of phosphorylated Akt, Atrogin-1, and MuRF1 was shown. The relative optical density of immunohistochemical images in five random visual fields of each section was determined by the image J 1.48 software. Data are presented as mean ± SEM (n = 8 for IRI model; n = 9 for adenine model). *p < 0.05 versus sham group; #p < 0.05 versus CKD alone group.
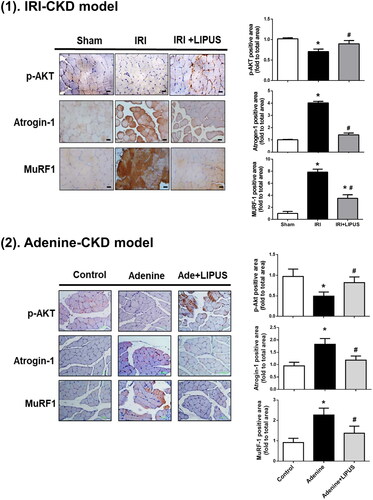
There are several factors contributing to CKD-related loss of muscle mass and strength, including physical inactivity, dysregulation of positive regulators of muscle protein turnover such as the Akt pathway, and hormonal changes, such as testosterone, vitamin D, and parathyroid hormone [Citation26]. Brightwell et al. have reported that CKD-related muscle fibrosis and maladaptation in patients can be improved by dialysis [Citation20]. Uraemic toxin-indoxyl sulphate has been found to be involved in the CKD-associated sarcopenia in a mouse model; AST‐120 (a charcoal oral absorbent) could effectively decrease the CKD-induced indoxyl sulphate levels and sarcopenia, but not the CKD-enhanced BUN/creatinine levels, suggesting that the therapeutic effect of AST-120 on sarcopenia was not attributable to improved renal function [Citation27]. Our previous study showed that serum renal function markers and indoxyl sulphate levels were markedly increased in both IRI- and adenine-CKD mice, which could be effectively prevented by LIPUS treatment [Citation12]. The present study was carried out according to our previous research conditions. We found that LIPUS application was capable of reducing the increased levels of BUN/creatinine and alleviating the decreased muscle mass, grip strength, and cross-section areas of muscle fibres in both IRI- and adenine-CKD mice. These findings suggest that LIPUS may alleviate CKD progression and CKD-induced muscle wasting.
Several non-invasive therapeutic interventions, such as neuromuscular electrical stimulation, extracorporeal shockwave therapy, and photobiomodulation, have been developed for the treatment of CKD-associated muscle wasting [Citation28–31]. LIPUS treatment has been applied to the type 1 diabetes-related skeletal muscle atrophy in rats [Citation11]. In the present study, we developed the application of LIPUS to prevent muscle wasting associated with CKD as an alternative non-invasive treatment for this disease.
Conclusion
Our findings demonstrate that a non-invasive therapeutic intervention by LIPUS protects against CKD-associated muscle wasting in mouse models. LIPUS application can help improve muscle mass loss, weak muscle strength, muscle atrophy-related protein expression, and Akt inactivation (). The detailed mechanism of LIPUS on the treatment of muscle wasting/sarcopenia and its clinical applicability still need to be further studied.
Author contribution
Conceptualisation: Chih-Kang Chiang, Kuan-Yu Hung, Shing-Hwa Liu; Formal Analysis: Chen-Yu Lin, Jui-Zhi Loh; Funding Acquisition: Chih-Kang Chiang, Kuan-Yu Hung, Shing-Hwa Liu; Investigation: Chen-Yu Lin, Te-I Weng; Methodology: Chen-Yu Lin, Te-I Weng, Ding-Cheng Chan, Chih-Kang Chiang; Supervision: Kuan-Yu Hung, Shing-Hwa Liu; Validation, Chen-Yu Lin, Chih-Kang Chiang; Writing-Original Draft: Chen-Yu Lin, Shing-Hwa Liu; Writing-Review & Editing: Chih-Kang Chiang, Kuan-Yu Hung, Shing-Hwa Liu.
Supplemental Material
Download TIFF Image (7.2 MB)Supplemental Material
Download TIFF Image (7.3 MB)Supplemental Material
Download TIFF Image (6.9 MB)Supplemental Material
Download TIFF Image (3.1 MB)Supplemental Material
Download PDF (281.3 KB)Supplemental Material
Download TIFF Image (7.5 MB)Supplemental Material
Download TIFF Image (6.2 MB)Supplemental Material
Download TIFF Image (3.3 MB)Disclosure statement
No potential conflict of interest was reported by the author(s).
Data availability statement
All data supporting the findings of this study are included in the article (and its Supplementary Information files).
Additional information
Funding
References
- Kovesdy CP. Epidemiology of chronic kidney disease: an update 2022. Kidney Int Suppl (2011). 2022;12(1):7–11.
- United States Renal Database System (USRDS). 2022. Annual Data Report, Chronic Kidney Disease: Chapter 1. https://usrds-adr.niddk.nih.gov/2022/chronic-kidney-disease/1-ckd-in-the-general-population.
- Koppe L, Fouque D, Kalantar-Zadeh K. Kidney cachexia or protein-energy wasting in chronic kidney disease: facts and numbers. J Cachexia Sarcopenia Muscle. 2019;10(3):479–484.
- Cianciaruso B, Brunori G, Kopple JD, et al. Cross-sectional comparison of malnutrition in continuous ambulatory peritoneal dialysis and hemodialysis patients. Am J Kidney Dis. 1995;26(3):475–486.
- Bataille S, Serveaux M, Carreno E, et al. The diagnosis of sarcopenia is mainly driven by muscle mass in hemodialysis patients. Clin Nutr. 2017;36(6):1654–1660.
