Abstract
In order to load metformin in a nano formula and evaluate the produced nano form towards cancer cells, metformin was loaded on natural carrier coconut oil. The formed metformin-loaded coconut oil nanoemulsion was characterized by Zeta potential, particle size, drug content, drug release, and drug stability. The formed nanoemulsion was evaluated towards MCF-7, HepG2, and HCT-116 cell lines. Cell cycle analysis and apoptosis mechanism were studied. The nanoemulsion was created using deionized water, 1.5% Span 20, 1.5% Tween 80, 1.5% coconut oil, and 0.5% Metformin in an ultrasonicator to produce a homogenous solution. The anticancer activities of the metformin-loaded coconut nanoemulsion were highly improved compared to non-formulated metformin with IC50s of 8.3 ± 0.1 µg/ml, 12 ± 1.5 µg/ml, 2.685 ± 0.3 µg/ml for MCF-7, HepG2, and HCT-116 cell lines, respectively. There was a 76.5 ± 2.3 and 78.3 ± 3.2% increase in the number of apoptotic cells of MCF-7 and HepG2 cells after nanoemulsion treatment. This formula may be considered a new anticancer medication.
Introduction
Among non-communicable illnesses, cancer is a leading cause of death and disability throughout the globe. Millions of people died from cancer every year, and 19.5 million people are diagnosed with cancer in 2022 [Citation1]. Cancer cells are abnormal self-cells that have evaded the body’s regular growth controls [Citation2,Citation3]. In a healthy organism, the generation of new cells is controlled to keep the total amount of a given cell type at a steady state, via a delicate balancing act between cell renewal and cell death. However, tumours and neoplasms form when cells cease responding to normal growth control systems and instead give birth to clones of cells that develop to great size. The abnormalities in cancer cell physiology include; growth signal independence, growth signal insensitivity, programmed cell death resistance, unlimited replicative capacity, persistent angiogenesis, and metastasis [Citation4,Citation5].
The creation of new medications that enhance cancer treatment can take years and is quite expensive. Repurposing existing medications with potential cytotoxic activity is one option to cut down on this time and expense. Drug development is sped up by using the drug repurposing technique, which offers less expensive, more potent, and safe medications with fewer adverse effects. Although these medications were initially indicated for a different condition, they are now frequently used to treat cancer.
Metformin, an oral medication used to regulate blood glucose levels in people with type 2 diabetes, was approved by the US Food and Drug Administration in 1994 [Citation6]. Metformin cellular mechanism is shown to activate AMPK-Thr172 phosphorylation independently of changes in adenine nucleotides. In addition to its approved use as an anti-diabetes medication, metformin has been shown to be effective in the management of polycystic ovary [Citation7] syndrome, hyperinsulinemia [Citation8], obesity and inhibition of endothelial oxidative stress [Citation9]. Metformin is considered one of the drugs that are receiving wide research interest as a promising anticancer agent [Citation10], several studies mentioned that Metformin has been shown to have a favourable effect on markers of tumour proliferation [Citation11]. The incidence of various cancers is higher among patients with T2DM due to insulin resistance, and mitogenic effects caused by hyperglycaemia [Citation12,Citation13]. A retrospective cohort study in type 2 diabetic patients showed that patients on metformin have a lower risk of mortality from cancer than patients who are not taking metformin [Citation14]. Clinical trials were initiated, according to reports, to investigate the potential anticancer effect of metformin against endometrial, prostate, pancreatic, lung, and breast cancer after data from in vitro and preclinical research revealed the anticancer activity of the drug against numerous forms of cancer [Citation15]. Clinical cancer research suggests that metformin may represent an effective and inexpensive means to improve radiotherapy outcomes with an optimal therapeutic ratio [Citation16].
Coconut oil (or coconut butter) is an edible oil derived from the wick, meat, and milk of the coconut palm fruit, Coconut oil contains a variety of saturated fatty acids, which are smaller molecules that makeup fat [Citation17]. A few researchers have examined coconut oil’s potential to fight liver, oral, and lung cancer types [Citation11,Citation18].
