Abstract
Background
Curcumin has been used in the treatment of several diseases; however, its low pharmacologic profile reduces its therapeutic use. Towards improving its biological activity, nanoformulations have emerged. Thus, we aimed to determine whether curcumin nanoparticles (Cur-NPs) coated with PEG/chitosan improve the treatment of liver cancer (LC) cells and underpin the molecular mechanisms underlying their anti-cancer activity.
Methods
Cur-NPs were synthesised in the form of Cur-PLGA-PEG/chitosan NPs. The effect of Cur-NPs was assessed in HepG2 and Huh 7 LC cells and THLE-2 normal liver cells.
Results
The size of synthesised Cur-NPS was determined in the standard range of 141.2 ± 47.5 nm. Compared to THLE-2 cells, LC cells treated with Cur-NPs exerted cytotoxicity at 6.25 µg/mL after 48h. Treatment of HepG-2 cells with 2.5 µg/mL of Cur-NPs inhibited cell migration and this inhibition was augmented at 10 µg/mL (p < 0.001). Treatment of chicken embryo with 5 µg/mL Cur-NPs reduced angiogenesis (p < 0.001) of 4-day-old embryos. The nanoformulation upregulated Bax and p53 and downregulated Bcl-2 in a concentration-dependent manner and subsequently induce apoptosis in HepG-2 cells.
Conclusion
Treatment of LC cells with Cur-NPs decreased cell proliferation, migration, and angiogenesis, and induced cell death by promoting the proapoptotic pathway.
HIGHLIGHTS
Curcumin nanoparticles (Cur-NPs) increase the anticancer efficiency of Curcumin against liver cancer cells.
Cur-NPs induce apoptotic cell death of Liver cancer cells.
Cur-NPs have ant-angiogenesis and metastasis effect.
Introduction
Primary liver cancer (LC) comes six for the most diagnosed cancer among men and women [Citation1]. There are estimated new 600,000 cases diagnosed with liver cancer globally each year [Citation2]. LC is the fourth most cause of cancer-related death in both men and women, with more than 250,000 deaths each year [Citation3–5]. Although LC recurrence rates are high, surgical excision is now the most common method for treatment of the disease [Citation6]. In most cases, patients are presented with advanced tumour stages where physicians have limited treatment options to offer patients, specifically when the tumour starts to metastasise [Citation7]. Although tremendous efforts have been taken towards successful treatment of primary tumours, this success is challenged by off-site targets, development of aggressive tumours, drug resistance and relapse [Citation8]. Therefore, new strategies are needed to improve current anti-cancer agents and/or develop new targeted therapies against aggressive forms of LC. One of these current efforts is to explore the efficacy of herbal medicine using nanoformulations.
Curcumin is a diketone-yellow natural product derived from Curcuma longa Linn and it has been used for decades in traditional medicine. Curcumin has been demonstrated to have antioxidant, anti-inflammatory and anti-tumour activities in cancer cells and animal models [Citation9,Citation10]. Numerous forms of cancers have been shown to be inhibited by curcumin, which has been examined extensively in recent years [Citation11]. Along with its capacity to control cancer cell proliferation [Citation12], tumour development [Citation13], apoptosis [Citation14] as well as migration, invasion, angiogenesis, metastatic growth [Citation15], bioenergetics [Citation15], oxidative stress [Citation16], inflammation, and immune-regulating activities [Citation17–19], curcumin has been shown to have favourable impacts on health [Citation20]. The main challenge with curcumin is Curcumin’s bioavailability has been shown to be very low, with several investigations reporting amounts in blood and extraintestinal tissue that were undetectable. Because of its poor absorption, fast metabolism, chemical instability, and rapid removal from the body [Citation21].
Nanoparticles (NPs) have been used for developing traditional and FDA-approved anti-cancer agents to increase their targetable organs, stability and bioavailability, decreases therapeutic doses, and minimises off-target organs [Citation22]. It has been reported that diverse types of NP preparations are used as drug delivery vehicles and the concept of modifying their surfaces can improve the antitumor specificity and efficacy [Citation23]. Targeted co-delivery of curcumin and paclitaxel showed a promising strategy in treating breast [Citation24] and ovarian [Citation25] cancers. The use of co-delivery of curcumin with sorafenib was also reported in hepatocellular carcinoma [Citation26]. NPs prepared from FDA-approved biodegradable Poly Lactic-co-Glycolic Acid (PLGA) and loaded with different therapeutic agents have been extensively evaluated for their anti-tumour activities in a wide variety of malignancies [Citation26,Citation27]. The major limitation of using PLGA formulated NPs is the rapid clearance of NPs-loaded therapy from the blood by the process of phagocytosis. Thus, polymers with more hydrophilic properties acting as surface coating materials such polyethylene glycol (PEG) and chitosan have been used to reduce the phagocytic effect of PLGA [Citation28]. Towards this goal, NPs coated with PEG and chitosan and loaded with curcumin improved the drug release by increasing the retention time of the loaded drug, and hence increasing its therapeutic efficacy [Citation29].
Thus, the aim of this study is to evaluate the anti-tumour effect of curcumin NPs coated with PEG/chitosan in liver cancers. We used three cell lines; two liver cancer cells and one normal liver cells to determine the anti-tumour activity of curcumin NPs against liver cancer cells and the toxicity against normal cells and uncover the mechanism of cell death.
