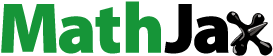
Abstract
The present study describes a method for the preparation of green titanium dioxide (TiO2) nanoparticles from the peel of Solanum tuberosum, commonly known as potato, and the potato peel being a kitchen waste. The green synthesized TiO2 (G- TiO2) nanoparticles were characterized using UV-visible spectroscopy, dynamic light scattering, scanning electron microscopy, TEM, XRD, and FTIR spectroscopy. The photocatalytic activity of the G- TiO2 nanoparticles was also shown using the dye bromophenol blue. To explore the biocompatibility of the G- TiO2, the cell viability in normal as well as cancer cells was assessed. Further, the in vivo toxicity of the G- TiO2 nanoparticles was assessed using zebrafish embryos. The novelty of the present invention is to utilize kitchen waste for a useful purpose for the synthesis of titanium dioxide nanoparticles which is known to have UV light scavenging properties. Moreover, the potato peel is a natural antioxidant and possesses a skin-lightening effect. A combination of the potato peel extract and titanium dioxide prepared using the extract will have a combinatorial effect for protecting UV light exposure to the skin and lightening the skin colour.
Introduction
Nanoscience and nanotechnology have been recently used in different biomedical fields among which metal as well as metal oxide nanoparticles have gained particular attention [Citation1]. Photocatalysis mediated by engineered nanoparticles has been researched profoundly for their potential applications in cosmetics, paints, remediation of wastewater, and many more [Citation2–4]. Titanium dioxide (TiO2) is a metal oxide semiconductor nanoparticle exhibiting a band gap similar to a semiconductor and has been used profoundly as a component of sunscreens, paints, and cosmetics for their capacity to scavenge the harmful ultraviolet (UV) light, thereby offering protection to the skin [Citation4]. TiO2 nanoparticles are also added to other nanostructures to make nanocomposites that exhibit superior activities, such as water splitting [Citation5] or photocatalysis [Citation6]. The drawback of chemically synthesized TiO2 is that the harmful chemicals used for its synthesis may cause damage to the skin. To overcome these limitations, many green synthesis methods are utilized for the TiO2 nanoparticle synthesis [Citation7].
Several green synthesis methods have been exploited for the TiO2 nanoparticle synthesis. Cicer arietinum L. extract [Citation8], Aloe Vera leaf extract [Citation9], Annona squamosa fruit peel extract (an agricultural waste) [Citation10], aqueous extract of Acanthophyllum laxiusculum [Citation11], and Psidium guajava extract [Citation12], were used by different researchers to synthesize TiO2 NPs and they exhibited different properties, such photocatalytic or antimicrobial. In another study, the Jatropha curcas L aqueous extract was used to synthesize TiO2 NPs with an average size between 25–100 nm [Citation13]. Hydrothermal synthesis of TiO2 NPs using extract of Piper betel leaf, a novel biogenic source was executed as capping and reducing agent . The average size of the synthesized TiO2 NPs was about 8–75 nm [Citation14]. Sundrarajan et al. (2017) used the leaf extract of M. citrifolia to synthesize TiO2 NPs via the hydrothermal method and the synthesized nanoparticles showed antimicrobial activity [Citation15]. Solanum trilobatum extract medicated TiO2 NP synthesis was carried out which inhibited the growth of Hyalomma anatolicum, Pediculus humanus capitis, and Anopheles subpictus [Citation16]. Sankar et al. green synthesized TiO2 NPs by using Azadirachta indica aqueous leaf extract with dependence on pH and temperature that yielded a mean particle size of 124 nm [Citation17]. Maiden attempt was made to synthesize TiO2 NPs using Catharanthus roseus aqueous extract by Velayutham et al. with a particle size of 25–110 nm. These TiO2 NPs showed activity against Bovicola ovis and Hippobosca maculata [Citation18]. Using Cinnamomum Tamala leaves extract, TiO2 NPs were synthesized, because this extract acted as the reducing agent [Citation19]. Orange peel extract mediated synthesis of TiO2 NPs was done by researchers, where the citric acid present in its peel acted as a reducing as well as a capping agent [Citation20]. In another study, Chatterjee et al. used Vigna radiata extract to synthesize TiO2 NPs that inhibited the growth of both Gram-positive and Gram-negative bacteria [Citation21].