Simply described, nanoemulsions are emulsions with a nanometre range size (between tens and hundreds of nanometres) [Citation19]. Nanoparticles have many promising utilities in the drug industry due to their attractive characteristics, including their small sizes, high surface area per unit volume, improved dispersion of active hydrophobic components, enhanced absorption, nanoemulsion preparation, and nanoemulsion stability [Citation20]. Researchers in the field of cancer therapy are interested in nanoemulsions due to their large surface area, superficial charge, increased circulation half-life, and accurate targeting [Citation21]. Nanoemulsions grow swiftly in vascularized tissues due to the fact that they move with cancer cells and may use their diminutive size to overcome obstacles. They may be customized to the issue they are attempting to tackle, use many pharmacological classes, and target certain people. Exciting possibilities exist for nanoemulsions to revolutionize cancer treatment. These novel formulations selectively target cancer cells and overcome the solubility issue.
In this study, we investigated the efficacy of Metformin and coconut oil delivery using nanoemulsion as a nanocarrier to enhance and synergize the anticancer effect of metformin on several cancer cell lines. We looked into the zeta potential, loading and release characteristics, stability, and particle size of the Metformin-coconut oil-formulated optimal nanoemulsion. Additionally, we tested the Metformin-coconut oil nanoemulsion’s anticancer effects on human breast adenocarcinoma (MCF-7), liver carcinoma cells (HePG2), and colon adenocarcinoma (HCT 116).
Materials and methodsMaterials
Metformin (CAD Middle East Pharmaceutical Industries LLC, Riyadh Saudi Arabia), Tween 80 and Span 20 (Merck SA, Darmstadt, Germany), coconut oil (Saudi Green industry, Riyadh, Saudi Arabia). Cell culture materials were purchased from Gibco (Gaithersburg, MD, USA). All other chemicals and solvents used were of analytical grade.
Formulation of nanoemulsion
The nanoemulsion of metformin-coconut oil was formed using coconut oil, water, and surfactant. The mixture of non-ionic surfactant (3.5% Tween 80 and 1.5% Span 20) was added gradually to warm 0.5% of the metformin solution in distilled Milli Q water with a magnetic stirrer to produce a clear solution. A 1.5% of coconut oil was added gradually to aqueous solution of surfactant with continuous stirring at 60 °C. A clear solution was then created by repeatedly heating and cooling the resulting emulsion in an ultrasonicator for 90–120 min at intervals of 25 °C and 70 °C.
Characterization of metformin-loaded coconut oil- nanoemulsion
Measurement of particle size, zeta potential, and polydispersity index (PDI)
The mean droplet size, zeta potential, and PDI of the nanoemulsion were determined by adopting a Malvern particle size analyzer (Zetasizer Nano ZS; Holtsville, NY, USA) kept at 25 ± 1 °C. The nanoemulsion was diluted with distilled (1:200), then transferred to a disposable cuvette for particle size and PDI measurements, and a glass electrode sample holder for zeta potential measurement [Citation22].
Drug content determination
The drug content of metformin-loaded nanoemulsion was evaluated by dissolving 1 ml of formulation in 10 ml methanol. The sample was then centrifuged for 30 min at 5000 rpm. The clear supernatant was then collected and analyzed spectrophotometrically at λmax 228 nm.
In vitro drug release
A Franz diffusion cell with a diffusion area of 0.79 cm2 was employed to conduct in vitro drug release. Briefly, a dialysis membrane (MWCO 12,000 –14,000 Da) was placed between the receptor and donor compartments. 1 ml of either drug-loaded formulation or drug suspension (5 mg/mL) was added to the donor compartment. The receptor compartment was supplemented with 20 ml phosphate buffer saline (PBS; pH 7.4) with 0.5% Tween 80 maintained at 37 ± 1 °C and was stirred at 100 rpm. At scheduled time points, 1 ml samples were collected and replenished with 1 ml of PBS buffer to retain sink conditions. The drug content in each sample was then estimated spectrophotometrically at λmax 228 nm, and the cumulative drug release percentage was calculated.
Stability studies on optimized nanoemulsion
Stability investigations of metformin-loaded nanoemulsion were conducted in accordance with ICH guidelines. Briefly, nanoemulsion formulations were stored at 4 ± 0.5 °C for a period of 3 months. Droplet size, zeta potential, and drug content of different nanoemulsion formulations were estimated and set as parameters for formulation stability.
Cell lines
Human breast adenocarcinoma (MCF-7), liver carcinoma cells (HePG2), and colon adenocarcinoma (HCT 116) cell lines were provided by the American Type Culture Collection (ATCC, Boston, MA, USA). RPMI 1640 medium supplemented with 10% foetal bovine serum and 100 IU/mL PS (penicillin/streptomycin), was used to culture MCF-7, HePG2, and HCT 116 cell lines.