Curcumin-nanoparticles preparations
Curcumin-nanoparticles (Cur-NPs) were synthesised according to the method previously described [Citation30]. Briefly, PLGA-PEG/DSPE-PEG was used in a 99:1 ratio along with polyvinyl alcohol (PVA) and 0.05% chitosan was used as the main component of the nano formulation. The size of NPs was characterised by dynamic light scattering (DLS). The concentration of encapsulated curcumin was assayed by UV-Vis spectroscopy at λ440 nm according to a prior report [Citation30]. Chitosan served as mucoadhesive for long-residence time on the skin layers, vitamin E/Lycopene/Omega 3 fatty acids severs + hyaluronic acid (each at 1–5 mg/100–500 mg curcumin) as skin protective and skin regeneration bioactive ingredients.
Cell lines
HepG2 and Huh 7.4 liver cancer cell lines and THLE2 (normal liver) were obtained from the American Tissue Culture Collection (ATCC). All cell lines were cultured in RPMI-1640 medium supplemented with 10% inactivate Foetal Bovine Serum (FBS) and 1% penicillin/streptomycin and maintained in a humidified 5% CO2 incubator.
Cell viability
Cell cytotoxicity was assessed using the MTT assay as described [Citation31]. Briefly, cells (5x103/well) were seeded into 96-well plates and treated with different concentrations (0- 200 µg/ml) of curcumin NPs for 24 and 48h time points. Tetrazolium salt 10-μl (5 mg/ml) were added to each well and incubated at 37 °C for 3 - 4h and the absorbance was read at 570 nM using an ELISA microplate reader (Molecular Devices, Downingtown, PA, USA). For each cell line, the 50% inhibition concentration (IC50) of curcumin NPs was calculated according to Reed-Muench method [Citation32].
Cell apoptosis by flowcytometry
Apoptotic and necrotic cell death was confirmed using propidium Iodide and annexin-V method. Briefly, cells were plated in 10 cm3 dishes and treated with the effective doses (0, 6.25, 12.5, and 25 5 mg/mL) of curcumin NPs. After 24 h, cells were harvested, and the resulting pellets were fixed in ice-cold 70% ethanol. Those cells were centrifuged, washed, and re-suspended in PBS solution containing RNase A (1 mg/ml), and incubated for 30 min at 37 °C. Annexin-V/propidium iodide (PI) solution at 1.0 mg/mL concentration was added. Analysis of the stained cells was performed using a fluorescence-activated cell sorter (FACS) following the manufacturer’s instructions.
Gene expression analysis
Treated and untreated LC cells will be trypsinized and collected on ice. Total RNA was extracted using RNeasy kit (Qiagen; Germantown, MD). cDNA was synthesised using M-MuLV RT and random primer mix (New England Biolabs, Ipswich, MA). Quantitative Real-Time PCR (qPCR) was performed using SYBR Green master-mix (Bio-Rad, Hercules, CA). The fold change calculated regarding GAPDH. The sequence of primers for Bax was F: 5′‐CCCGAGAGGGTCTTTTTCCGAG‐3′ and R: 5′‐CCAGCCCATGATGGTTCTGAT‐3′, Bcl-2 F: 5′- TCAGAGCTTTGAGCAGGTAG‐3′ and R: 5′‐AAGGGCTCTAGGTCATTC‐3′, Tp53 F: 5′- TGACTGTACCACCATCCACTA‐3′ and R: 5′‐AAACACGCACCTCAAAGC‐3′, and GAPDH F: 5′-TGTCCGTCGTGGATCTGAC‐3′ and R: 5′‐ CCTGCTTCACCACCTTCTTG ‐3′.
Wound-healing assay
The effect of curcumin NPs on HepG2 cell migration was assessed using the wound healing assay as described [Citation33]. In brief, wound was created by making a scratch per each well of 6-well plate when cell confluency reached 80–90% using a pipette tip. Cells were gently washed with PBS and treated with 0, 6.25, 12.5, and 25 µg/ml of curcumin NPs. Images were acquired from at least 5 areas and then analysed by ImageJ software (NIH, Bethesda, MD, USA).
Blood vessel formation using a chick embryo model
The effect of curcumin NPs on blood vessels formation was assessed using chick embryo model [Citation34]. Briefly, fertilised eggs of the chick Gallus domesticus obtained from the farm of Faculty of Agriculture, Assiut University, were used in the study. All embryological materials needed for the experiments were obtained by artificial incubation using an electrical thermostatically controlled incubator. Both the trays of the eggs and inside of the incubator were thoroughly cleaned. Labelled fertile eggs were placed vertically in the trays inside the incubator for 4 days after treatment with curcumin NPs in addition to PBS as a control group. A small window of about 1 cm2 was made in the eggshell. Curcumin NPs were placed, and the windows were sealed with a tape to the end of the experiment [Citation34]. By the end of each time point, images were acquired.
Animals and treatment
Six to eight weeks adult female Swiss albino mice (n = 20) weighing 20–25 g were kept at the animal house unit of the King Fahd Medical Research Centre, King Abdulaziz University (KAU), Jeddah, Saudi Arabia. Before initiating preclinical mouse model, the experimental protocol received an official approval from the Ethical Committee at KAU University. Once mice received, mice were acclimatised for one week with the new surrounding fed commercial diet and allows access to water ad libitum. Ehrlich solid carcinoma (ESC) was induced in mice using Ehrlich Ascites Carcinoma (EAC) cells purchased from the National Cancer Institute (NCI) of Egypt, Cairo, Egypt. The viability of ESC cells was measured using a trypan blue exclusion assay. About 2.5 × 106 viable EAC cells resuspended in 0.2 ml PBS/mouse were injected intramuscularly in the left thigh of each mouse to induce a solid tumour following the standard protocol [Citation35]. Tumour growth was reported in 100% of the mice, with a palpable solid tumour mass within 10 days of implantation. The mice bearing tumours were randomly divided into 3 groups; 5 mice each, in addition to one group of mice with non-tumour which served as a control healthy group. The tumour groups were designed as follows; tumour group, tumour + cisplatin (3.5 mg/kg), and tumour + cisplatin + Cur-NPs (3 mg/kg). The selected doses of cisplatin and Cur-NPs were intraperitoneally administered on a daily base for 2 weeks as previously reported [Citation36]. The tumour group was injected with phosphate buffer saline (PBS) solution and kept as a second control.