Potato (Solanum tuberosum) peel is a kitchen waste and can be useful for the sustainable synthesis of TiO2 nanoparticles. Moreover, Potato peel is a natural antioxidant [Citation22] and is known to possess de-tanning activity, i.e., it can reduce the pigmentation of dark skin caused due to exposure to the sun. So far, many techniques have been developed for the synthesis of green TiO2 nanoparticles, but the synthesis using potato peel extract has not been done. Thus, a combination of a nanoparticle with UV light-protecting activity as well as skin-lightening properties will be ideal to be used in cosmetics. In order to provide a superior sunscreen property along with skin-lightening action, there is a need in the art to develop a technique for the synthesis of green TiO2 nanoparticles, using potato peel extract.
In this study, we have synthesized TiO2 nanoparticles using potato peel extract and characterized the nanoparticles using different photophysical tools, like scanning electron microscopy, FTIR spectroscopy, UV visible spectrophotometry, dynamic light scattering for hydrodynamic diameter measurement and zeta potential for its stability in the aqueous environment. We have done the phytochemical analysis of the potato peel extract also. To assess the biocompatibility of the synthesized nanoparticles in vitro and in vivo, we have tested the cytotoxicity using cell lines as well as zebrafish embryos, respectively.
Materials and methods
Materials
Titanium tetraisopropoxide, acetic acid, isopropanol, bromophenol blue, DMEM, Antibiotics, and XTT assay kit were purchased from HiMedia Ltd. Foetal bovine serum was procured from Gibco Ltd. Potatoes were procured locally. L132 and A549 cells were purchased from NCCS, Pune. We have purchased the zebrafish embryos from the local supplier whose details is given below: Name: Srider; Address: No:2/23 C, East Mada street, Manimangalam, Chennai-601301, Tamilnadu, India.
Potato (Solanum tuberosum) peel collection, extraction, and phytochemical analysis
Peel of potato (Solanum tuberosum) was obtained by freshly peeling the potatoes and dried at room temperature (25 °C) for 1 day. The dried peel was ground in a grinder, and processed immediately for the extract preparation. 20 g of the grinded powder was added to 100 ml of distilled water followed by heating at 60 °C for 2 h to procure the potato peel extract. Filtration of the extract was done using a muslin cloth followed by Whatman filter paper (Cat # 1440-090) filtration of pore size 8 μm. The extract was collected and stored at 4 °C in the refrigerator for further analysis. Various biochemical tests were performed for the analysis of the phytochemicals present in the potato (Solanum tuberosum) peel extract. The methods used were according to Garg et al. [Citation23–26]. Briefly, the presence of carbohydrates was determined by Fehling’s test, Benedict’s test, and Barford’s test. Proteins and amino acids were tested by Ninhydrin test and Biuret test. Mayer’s and Hager’s test was employed to detect the presence of alkaloids. Saponins were detected using the Foam test and flavonoids were detected by Ferric chloride test, alkaline reagent test, lead acetate solution test, and sodium hydroxide test. Glycosides and tannins were detected using Keller Kiliani and Gelatine tests, respectively.
Preparation of chemically and green synthesized titanium oxide nanoparticles
The extract obtained in the previous step was used for the sol-gel synthesis of TiO2 nanoparticles. Briefly, 5.2 ml of isopropanol was mixed with 2.5 ml of acetic acid in a 100 ml clean and dry beaker and stirred for 15 min using a magnetic stirrer. The beaker was covered with aluminium foil to avoid evaporation. Further, 2.5 ml of titanium tetraisopropoxide was added dropwise to this solution with continuous stirring for a further 15 min. For the gel formation, 1.75 ml of potato peel extract obtained in the previous step was added dropwise and stirred continuously for the preparation of green TiO2 nanoparticles. For chemically synthesized TiO2 nanoparticles, 1.75 ml of double distilled water was added in this step. After 20 min of stirring a gel was formed which was decanted into a tube and added with 5 ml of distilled water for washing and removing excess solvent. Then, the tube was centrifuged at 8000 rpm for 5 min, and the supernatant was discarded and the step was repeated twice. The pellet that remained after centrifugation was taken in a porcelain crucible and dried at 80 °C for 1 h. After complete drying, the remaining residue was ground to a fine powder using a mortar pestle and calcinated at 300 °C. This fine powder obtained was as synthesized chemically synthesized TiO2 nanoparticles (TiO2) and green TiO2 nanoparticles (G-TiO2).