Cell viability assay
Anticancer efficacy of plain metformin, coconut oil nanoemulsion, and metformin-coconut oil activity was assessed using sulforhodamine B (SRB) assay [Citation23]. Briefly, MCF-7, HePG2, and HCT 116 cells, at a density of 1 X 104 cells per well, were plated in 96-well plates for 24 h before being treated with nanoemulsion formulations to adhere to the plate wall. Different concentrations of each formulation (0, 0.01, 0.1, 1, 10, and 100 μg/ml) were applied to each well and the cells were incubated for 72 h at 37 °C. After 72 h, culture media were exchanged with 150 μL of 10% trichloroacetic acid (TCA) for 1 h at 4 °C, followed by 5 times washes with distilled water. The cells were stained with 50 μL SRB solution (0.04% w/v) and incubated for 10 min at 25 °C in a dark area. Afterward, the excess stain was washed away with 1% acetic acid and 100 μL of 10 mM Tris base solution (pH 10.5) was added to each well to dissolve the protein-bound dye. Eventually, a microplate reader (FluoStar Omega, BMG Labtec, Ortenberg, Germany) set at 540 nm was used to estimate the optical density [Citation24].
Cell cycle analysis
MCF-7, HePG2, and HCT 116 cells (1 X 105) were plated in a 6-well plate for 24 to adhere to the plate wall. Cells were treated with either plain metformin, coconut oil nanoemulsion, or metformin-coconut oil nanoemulsion, at their IC50 values, and further incubated for 48 h. Cells were then harvested and fixed in ice-cold 60% ethanol at 40 °C. The cells were then stained with 500 μL of propidium iodide (PI) in RNase staining buffer and incubated for 15 min. Finally, FACS analysis was performed using a Cytek® Northern Lights 2000 spectral flow cytometer (Cytek Biosciences, Bethesda, MD, USA) supplemented with SpectroFloTM Software version 2.2.0.3 [Citation25].
Apoptosis analysis
Annexin V-FITC/propidium iodide staining was conducted to scrutinize the induction of apoptosis in different cell lines upon treatment with metformin [Citation26]. MCF-7, HePG2, and HCT 116 cells (1 X 105) were cultured in a 6-well plate and were incubated for 24 h at 37 °C. Cultured cells were treated with either plain metformin, coconut oil nanoemulsion, or metformin-coconut oil nanoemulsion, at their IC50 values, and further incubated for 48 h. The cells were then trypsinized and washed twice with PBS. Apoptosis assessment was done using Annexin V-FITC/PI apoptosis detection kit (Cell Signalling Technology; Boston, MA, USA), as stated by the manufacturer. In Brief, cell pellets were resuspended in 500 μL of binding buffer and then stained with a staining solution, composed of 5 μL of PI and 5 μL of Annexin V-FITC, with gentle mixing for 15 min at 25 °C in a dark place. Finally, cellular apoptosis/necrosis was evaluated using Cytek® Northern Lights 2000 spectral flow cytometer supplemented with SpectroFloTM Software version 2.2.0.3.
Statistical analysis
All experiments were conducted in triplicate, and the results were reported as mean ± SD. Student’s t-test and one-way analysis of variance (ANOVA) were adopted to evaluate statistical significance using Graph pad prism (version 7). P values < 0.05 were regarded as statistically significant.
Results and Discussion
Characterization of metformin-loaded coconut oil-based nanoemulsion
Particle size, and polydispersity index (PDI) determinations
Coconut oil nanoemulsion and metformin-loaded coconut oil nanoemulsion behaviours can be influenced by a number of physical and chemical factors. Average size is a critical metric. Furthermore, size distribution as manifested by the polydispersity index (PDI) is an important parameter that measures the size distribution’s broadness. Both characteristics can be evaluated using the dynamic light scattering (DLS) technique, which is based on colloidal particle Brownian motion. The formulated nanoemulsions ranged in size from 230.4 ± 16.2 nm for coconut oil nanoemulsion to 310.5 ± 23.1 nm for metformin-loaded coconut oil nanoemulsion (), which was within the nanoparticulate range of 1000 nm, as summarized in . The addition of metformin clearly increased the size of the nanoemulsions. The PDI values of the nanoemulsions ranged from 0.475 to 0.611. A PDI of < 0.8 indicates a nearly monodisperse sample. These PDI values assure that no aggregation/agglomeration of nanoemulsion droplets occurs in both coconut oil nanoemulsion and metformin-loaded coconut oil nanoemulsion.