Statistical analysis
Data were represented as mean ± stand error of the mean (SEM). Data statistically evaluated using the two-way analysis of variance (ANOVA) method for comparing multiple groups, followed by Dunnett’s post-hoc multiple comparison test to evaluate differences with control counterparts. Correlations between the protein expression in mice tumour tissues with and without NP treatments was analysed with a two-tailed Spearman rank correlation (Rho) using GraphPad Prism 6.0 (Intuitive Software for Science, San Diego, CA, USA). The value of p < 0.05 was considered statistically significant.
Results
Characterisation of curcumin-nanoparticles
Curcumin nanoparticles (Cur-NPs) were prepared by double emulsion method in which 2 g of poly lactic-co-glycolic acid (PLGA)/chitosan were mixed with 100 mg curcumin in DMSO as depicted in . Once prepared, the spectra of Cur-NP and non-capsulated curcumin (Cur), used as a standard. Were measured and the size of nanoparticles were characterised by DLS (). The resulted size of curcumin nano formulation was 139.3 ± 55.16 nm in diameter.
Figure 1. Preparation and characterisation of curcumin nanoparticles. (A) Schematic representation shows the preparation of curcumin nanoparticles (Cur-NPs). (B) Comparison of UV-Visible spectra for the nanoparticles and non-encapsulated curcumin. (C) Size measurement of Cur-NPs using dynamic light scattering (DLS).
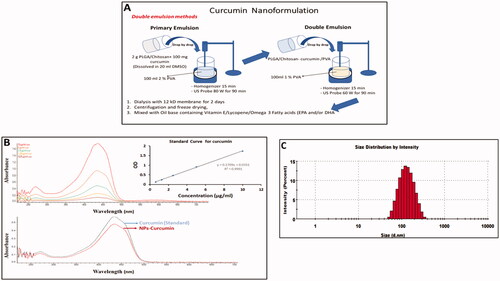
The effect of different concentrations of curcumin-nanoparticles on cell proliferation
To determine the effect of Cur-NPs on inducing cell toxicity, cytotoxicity assay was performed on two liver cancer HepG2 and Huh 7 cells in addition to THLE2 normal liver cells. Cells were treated with different concentrations of Cur-NPs for 24h as shown in and 48h as shown in and curcumin for 48h as in . Initially, treatment of cancer cells with Cur-NPs showed an early cytotoxic effect after 24h and reached the maximum effect after 48h. The IC50 of HepG2 and Huh 7 was 7.42 and 11.27 µg/ml, respectively compared to normal THLE-2 (IC50 > 100 µg/ml) cells after 48h of treatment and significantly lower than normal curcumin which show IC50 of 90 µg/ml.
Figure 2. The effect of different concentrations of curcumin nanoparticles on cell proliferation of liver cancer Huh7 and HepG2 cells and THLE-2 as a control normal liver cell. Cells were treated with the indicated concentrations of the curcumin nanoparticles for (A) 24h and (B) 48h and curcumin (C) 48h, respectively, and cell proliferation was assessed by MTT assay in triplicates.
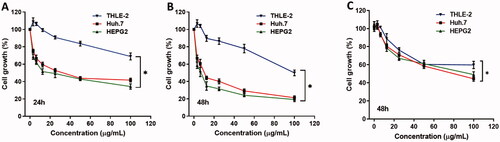
The effect of curcumin-nanoparticles on the cell migration using wound healing assay
Treatment of HepG2 cells for 48h with Cur-NPs (2.5, 5 and 10 µg/ml) significantly suppressed cell migration compared to vehicle control cells (). The suppression of migratory cells was shown at low concentration of Cur-NPs at 2.5 µg/ml and reached the maximum inhibition at 10 µg/ml after 48h of treatment (p < 0.0001) for the tested cells as shown in .
Figure 3. The effect of curcumin nanoparticles on cell migration using wound-healing assay. HepG-2 cells were treated with Cur-NPs for 24, and 48 h. Wounds were visualised using a converted microscope and the scale bar is 100 µm and images were analysed with ImageJ software. Data considered statistically significant at p < 0.05 regarding control nontreated cells and treatment after 24 and 48 h. All data are represented as mean ± SD from three independent experiments and analysed with two-way ANOVA, Data considered statistically significant at (***p ≤ 0.001) and (****p ≤ 0.0001).
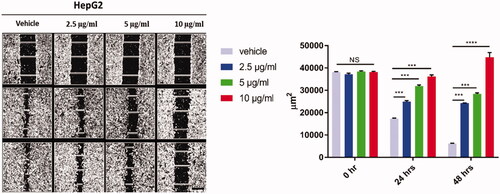
The effect of curcumin-nanoparticles on blood vessel formation of chick embryo
The effect of Cur-NP on the blood vessel formation using chick embryo was examined after 4 days using one medium concentration of 5 µg/ml. The results indicate that treatment of fertilised eggs with Cur-NPs inhibited the blood vessel formation after 2, 3 and 4 days of Cur-NPs application as compared to control embryos ().