- Kim JK, Choi SR, Choi MJ, et al. Prevalence of and factors associated with sarcopenia in elderly patients with end-stage renal disease. Clin Nutr. 2014;33(1):64–68.
- Stenvinkel P, Carrero JJ, von Walden F, et al. Muscle wasting in end-stage renal disease promulgates premature death: established, emerging and potential novel treatment strategies. Nephrol Dial Transplant. 2016;31(7):1070–1077.
- Zhang Y, Liu Y, Bi X, et al. Therapeutic approaches in mitochondrial dysfunction, inflammation, and autophagy in uremic cachexia: role of aerobic exercise. Mediators Inflamm. 2019;2019:2789014.
- Palanisamy P, Alam M, Li S, et al. Low-intensity pulsed ultrasound stimulation for bone fractures healing: a review. J Ultrasound Med. 2022;41(3):547–563.
- Ogata T, Ito K, Shindo T, et al. Low-intensity pulsed ultrasound enhances angiogenesis and ameliorates contractile dysfunction of pressure-overloaded heart in mice. PLoS One. 2017;12(9):e0185555.
- Tang L, Li N, Jian W, et al. Low-intensity pulsed ultrasound prevents muscle atrophy induced by type 1 diabetes in rats. Skelet Muscle. 2017;7(1):29.
- Lin CY, Wang CC, Loh JZ, et al. Therapeutic ultrasound halts progression of chronic kidney disease in vivo via the regulation of markers associated with renal epithelial-mesenchymal transition and senescence. IJMS. 2022;23(21):13387.
- Shu S, Zhu J, Liu Z, et al. Endoplasmic reticulum stress is activated in post-ischemic kidneys to promote chronic kidney disease. EBioMedicine. 2018;37:269–280.
- Webster AC, Nagler EV, Morton RL, et al. Chronic kidney disease. Lancet. 2017;389(10075):1238–1252.
- Chiang CK, Loh JZ, Yang TH, et al. Prevention of acute kidney injury by low intensity pulsed ultrasound via anti-inflammation and anti-apoptosis. Sci Rep. 2020;10(1):14317.
- Chiu HC, Chiu CY, Yang RS, et al. Preventing muscle wasting by osteoporosis drug alendronate in vitro and in myopathy models via sirtuin-3 down-regulation. J Cachexia Sarcopenia Muscle. 2018;9(3):585–602.
- Xin Z, Lin G, Lei H, et al. Clinical applications of low-intensity pulsed ultrasound and its potential role in urology. Transl Androl Urol. 2016;5(2):255–266.
- Mak RH, Ikizler AT, Kovesdy CP, et al. Wasting in chronic kidney disease. J Cachexia Sarcopenia Muscle. 2011;2(1):9–25.
- Tsuruta JK, Dayton PA, Gallippi CM, et al. Therapeutic ultrasound as a potential male contraceptive: power, frequency and temperature required to deplete rat testes of meiotic cells and epididymides of sperm determined using a commercially available system. Reprod Biol Endocrinol. 2012;10:7.
- Brightwell CR, Kulkarni AS, Paredes W, et al. Muscle fibrosis and maladaptation occur progressively in CKD and are rescued by dialysis. JCI Insight. 2021;6(24):e150112.
- Dong J, Dong Y, Chen Z, et al. The pathway to muscle fibrosis depends on myostatin stimulating the differentiation of fibro/adipogenic progenitor cells in chronic kidney disease. Kidney Int. 2017;91(1):119–128.
- Holecek M. Muscle wasting in animal models of severe illness. Int J Exp Pathol. 2012;93(3):157–171.
- Sirago G, Picca A, Calvani R, et al. Mammalian target of rapamycin (mTOR) signaling at the crossroad of muscle fiber fate in sarcopenia. IJMS. 2022;23(22):13823.
- Sasako T, Umehara T, Soeda K, et al. Deletion of skeletal muscle Akt1/2 causes osteosarcopenia and reduces lifespan in mice. Nat Commun. 2022;13(1):5655.
- Jaiswal N, Gavin M, Loro E, et al. AKT controls protein synthesis and oxidative metabolism via combined mTORC1 and FOXO1 signalling to govern muscle physiology. J Cachexia Sarcopenia Muscle. 2022;13(1):495–514.
- Molina P, Carrero JJ, Bover J, et al. Vitamin D, a modulator of musculoskeletal health in chronic kidney disease. J Cachexia Sarcopenia Muscle. 2017;8(5):686–701.
- Enoki Y, Watanabe H, Arake R, et al. Potential therapeutic interventions for chronic kidney disease-associated sarcopenia via indoxyl sulfate-induced mitochondrial dysfunction. J Cachexia Sarcopenia Muscle. 2017;8(5):735–747.
- Cheng TC, Huang SH, Kao CL, et al. Muscle wasting in chronic kidney disease: mechanism and clinical implications-A narrative review. IJMS. 2022;23(11):6047.
- Schardong J, Dipp T, Bozzeto CB, et al. Effects of intradialytic neuromuscular electrical stimulation on strength and muscle architecture in patients with chronic kidney failure: randomized clinical trial. Artif Organs. 2017;41(11):1049–1058.
- Schardong J, Falster M, Sisto IR, et al. Photobiomodulation therapy increases functional capacity of patients with chronic kidney failure: randomized controlled trial. Lasers Med Sci. 2021;36(1):119–129.
- Cho YS, Joo SY, Lee EK, et al. Effect of extracorporeal shock wave therapy on muscle mass and function in patients undergoing maintenance hemodialysis: a randomized controlled pilot study. Ultrasound Med Biol. 2021;47(11):3202–3210.