Table 1. Physicochemical characteristics of nanoemulsion formulations.
Zeta potential of nanoemulsion formulations
Another important parameter that influences formulation stability and/or biological activity is the surface charge, which is expressed primarily as zeta potential. The surface charge of the nanoemulsions was thus determined. The Zeta Potential values for coconut oil plain nanoemulsion and metformin-coconut oil nanoemulsion were −14.3 ± 4.2 and −18.9 ± 3.2 mV, respectively ( and ). These findings demonstrated that the nanoemulsions created in this study were stable under dynamic conditions. The slightly negatively charged coconut oil and surfactants on the particle surfaces at neutral pH may be explained by the relatively negative zeta potential values of different formulations.
Drug content analysis
The amount of drug loaded into a nanoemulsion is an important formulation parameter determining the amount delivered to a target tumour tissue. Metformin-loaded coconut oil nanoemulsion had a drug content of 95.23 ± 0.92%. This result demonstrated that the nanoemulsion had a high drug loading capacity, which is required for nanoemulsion formulation.
In vitro release study
Using a Franz-type diffusion cell apparatus, the in vitro release profile of metformin from formulated nanoemulsion was investigated. As depicted in , by being highly water soluble, pure metformin was released rapidly in the dissolution medium with more than 90% of the drug was released within the first hour. On the other hand, metformin-loaded nanoemulsion sustained drug release for up to 6 h. The percentage drug release from metformin-loaded nanoemulsion was in a range of 87.3 ± 2.3% after 360 min. Formulation characteristics such as droplet size of nanoemulsion, drug-oil and surfactant-oil interactions and drug solubility in oil, were found to have a significant impact on drug release from nanoemulsions ().
Stability studies
Stability tests are essential for characterizing nanoemulsions. The effect of storage conditions on the stability of formulated drug-loaded nanoemulsion was investigated. for stability study, drug-loaded nanoemulsion formulation was stored for 90 days at 4 ± 0.5 °C, then the change in particle size, zeta potential and % drug content were set as parameters to examine formulation stability when compared with fresh (0 day) formulation. The data in represent particle size, zeta potential and % drug content of fresh formulation (Day 0) and stored formulation (Day 90). As shown in , there was no remarkable change in the particle size, zeta potential, or drug content of metformin-loaded nanoemulsion, indicating that the nanoemulsion formulations were stable and remained clear and transparent for three months.
Table 2. Stability profile of formulated nanoemulsion after three months.
In vitro cytotoxicity of metformin-loaded coconut oil nanoemulsion
The sulforhodamine B (SRB) assay is one of the most commonly used colorimetric assays for evaluating cell viability via measuring cellular protein content. Consequently, an SRB assay was adopted to assess the cytotoxic potential of metformin-loaded coconut oil nanoemulsion against MCF-7, HepG2, and HCT 116 cells. As depicted in , metformin remarkably lowered the viability of all tested cell lines in a dose-dependent manner. The IC50 values observed on various cell lines are summarized in . It was evident that metformin has a potent potential activity towards MCF-7 cancer cells, with IC50 19.61 ± 1.3 µg/mL and promising effect on HepG2 and HCT 116 cells with IC50 values of 18.17 ± 1.33 and 6.26 ± 02.1 µg/mL, respectively. Plain coconut oil nanoemulsion exerted a pronounced cytotoxic effect against all the tested cell lines, with a lower cytotoxic effect against MCF7 cells (IC50 44.72 ± 4.3 µg/mL) compared to either HepG2 or HCT116 cancer cells (IC50 values were 24.84 ± 3.9 µg/mL and 35.5 ± 4.2 µg/mL, respectively.
Figure 3. Cell viability of MCF-7 following treatment with various concentrations (0–100 μg/mL) of either metformin, coconut oil nanoemulsion, or metformin-loaded coconut oil nanoemulsion for 72 h. data represent mean ± SD.
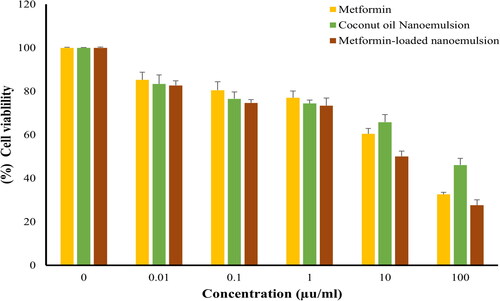
Figure 4. Cell viability of HePG-2 following treatment with various concentrations (0–100 μg/mL) of either metformin, coconut oil nanoemulsion, or metformin-loaded coconut oil nanoemulsion for 72 h. data represent mean ± SD.