Effect of curcumin-nanoparticles on inducing apoptotic cell death
The effect of treatment of Cur-NPs on apoptosis-related gene expression in HepG-2 and Huh 7 cells was determined by quantitative Real-Time PC analysis. As shown in , Cur-NPs induced a significant upregulation both of Bax mRNA expression by 3, 8 and 12 folds and p53 by 3, 6, and 10 folds after 48h of treatment with 6.25, 12.5 and 25 µg/ml, respectively. On the other hand, BCL-2 was down-regulated by 0.6, 0.4, and 0.25 folds under the same treatment conditions in HepG2 cells. In huh-7, not only Bax mRNA expression was upregulated roughly by 2, 17 and 21 folds but also p53 expression was increased by 2, 7.5, and 9 folds after 48h of treatment with 6.25, 12.5 and 25 µg/ml, respectively. BCL-2 was significantly down regulated at 12.5 and 25 µg/ml.
Figure 5. Effect of curcumin nanoparticles on gene and protein expressions. HepG-2 and HuH7cells were treated with Cur-NPs at the indicated concentrations for 48 h. (A–C) BAX, P53, and BCL-2 mRNA fold change in HepG2 cells, respectively and (D–F): BAX, P53, and BCL-2 mRNA fold change in Huh 7 cells, respectively. Data considered statistically significant at p < 0.05. Significance was calculated regarding cells treated 6.25 (a), 12.5 (b) and 25 µg/ml (c) of curcumin NPs compared to cells received DMSO. Experiments were conducted in triplicates and repeated at least twice.
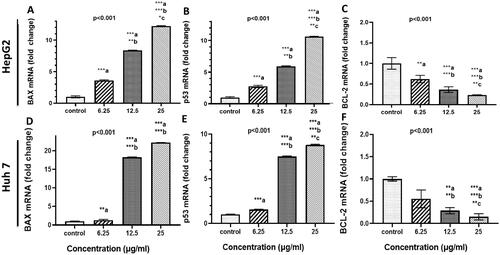
In consistent the gene expression data, Flowcytometric analysis significantly showed treatment of HepG2 cells with 6.25, 12.5, and 25 µg/ml of Cur-NPs for 48 h developed 72.7, 100, and 100% apoptotic cell death without any necroptotic cell death signal as depicted in and evidenced by bright filed images.
Figure 6. Effect of curcumin nanoparticles on cell apoptosis. Upper panel (A): bright field images showing the effect of different concentrations of cur-NPs (6.25, 12.5 and 25 µg/mL) on HepG2 cells. Lower panel (B): Apoptotic and necrotic cell death of HepG2 were assessed using Annexin V and 7AAD assay after 48 h treatment with curcumin NPs.
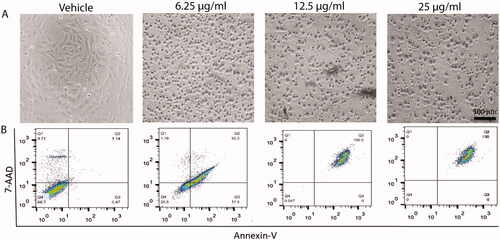
Effect of curcumin-nanoparticles on mice induced with Ehrlich ascites carcinoma
To validate our in vitro findings, we recapitulated the effect of Cur-NPs treatment using mouse engrafted Ehrlich solid carcinoma (ESC) model. Haematoxylin and eosin (H&E) staining was performed on formalin-fixed paraffin-embedded (FFPE) liver tissues collected from four animal groups; healthy mice received vehicle, mice with ESC, mice with ESC received cisplatin and mice with ESC received cisplatin and Cur-NPs. As illustrated in , Control mice only received vehicle demonstrates normal central vein (CV) and portal vein (PV) regions with normal hepatocytes characterised by rounded vesicular nuclei (black arrows) and separated by thin blood sinusoids (white arrows). In mice with Ehrlich carcinoma, they show a dilation of liver vessels (CV and PV), marked infiltration of hepatic sinusoids with malignant Ehrlich cells (thick black arrows), and hepatocytes showed large nuclei (dotted arrow) compared to control mice. In animals with Ehrlich carcinoma and received cisplatin, degenerated neoplastic cells with dark nuclei (thick black arrows) were identified. Hepatocytes showed large size nuclei (karyomegaly; dotted thin arrows). Other features looked shrined with small dark degenerated nuclei (thin black arrows). Blood sinusoids were dilated with few inflammatory cells (white arrows). Mice with Ehrlich carcinoma treated with cisplatin and Cur-NPs demonstrated normal hepatocytes with normal size and nuclear appearance. Hepatic blood sinusoids looked normal and not dilated (white arrows). Tumour cells still showed degrative changes (thick black arrows).
Figure 7. Anti-tumour activity of curcumin nanoparticles in mice engrafted Ehrlich solid carcinoma. Histological sections from liver stained with H&E and photographed at 100 with scale bar 200 µm and and 400X with scale bar 50 µm. Control group (A) shows normal central vein (CV) and portal vein (PV) regions with normal hepatocytes having rounded vesicular nuclei (black arrows) and separated by thin blood sinusoids (white arrows). Solid Ehrlich tumour group (B) shows a dilation of liver vessels (CV& PV), marked infiltration of hepatic sinusoids with malignant Ehrlich cells (thick black arrows), and hepatocytes showed large nuclei (dotted arrow). Solid Ehrlich tumour + Cis group (C) shows degenerated neoplastic cells with dark nuclei (thick black arrows). Hepatocytes showed large size nuclei or karyomegaly (dotted thin arrows). Others looked shrined with small dark degenerated nuclei (thin black arrow). Blood sinusoids are dilated with few inflammatory cells (white arrows). Solid Ehrlich tumour + Cis + Cur-NPs group(D) shows nearly normal hepatocyte with normal size and nuclear appearance. Hepatic blood sinusoids looked normal and not dilated (white arrows). Tumor cells still showed degrative changes (thick black arrows).