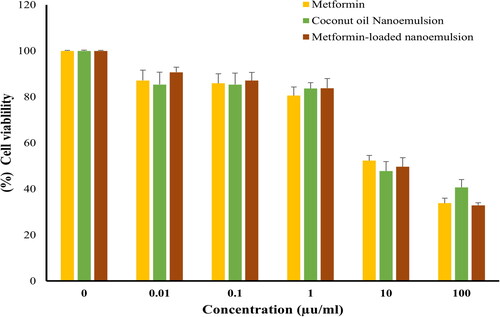
Figure 5. Cell viability of HCT116, following treatment with various concentrations (0–100 μg/mL) of either metformin, coconut oil nanoemulsion, or metformin-loaded coconut oil nanoemulsion for 72 h. data represent mean ± SD.
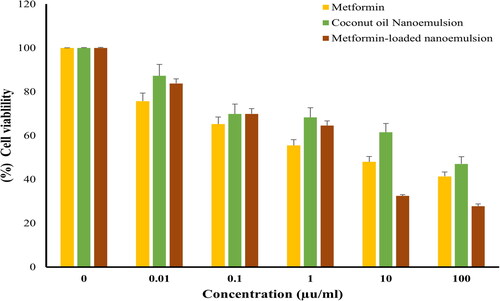
Table 3. The IC50 of different formulations against different human Solid tumour cells.
Cell cycle analysis
Attempting to check the tumour cell cycle phases was used to investigate anticancer effects. As a result, after 48 h of treatment in cancer cells, flow cytometry was used to examine the impact of metformin-loaded nanoemulsion on the distribution of cell cycle phases in MCF-7, HepG2, and HCT 116 cells. The metformin-loaded nanoemulsion formulation significantly arrested the MCF-7 cancer cells in the G1 phase with a ratio of 48.75 ± 2.1%, as well as when the HCT 116 cells treated with the same formulation significantly increased the number arrested in the G1 phase by 55.04 ± 3.2%. Also, with a ratio of 38.3 ± 1.7 and 36.6 ± 1.9 in HepG2 cells were significantly arrested in the G1 and G2 phases, respectively in comparison to the control cell ().
Evaluate cell apoptosis using Annexin V-FITC
Annexin V-FITC/PI staining in MCF-7, HepG2, and HCT 116 cells was used in conjunction with flow cytometry to identify the apoptosis mechanism of the metformin-loaded coconut oil nanoemulsion. Far before taking into account the formulation’s effect on cancer cells, there was a 76.5 ± 2.3 and 78.3 ± 3.2% increase in the number of apoptotic cells MCF-7 and HepG2 cells after metformin-loaded coconut oil nanoemulsion treatment, respectively. The apoptotic effect in colon cancer cells (HCT116) was reduced slightly to 74.46 ± 1.9% after treatment with the combination formula, comparable to the apoptotic percent in other cancer cells ().
Figure 7. Cellular apoptosis of MCF-7, HePG2, and HCT 116 treated with metformin-loaded nanoemulsion for 48h. Dot plots depict apoptotic cell distribution following annexin V-FITC/PI staining. Column charts represent a quantitative evaluation of apoptotic cell percentage post-Metformin-loaded nanoemulsion treatment. Data are presented as the mean ± SD.
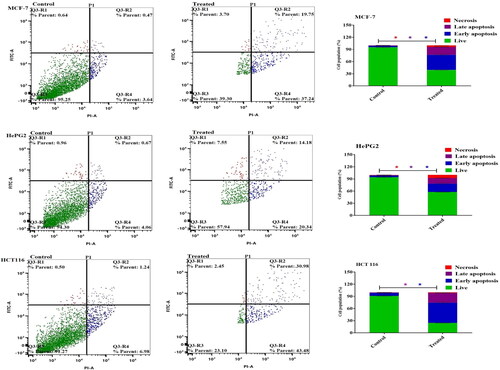
Discussion
The emerging trend in the drug delivery system is primary concerned with the effective delivery of loaded drugs to the target site at adequate concentrations. Nanoemulsions are one of the most promising nano-delivery systems for the targeted delivery of both hydrophilic and lipophilic cytotoxic agents to tumour site [Citation21]. In this study, coconut oil, as an oily phase, was challenged for its efficiency to produce a stable nanoemulsion formulation for the hypoglycaemic agent metformin along with its potential to augment the cytotoxic potential of metformin against various cancer cell lines (MCF-7, HepG2, and HCT-116). Our results revealed the efficacy of coconut oil to produce a physically stable nanoemulsion system with an average size of size 310.5 ± 23.1 nm and a zeta potential of −18.9 ± 3.2 mV. Most importantly, entrapment of metformin within the developed coconut oil-based nanoemulsion systems efficiently potentiate its cytotoxic effect against test cancer cell lines, compared to pure metformin. Collectively, our results underscored the potential use of coconut oil-based nanoemulsion as an adjuvant to chemotherapeutic agents in treating cancer patients.
Anticancer effect is usually mediated by the inhibition of cancer cell proliferation. In this study, we demonstrated that metformin-loaded coconut-based nanoemulsion efficiently suppressed the growth of all test cancer cell lines (MCF-7, HepG2, and HCT-116) in a dose-dependent manner (), with maximum efficacy (>70% growth inhibition) observed at a concentration of 100 μM. This cytotoxic effect of metformin-loaded coconut-based nanoemulsion was comparably higher than those of pure drug, signifying the efficiency of coconut oil in augmenting the cytotoxic potential of free metformin. The IC50 values of metformin against all test cell lines were reduced by up to 50% upon its incorporation into coconut-based nanoemulsion, compared to free metformin. Similar results were reported by Alkhatib et al. [Citation27], who emphasized the potential role of coconut oil in enhancing the cytotoxic potential of the antimetabolite methotrexate against breast cancer cells. The proposed mechanism for the enhanced cytotoxicity of metformin-loaded coconut-based nanoemulsion might be ascribed to the preferential cellar uptake of nanoemulsion formulation with subsequent efficient drug release within tumour cells. Nanoemulsions owing to their particle size in the range of few nanometres have been reported to facilitate the uptake and/or internalization of entrapped drugs within cancer cells, and thereby, boost their anticancer activity [Citation21,Citation28,Citation29].
Cell cycle arrest and induction of apoptosis are two primary causes for the inhibition of cell proliferation [Citation30]. Consequently, to address whether metformin-loaded coconut oil-based nanoemulsion affect cell cycle progression, the distribution of either MCF-7, HepG2, or HCT-116 cancer cells in various cell cycle stages was investigated upon treatment with metformin-loaded coconut oil-based nanoemulsion. Compared with untreated controls, metformin treatment blocked proliferation progression by causing accumulation of G1 phase with a concomitant depletion of S- and G2-phase in all tested cancer cells. The mean percentage of cells in the G1 phase of the cell cycle were 48.75 ± 2.1, 55.04 ± 3.2, and 38.3 ± 1.7% for MCF-7, HepG2, and HCT-116 cells, respectively. These results suggest that, following treatment with metformin-loaded coconut oil-based nanoemulsion, cancer cells were encumbered in their cell cycle progression and were accumulated in the G1 phase. Similar results were reported by Li et al. who demonstrated the efficacy of metformin to induce cell cycle arrest in human osteosarcoma [Citation31].
Apoptosis induction by drugs plays crucial role in the induction of cancer cell death [Citation30]. Accordingly, to assess the apoptotic potential of metformin-loaded coconut oil-based nanoemulsion, cancer cells were labelled with annexin V-FITC and propidium iodide and evaluated using flow cytometry. Annexin V staining effectively distinguish viable cells, apoptotic cells, and necrotic cells. It was obvious that, compared to untreated controls, metformin-loaded coconut oil-based nanoemulsion significantly increased the rate of apoptosis (the sum percentage of early and late apoptotic cells) in MCF-7, HepG2, and HCT-116 cells. The apoptotic rates of metformin-loaded coconut oil-based nanoemulsion were 76.5 ± 2.3%, 78.3 ± 3.2%, and 74.46 ± 1.9% in MCF-7, HepG2, and HCT-116 cells, respectively. These results imply that loading metformin into coconut oil-based nanoemulsion might effectively enhance metformin cytotoxic efficiency not only by increasing cellular uptake of the drug by cancer cells, but also by triggering considerable cell cycle arrest and subsequent production of a robust apoptotic response.
Conclusion
In the current study we challenged the efficacy of coconut oil-based nanoemulsion system to augment the cytotoxic potential of the hypoglycaemic agent, metformin. The loading of metformin into coconut oil as nanoemulsion formulation improved the anticancer properties of metformin and further studied should be performed to introduce this formulation in drug market.