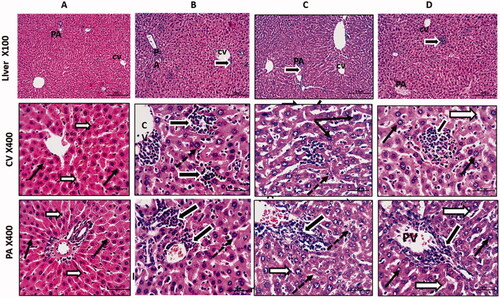
Discussion
Although non-formulated curcumin has shown anti-tumour effects in different cancer types, its therapeutic efficacy is compromised by its low water solubility, chemical instability and bioavailability [Citation37]. Therefore, nanoparticles (NPs) loaded with curcumin have been developed to improve its bioavailability and therapeutic efficacy [Citation38]. For example, poly lactic-co-glycolic acid (PLGA) nanoparticles loaded with curcumin have shown an improved cellular uptake and bioavailability of curcumin using tissue culture and animal models [Citation30]. Other polymers have been used for synthesis of nanoparticles loaded with curcumin such as N-isopropylacrylamide, polyvinyl alcohol, PLGA, polyethylene glycol monoacrylate, fibroin, and chitosan [Citation39]. The current study aimed to evaluate whether the efficacy of curcumin PLGA nanoparticles coated with PEG/chitosan will improve the anti-neoplastic activity against LC cells using in vitro and in vivo Ehrlich carcinoma mouse model.
Prior studies reported that curcumin release from PLGA-formulated nanoparticles was less than 75% and this decrease was attributed to high molecular weight of PLGA [Citation40,Citation41]. Thus, surface modification of NPs with various macromolecules, such as chitosan and polyethylene glycol (PEG) exhibited a significant improvement in the bioavailability of curcumin [Citation42]. Other types of nanoformulations have been developed such as poly-glycerol-malic acid-dodecanedioic acid)/curcumin nanoparticles (PGMD) which are used for evaluating their anti-neoplastic activity against MDA-MB-231 and MCF7 cells [Citation31].
Our results demonstrate that the average size of the prepared Cur-NPs was 139 nm in diameter which is comparable with other previous studies [Citation41]. When the effect of Cur-NPs was assessed on liver cancer cells, Cur-NPs has shown to induce significant cytotoxicity in HepG2 and Huh7 cells at 6.25 µg/ml after 48h of treatment, compared to vehicle controls. In a study conducted on pancreatic cancer cells treated with PLGA and coated with PEG and chitosan, a significant increase of cell toxicity was identified compared to non-formulated curcumin [Citation42]. Comparable to our results, dimethyl curcumin encapsulated PLGA nanoparticles inhibit growth rate of breast cancer cells [Citation43]. PEG polylactic acid nanoparticles showed a cytotoxic effect on MDA-MB-231 and HeLa cells [Citation44]. Concerning HepG2 cells, treatment of these cells with chitosan-coated poly (butyl) cyanoacrylate NPs induced cell apoptosis when compared to non-formulated curcumin [Citation45].
It has been known that primary cancer cells metastasise to distant organs through degradation of extracellular matrix and promoting cell migration, invasion, and angiogenesis [Citation46]. Different approaches, including nanoformulation, have been undertaken to inhibit cancer metastasis and recurrence. Our results show that treatment of LC with 2.5 to 10 µg/ml of Cur-NPs inhibited cell migration and angiogenesis as evidenced by the results from wound-healing assay and chicken embryo model. Arya et al. reported comparable results of anti-migratory and anti-invasive property when pancreatic cells were treated with PLGA chitosan/PEG curcumin NPs compared to cells treated with native curcumin [Citation42]. The chick embryo and its chorioallantois membrane have been used as an accepted model although its reported limitations for evaluating drug delivery loaded in nanoparticles [Citation47,Citation48]. An investigation performed by de Carvalho et al. examined the effect of curcumin-loaded nanoformulations and their different coating agents using a chick embryo model. They found that curcumin NPs coated with P80, PEG, EUD and CS reduce angiogenic and teratogenic effects in the treated chick embryos [Citation49]. In addition, a study conducted by Dragostin et al. revealed that treatment of chick embryo with chitosan-sulfadimethoxine (CLC) and chitosan-sulfisoxazole (CLD) NPs exerted an antiangiogenic effect [Citation50].
At the molecular level, our results show that Cur-NPs upregulated Bax and p53 whereas Bcl-2 was downregulated in a concentration-dependent manner. Another mounted data showed Cur-NPs induced cell apoptosis in HepG2 cells. Experimental studies revealed curcumin-PLGA NPs decreases the activity of multiple drug resistance protein 1 and increases reactive oxygen species (ROS) in cisplatin-resistant oral cancer cells through the activation of Caspases 3 and 9 pathways [Citation51]. In another study, curcumin-loaded liposomes nanoparticles (CLNP) induced cytotoxic activity in B16BL6 melanoma cells and inhibits the PI3K/AKT pathway [Citation52]. Anand et al. demonstrate curcumin NPs inhibit cell proliferation, invasion and angiogenesis by decreasing cyclin D, MMP-9, and VEGF protein expressions [Citation30].
Interestingly, curcumin NPs improved deteriorated histological features in liver of mice as a result of induction of Ehrlich solid carcinoma when compared to mice injected with Ehrlich carcinoma cells and received cisplatin. El-Gizawy et al. reported the hepatoprotective and nephroprotective effects of curcumin NPs in rats received intrapretonial12 mg/kg bw cisplatin for two weeks [Citation53]. An investigation conducted by Harakeh and co-authors, mice with Ehrlich carcinoma and received pomegranate PE NPs showed a significant decrease of cisplatin-induced oxidative stress in renal tissues by promoting antioxidative and anti-inflammatory mechanisms [Citation54]. The potential effect of curcumin NPs on liver tissues is a result of inducing multiple orchestrated mechanisms to reduce the deleterious effects of Ehrlich carcinoma and cisplatin on different organs including liver using rodent models [Citation53–55].
Conclusions
Although native curcumin has shown anti-neoplastic effects in liver cancer cells. Curcumin-loaded PLGA NPs inhibited cell proliferation and showed anti-migratory and anti-angiogenic property as compared to native curcumin. These NPs also induced cell apoptosis via upregulation of Bax and p53 and downregulation of Bcl-2. Moreover, there is an improvement in histological features of liver tissues of mice injected with Ehrlich solid carcinoma and received anticancer effect by inhibiting multiple signalling pathways in liver cancer cells, it has limitations including low resolve bioavailability. To this problem, PLGA nanoparticles have been used and showed a promise in treating different types of cancers by enhancing drug bioavailability. Coating of PLGA with PEG/chitosan further improved drug efficacy and increased a treatment with cisplatin and curcumin NPs.
Authors’ contributions
S. Mousa S. Harakeh, SH. Sabre, and ZY. Abd Elmageed; designed and guide the experiments. SH. Sabre, and ZY. Abd Elmageed; did the lab work. T. alamri, R Al-Raddadi, S. Al-Jaouni, H. M. Qari, M. Moulay, and S.Haque: Data visualisation and analysis, wrote the manuscript. All authors agree to the final version of the manuscript.
Consent for publication
All authors have provided consent for the manuscript to be published.
Disclosure statement
The authors declare that they have no known competing financial interests or personal relationships that could have appeared to influence the work reported in this paper.
Data availability statement
The data sets used and/or analysed during this study are available from the corresponding author on reasonable request.
Additional information
Funding
References
- Bray F, Ferlay J, Soerjomataram I, et al. Global cancer statistics 2018: GLOBOCAN estimates of incidence and mortality worldwide for 36 cancers in 185 countries. CA Cancer J Clin. 2018;68(6):394–424. doi: 10.3322/caac.21492.
- Huo T-I, Lui W-Y, Wu J-C, et al. Deterioration of hepatic functional reserve in patients with hepatocellular carcinoma after resection: incidence, risk factors, and association with intrahepatic tumor recurrence. World J Surg. 2004;28(3):258–262. doi: 10.1007/s00268-003-7182-6.
- Bruix J, Sherman M; Practice Guidelines Committee, American Association for the Study of Liver Diseases. Management of hepatocellular carcinoma. Hepatology. 2005;42(5):1208–1236. doi: 10.1002/hep.20933.
- Parkin DM, Bray F, Ferlay J, et al. Global cancer statistics, 2002. CA Cancer J Clin. 2005;55(2):74–108. doi: 10.3322/canjclin.55.2.74.
- Hu B, Sun D, Sun C, et al. A polymeric nanoparticle formulation of curcumin in combination with sorafenib synergistically inhibits tumor growth and metastasis in an orthotopic model of human hepatocellular carcinoma. Biochem Biophys Res Commun. 2015;468(4):525–532. doi: 10.1016/j.bbrc.2015.10.031.
- Visvader JE. Cells of origin in cancer. Nature. 2011;469(7330):314–322. doi: 10.1038/nature09781.
- Spano D, Heck C, De Antonellis P, et al. Molecular networks that regulate cancer metastasis. Semin Cancer Biol. 2012;22(3):234–249. doi: 10.1016/j.semcancer.2012.03.006.
- Anderson RL, Balasas T, Callaghan J, et al. A framework for the development of effective anti-metastatic agents. Nat Rev Clin Oncol. 2019;16(3):185–204. doi: 10.1038/s41571-018-0134-8.
- Wilken R, Veena MS, Wang MB, et al. Curcumin: a review of anti-cancer properties and therapeutic activity in head and neck squamous cell carcinoma. Mol Cancer. 2011;10:12. doi: 10.1186/1476-4598-10-12.
- Sharifi-Rad J, Rayess YE, Rizk AA, et al. Turmeric and its major compound curcumin on health: bioactive effects and safety profiles for food, pharmaceutical, biotechnological and medicinal applications. Front Pharmacol. 2020;11:01021. doi: 10.3389/fphar.2020.01021.
- Tian S, Liao L, Zhou Q, et al. Curcumin inhibits the growth of liver cancer by impairing myeloid‑derived suppressor cells in murine tumor tissues. Oncol Lett. 2021;21(4):286. doi: 10.3892/ol.2021.12547.
- Banerjee S, Ji C, Mayfield JE, et al. Ancient drug curcumin impedes 26S proteasome activity by direct inhibition of dual-specificity tyrosine-regulated kinase 2. Proc Natl Acad Sci U S A. 2018;115(32):8155–8160. doi: 10.1073/pnas.1806797115.
- Liu Y, Wang X, Zeng S, et al. The natural polyphenol curcumin induces apoptosis by suppressing STAT3 signaling in esophageal squamous cell carcinoma. J Exp Clin Cancer Res. 2018;37(1):1–12. doi: 10.1186/s13046-018-0959-0.
- Zhang P, Lai Z-L, Chen H-F, et al. Curcumin synergizes with 5-fluorouracil by impairing AMPK/ULK1-dependent autophagy, AKT activity and enhancing apoptosis in Colon cancer cells with tumor growth inhibition in xenograft mice. J Exp Clin Cancer Res. 2017;36(1):1–12. doi: 10.1186/s13046-017-0661-7.