Consent for publication
Not applicable
Authors’ contribution
Hadil Faris designed the experiment, El-Sayed Khafagy and Amr Selim performed methodology, Haifa F. data analysis, Serag Eldin and Mohammad Y. performed anticancer study, Ashwag S., Jawaher Abdullah and Sarah Salem validated the data, Hadil Faris and Fatma Alzahraa supervised the experiment, all authors contributed in the original draft writing, Hadil Faris and Fatma Alzahraa made the final revision.
Acknowledgement
This work was funded by the Deanship of Scientific Research at Princess Nourah bint Abdulrahman University, through the Research Groups Program Grant no (RGP-1443-0049).
Disclosure statement
No potential conflict of interest was reported by the author(s)
Data availability statement
All data generated or analyzed during this study are included in this published article.
Correction Statement
This article has been corrected with minor changes. These changes do not impact the academic content of the article.
Additional information
Funding
References
- Siegel RL, Miller KD, Wagle NS, et al. Cancer statistics, 2023. CA Cancer J Clin. 2023;73(1):17–48. doi: 10.3322/caac.21763.
- Emam SE, Ando H, Lila ASA, et al. Liposome co-incubation with cancer cells secreted exosomes (extracellular vesicles) with different proteins expressions and different uptake pathways. Sci Rep. 2018;8(1):14493. doi: 10.1038/s41598-018-32861-w.
- Chaitanya TS, Patil SN, Ghosh S, et al. Chapter 18 - Cross-presentation-based nanovaccine for cancer immunotherapy. In: rahman M, Beg S, Almalki WH, Alhakamy NA, Choudhry H, editors. Nanotherapeutics in cancer vaccination and challenges. Academic Press, Amsterdam; 2022. p. 349–396.
- Schiliro C, Firestein BL. Mechanisms of metabolic reprogramming in cancer cells supporting enhanced growth and proliferation. Cells. 2021;10(5), 1056) doi: 10.3390/cells10051056.
- Lila AS, Kiwada H, Ishida T. Selective delivery of oxaliplatin to tumor tissue by nanocarrier system enhances overall therapeutic efficacy of the encapsulated oxaliplatin. Biol Pharm Bull. 2014;37(2):206–211. doi: 10.1248/bpb.b13-00540.
- Bailey CJ. Metformin: historical overview. Diabetologia. 2017;60(9):1566–1576. doi: 10.1007/s00125-017-4318-z.
- Mathur R, Alexander CJ, Yano J, et al. Use of metformin in polycystic ovary syndrome. Am J Obstet Gynecol. 2008;199(6):596–609. doi: 10.1016/j.ajog.2008.09.010.
- Mokhtar FA, Selim NM, Elhawary SS, et al. Green biosynthesis of silver nanoparticles using annona glabra and annona squamosa extracts with antimicrobial, anticancer, apoptosis potentials, assisted by in silico modeling, and metabolic profiling. Pharmaceuticals. 2022;15(11), 1354. doi: 10.3390/ph15111354.
- Liu J, Aylor KW, Chai W, et al. Metformin prevents endothelial oxidative stress and microvascular insulin resistance during obesity development in male rats. Am J Physiol Endocrinol Metab. 2022;322(3):E293–E306. doi: 10.1152/ajpendo.00240.2021.
- Abd-Rabou AA, Abdelaziz AM, Shaker OG, et al. Metformin-loaded lecithin nanoparticles induce colorectal cancer cytotoxicity via epigenetic modulation of noncoding RNAs. Mol Biol Rep. 2021;48(10):6805–6820. doi: 10.1007/s11033-021-06680-8.
- Morales DR, Morris AD. Metformin in cancer treatment and prevention. Annu Rev Med. 2015;66:17–29. doi: 10.1146/annurev-med-062613-093128.
- Cheung YM, Hughes M, Harrod J, et al. The effects of diabetes and glycemic control on cancer outcomes in individuals with metastatic breast cancer. J Clin Endocrinol Metab. 2022;107(9):2511–2521. doi: 10.1210/clinem/dgac375.
- Scully T, Ettela A, LeRoith D, et al. Obesity, type 2 diabetes, and cancer risk. Front Oncol. 2020;10:615375. doi: 10.3389/fonc.2020.615375.