- Wang L, Chen X, Du Z, et al. Curcumin suppresses gastric tumor cell growth via ROS-mediated DNA polymerase γ depletion disrupting cellular bioenergetics. J Exp Clin Cancer Res. 2017;36(1):1–14. doi: 10.1186/s13046-017-0513-5.
- Rana M, Maurya P, Reddy SS, et al. A standardized chemically modified curcuma longa extract modulates IRAK-MAPK signaling in inflammation and potentiates cytotoxicity. Front Pharmacol. 2016;7:223. doi: 10.3389/fphar.2016.00223.
- Illuri R, Bethapudi B, Anandakumar S, et al. Anti-inflammatory activity of polysaccharide fraction of curcuma longa extract (NR-INF-02). Antiinflamm Antiallergy Agents Med Chem. 2015;14(1):53–62. doi: 10.2174/1871523014666150407150533.
- Kocaadam B, Şanlier N. Curcumin, an active component of turmeric (curcuma longa), and its effects on health. Crit Rev Food Sci Nutr. 2017;57(13):2889–2895. doi: 10.1080/10408398.2015.1077195.
- S, Darvesh A, B, Aggarwal B, Bishayee A. Curcumin and liver cancer: a review. Curr Pharm Biotechnol. 2012;13(1):218–228. doi: 10.2174/138920112798868791.
- Uchio R, Higashi Y, Kohama Y, et al. A hot water extract of turmeric (curcuma longa) suppresses acute ethanol-induced liver injury in mice by inhibiting hepatic oxidative stress and inflammatory cytokine production. J Nutr Sci. 2017;6:e3. doi: 10.1017/jns.2016.43.
- Anand P, Kunnumakkara AB, Newman RA, et al. Bioavailability of curcumin: problems and promises. Mol Pharm. 2007;4(6):807–818. doi: 10.1021/mp700113r.
- Kemp JA, Kwon YJ. Cancer nanotechnology: current status and perspectives. Nano Converg. 2021;8(1):34.
- Nakamura Y, Mochida A, Choyke PL, et al. Nanodrug delivery: is the enhanced permeability and retention effect sufficient for curing cancer? Bioconjug Chem. 2016;27(10):2225–2238. doi: 10.1021/acs.bioconjchem.6b00437.
- Baek JS, Cho CW. A multifunctional lipid nanoparticle for co-delivery of paclitaxel and curcumin for targeted delivery and enhanced cytotoxicity in multidrug resistant breast cancer cells. Oncotarget. 2017;8(18):30369–30382. doi: 10.18632/oncotarget.16153.
- Zhao MD, Li JQ, Chen FY, et al. Co-Delivery of curcumin and paclitaxel by "Core-Shell" targeting amphiphilic copolymer to reverse resistance in the treatment of ovarian cancer. Int J Nanomed. 2019;14:9453–9467. doi: 10.2147/IJN.S224579.
- Bian Y, Guo D. Targeted therapy for hepatocellular carcinoma: co-Delivery of sorafenib and curcumin using lactosylated pH-Responsive nanoparticles. Drug Des Devel Ther. 2020;14:647–659. doi: 10.2147/DDDT.S238955.
- Verderio P, Bonetti P, Colombo M, et al. Intracellular drug release from curcumin-loaded PLGA nanoparticles induces G2/M block in breast cancer cells. Biomacromolecules. 2013;14(3):672–682. doi: 10.1021/bm3017324.
- Hu FQ, Meng P, Dai YQ, et al. PEGylated chitosan-based polymer micelle as an intracellular delivery carrier for anti-tumor targeting therapy. Eur J Pharm Biopharm. 2008;70(3):749–757. doi: 10.1016/j.ejpb.2008.06.015.
- Parveen S, Sahoo SK. Long circulating chitosan/PEG blended PLGA nanoparticle for tumor drug delivery. Eur J Pharmacol. 2011;670(2–3):372–383. doi: 10.1016/j.ejphar.2011.09.023.
- Anand P, Nair HB, Sung B, et al. Design of curcumin-loaded PLGA nanoparticles formulation with enhanced cellular uptake, and increased bioactivity in vitro and superior bioavailability in vivo. Biochem Pharmacol. 2010;79(3):330–338. doi: 10.1016/j.bcp.2009.09.003.
- Kumari M, Sharma N, Manchanda R, et al. PGMD/curcumin nanoparticles for the treatment of breast cancer. Sci Rep. 2021;11(1):3824. doi: 10.1038/s41598-021-81701-x.
- Saganuwan SA. A modified arithmetical method of reed and muench for determination of a relatively ideal median lethal dose. Afr J Pharm Pharmacol. 2011;5(12):1543–1546. doi: 10.5897/AJPP11.393.
- Gaballa R, Ali HEA, Mahmoud MO, et al. Exosomes-Mediated transfer of Itga2 promotes migration and invasion of prostate cancer cells by inducing Epithelial-Mesenchymal transition. Cancers (Basel). 2020;12(8):2300. doi: 10.3390/cancers12082300.
- Lokman NA, Elder AS, Ricciardelli C, et al. Chick chorioallantoic membrane (CAM) assay as an in vivo model to study the effect of newly identified molecules on ovarian cancer invasion and metastasis. Int J Mol Sci. 2012;13(8):9959–9970. doi: 10.3390/ijms13089959.
- Aldubayan MA, Elgharabawy RM, Ahmed AS, et al. Antineoplastic activity and curative role of avenanthramides against the growth of ehrlich solid tumors in mice. Oxid Med Cell Longev. 2019;2019:5162687. doi: 10.1155/2019/5162687.
- El-Shitany NA, Abbas AT, Ali SS, et al. Nanoparticles ellagic acid protects against cisplatin-induced hepatotoxicity in rats without inhibiting its cytotoxic activity. Int J Pharmacol. 2019;15(4):465–477. doi: 10.3923/ijp.2019.465.477.
- Dei Cas M, Ghidoni R. Dietary curcumin: correlation between bioavailability and health potential. Nutrients. 2019;11(9):2147. doi: 10.3390/nu11092147.
- Carolina Alves R, Perosa Fernandes R, Fonseca-Santos B, et al. A critical review of the properties and analytical methods for the determination of curcumin in biological and pharmaceutical matrices. Crit Rev Anal Chem. 2019;49(2):138–149. doi: 10.1080/10408347.2018.1489216.
- Shome S, Talukdar AD, Choudhury MD, et al. Curcumin as potential therapeutic natural product: a nanobiotechnological perspective. J Pharm Pharmacol. 2016;68(12):1481–1500. doi: 10.1111/jphp.12611.
- Khalil NM, do Nascimento TC, Casa DM, et al. Pharmacokinetics of curcumin-loaded PLGA and PLGA-PEG blend nanoparticles after oral administration in rats. Coll Surf B Biointer. 2013;101:353–360. doi: 10.1016/j.colsurfb.2012.06.024.
- Sharma A, Hawthorne S, Jha SK, et al. Effects of curcumin-loaded poly(lactic-co-glycolic acid) nanoparticles in MDA-MB231 human breast cancer cells. Nanomedicine (Lond). 2021;16(20):1763–1773. doi: 10.2217/nnm-2021-0066.
- Arya G, Das M, Sahoo SK. Evaluation of curcumin loaded chitosan/PEG blended PLGA nanoparticles for effective treatment of pancreatic cancer. Biomed Pharmacother. 2018;102:555–566. doi: 10.1016/j.biopha.2018.03.101.
- Verderio P, Pandolfi L, Mazzucchelli S, et al. Antiproliferative effect of ASC-J9 delivered by PLGA nanoparticles against estrogen-dependent breast cancer cells. Mol Pharm. 2014;11(8):2864–2875. doi: 10.1021/mp500222k.
- Liang H, Friedman JM, Nacharaju P. Fabrication of biodegradable PEG-PLA nanospheres for solubility, stabilization, and delivery of curcumin. Artif Cells Nanomed Biotechnol. 2017;45(2):297–304. doi: 10.3109/21691401.2016.1146736.
- Duan J, Zhang Y, Han S, et al. Synthesis and in vitro/in vivo anti-cancer evaluation of curcumin-loaded chitosan/poly(butyl cyanoacrylate) nanoparticles. Int J Pharm. 2010;400(1–2):211–220. doi: 10.1016/j.ijpharm.2010.08.033.
- Guan X. Cancer metastases: challenges and opportunities. Acta Pharm Sin B. 2015;5(5):402–418. doi: 10.1016/j.apsb.2015.07.005.
- Vargas A, Zeisser-Labouebe M, Lange N, et al. The chick embryo and its chorioallantoic membrane (CAM) for the in vivo evaluation of drug delivery systems. Adv Drug Deliv Rev. 2007;59(11):1162–1176. doi: 10.1016/j.addr.2007.04.019.
- Nowak-Sliwinska P, Segura T, Iruela-Arispe ML. The chicken chorioallantoic membrane model in biology, medicine and bioengineering. Angiogenesis. 2014;17(4):779–804. doi: 10.1007/s10456-014-9440-7.
- de Carvalho FB, de Gomes MG, Savall ASP, et al. Evaluation of curcumin-loaded polymeric nanocapsules with different coatings in chick embryo model: influence on angiogenesis, teratogenesis and oxidative stress. Pharmacol Rep. 2021;73(2):563–573. doi: 10.1007/s43440-021-00218-2.
- Dragostin OM, Tatia R, Samal SK, et al. Designing of chitosan derivatives nanoparticles with antiangiogenic effect for cancer therapy. Nanomaterials (Basel). 2020;10(4:698). doi: 10.3390/nano10040698.
- Chang PY, Peng SF, Lee CY, et al. Curcumin-loaded nanoparticles induce apoptotic cell death through regulation of the function of MDR1 and reactive oxygen species in cisplatin-resistant CAR human oral cancer cells. Int J Oncol. 2013;43(4):1141–1150. doi: 10.3892/ijo.2013.2050.
- Chen Y, Wu Q, Zhang Z, et al. Preparation of curcumin-loaded liposomes and evaluation of their skin permeation and pharmacodynamics. Molecules. 2012;17(5):5972–5987. doi: 10.3390/molecules17055972.
- El-Gizawy MM, Hosny EN, Mourad HH, et al. Curcumin nanoparticles ameliorate hepatotoxicity and nephrotoxicity induced by cisplatin in rats. Naunyn Schmiedebergs Arch Pharmacol. 2020;393(10):1941–1953. doi: 10.1007/s00210-020-01888-0.
- Harakeh S, Almuhayawi MS, Akefe IO, et al. Novel pomegranate-nanoparticles ameliorate cisplatin-induced nephrotoxicity and improves cisplatin anti-cancer efficacy in ehrlich carcinoma mice model. Molecules. 2022;27(5:1605). doi: 10.3390/molecules27051605.
- Alotaibi B, Tousson E, El-Masry TA, et al. Ehrlich ascites carcinoma as model for studying the cardiac protective effects of curcumin nanoparticles against cardiac damage in female mice. Environ Toxicol. 2020;63(1):105-113. doi: 10.1002/tox.23016.