- Gandini S, Puntoni M, Heckman-Stoddard BM, et al. Metformin and cancer risk and mortality: a systematic review and meta-analysis taking into account biases and confounders. Cancer Prev Res. 2014;7(9):867–885. doi: 10.1158/1940-6207.CAPR-13-0424.
- Chae YK, Arya A, Malecek MK, et al. Repurposing metformin for cancer treatment: current clinical studies. Oncotarget. 2016;7(26):40767–40780. doi: 10.18632/oncotarget.8194.
- Rao M, Gao C, Guo M, et al. Effects of metformin treatment on radiotherapy efficacy in patients with cancer and diabetes: a systematic review and meta-analysis. Cancer Manag Res. 2018;10:4881–4890. doi: 10.2147/CMAR.S174535.
- Marina AM, Che Man YB, Amin I. Virgin coconut oil: emerging functional food oil. Trends Food Sci Technol. 2009;20(10):481–487. doi: 10.1016/j.tifs.2009.06.003.
- Verma P, Naik S, Nanda P, et al. In vitro anticancer activity of virgin coconut oil and its fractions in liver and oral cancer cells. Anticancer Agents Med Chem. 2019;19(18):2223–2230. doi: 10.2174/1871520619666191021160752.
- Sonneville-Aubrun O, Simonnet JT, L'Alloret F. Nanoemulsions: a new vehicle for skincare products. Adv Colloid Interface Sci. 2004;108-109:145–149. doi: 10.1016/j.cis.2003.10.026.
- Rai VK, Mishra N, Yadav KS, et al. Nanoemulsion as pharmaceutical carrier for dermal and transdermal drug delivery: formulation development, stability issues, basic considerations and applications. J Control Release. 2018;270:203–225. doi: 10.1016/j.jconrel.2017.11.049.
- Sánchez-López E, Guerra M, Dias-Ferreira J, et al. Current applications of nanoemulsions in cancer therapeutics. Nanomaterials. 2019;9(6):821. doi: 10.3390/nano9060821.
- Abdallah MH, Abu Lila AS, Unissa R, et al. Preparation, characterization and evaluation of anti-inflammatory and anti-nociceptive effects of brucine-loaded nanoemulgel. Colloids Surf B Biointerfaces. 2021;205:111868. doi: 10.1016/j.colsurfb.2021.111868.
- Vichai V, Kirtikara K. Sulforhodamine B colorimetric assay for cytotoxicity screening. Nat Protoc. 2006;1(3):1112–1116. doi: 10.1038/nprot.2006.179.
- Houghton P, Fang R, Techatanawat I, et al. The sulphorhodamine (SRB) assay and other approaches to testing plant extracts and derived compounds for activities related to reputed anticancer activity. Methods. 2007;42(4):377–387. doi: 10.1016/j.ymeth.2007.01.003.
- Nunez R. DNA measurement and cell cycle analysis by flow cytometry. Curr Issues Mol Biol. 2001;3:67–70.
- Moin A, Wani SUD, Osmani RA, et al. Formulation, characterization, and cellular toxicity assessment of tamoxifen-loaded silk fibroin nanoparticles in breast cancer. Drug Deliv. 2021;28(1):1626–1636. doi: 10.1080/10717544.2021.1958106.
- Alkhatib MH, Alyamani SA, Abdu F. Incorporation of methotrexate into coconut oil nanoemulsion potentiates its antiproliferation activity and attenuates its oxidative stress. Drug Deliv. 2020;27(1):422–430. doi: 10.1080/10717544.2020.1736209.
- Choudhury H, Pandey M, Gorain B, et al. Chapter 9 - Nanoemulsions as effective carriers for the treatment of lung cancer. In: Kesharwani P, editor. Nanotechnology-based targeted drug delivery systems for lung. Cancer: Academic Press; 2019. p. 217–247.
- Cheng Z, Li M, Dey R, et al. Nanomaterials for cancer therapy: current progress and perspectives. J Hematol Oncol. 2021;14(1):85. doi: 10.1186/s13045-021-01096-0.
- Al Saqr A, Khafagy ES, Aldawsari MF, et al. Screening of apoptosis pathway-mediated anti-proliferative activity of the phytochemical compound furanodienone against human non-small lung cancer A-549 cells. Life. 2022;12(1), 114. doi: 10.3390/life12010114.
- Li B, Zhou P, Xu K, et al. Metformin induces cell cycle arrest, apoptosis and autophagy through ROS/JNK signaling pathway in human osteosarcoma. Int J Biol Sci. 2020;16(1):74–84. doi: 10.7150/ijbs.33787.