Characterization of nanoparticles
Various photophysical tools were used for the characterization of the as- synthesized TiO2 and G- TiO2 nanoparticles including UV-visible spectrophotometry (Shimadzu UV 1800) for the measurement of characteristic absorption spectrum, dynamic light scattering (Malvern zeta sizer) for the hydrodynamic diameter measurement, and surface charge, Fourier transformed infra-red (FTIR) spectroscopy (Bruker alpha) for the measurement of characteristic bonds present, and Scanning electron microscopy (SEM) (FEI Quanta FEG 200) for surface morphology visualization, and size of the synthesized green titanium dioxide nanoparticles [Citation1,Citation3,Citation27]. TEM analysis was done using (Talos F200S G2 TEM) at 80 Hz to find the size of the nanoparticles [Citation28] and XRD (Bruker Kappa APEXII) was executed to confirm the synthesis of the crystalline (rutile or anatase) TiO2 nanoparticles, setting the 2θ values from 20 to 80 degrees [Citation1]. For the analysis of the absorption spectrum using UV visible spectrometer, hydrodynamic diameter, and zeta potential measurement using dynamic light scattering, the samples were prepared in the same manner; briefly, 0.001 g of the ynthesized respective titanium dioxide nanoparticles were dissolved in 25 ml of distilled water taken in a glass beaker and sonicated for 15 min in a bath sonicator. This solution was used for the measurement of absorption using a Shimadzu (UV1800) UV-visible spectrophotometer; and hydrodynamic diameter and surface charge using a Malvern zeta sizer. For FTIR measurement, standard KBr pellet were prepared using dry powder of the respective titanium dioxide nanoparticles and the potato peel extract which was dried at 40 °C, and powdered and the transmittance were measured using Bruker alpha FTIR in the range from 500 to 4000 cm−1. The samples were prepared for the SEM analysis by coating a thin layer of synthesized respective titanium dioxide nanoparticles over a clean aluminium foil and vacuum dried, which was further scanned for SEM as well as EDX analysis. For the sample preparation of TEM, the powdered samples were coated on a copper grid and processed further for analysis. Powdered sample were used for XRD analysis.
Photocatalytic activity of the green titanium dioxide nanoparticles
The photocatalytic application of TiO2 and G- TiO2 nanoparticles was done using the dye bromophenol blue (BB). 1 mg of bromophenol blue was dissolved in 50 ml of distilled water. It is then divided into five parts equally, and to four parts (5 ml each) 0.02 gm of synthesized nanoparticles was added and in the fifth part (5 ml) of the solution nothing was added. The tubes were exposed to sunlight for 10, 20, 30, and 40 min respectively for the first four tubes and the tubes with G-TiO2 nanoparticles and bromophenol blue solution were centrifuged at 12000 rpm for 10 min, immediately after sunlight exposure to remove the nanoparticles, and the supernatant was collected. Now, the absorbance spectra of the supernatant in the respective tubes and the tube with bromophenol blue solution alone were measured and the absorbance at the absorption maxima of bromophenol blue (590 nm) was used to calculate the percentage of photocatalytic activity using the formula:
Where,
OD1-absorbance value of only BB;
OD2-absorbance value of BB + G-TiO2.
The decrease in absorbance at 590 nm was plotted for the samples exposed to sunlight at different time points.
Cell viability assay using XTT reagent
To ensure the biocompatibility of TiO2 and G- TiO2 nanoparticles, an XTT assay was performed as done earlier [Citation27]. L132 cells and A549 cells were cultured in DMEM containing 10% FBS and 1% antibiotics and incubated at 37 °C in a 5% CO2 with humidified atmosphere. The assessment of cell viability was done using XTT assay using the protocol supplied by the manufacturer (Hi-Media). Briefly, both L132 cells and A549 cells were seeded at a low density (10 4 cells in each well) of a 96- well plate, respectively, and incubated for 24h to adhere at the bottom of the plate. After 24 h, treatment was done at different concentrations (25 μg/ml, 50 μg/ml, 100 μg/ml, 150 μg/ml, and 200 μg/ml) of TiO2 and G-TiO2 nanoparticles, respectively. Post 24 h of treatment, under sterile conditions, the XTT dye was added to each well and allowed to further incubate for 4 h at 37 °C in a CO2 incubator. The cells which were metabolically active changed the colour of the solution to orange and their O.D. at 450 nm was measured in a Robonik ELISA plate reader. The percentage of cell viability was calculated using the following formula
Where, ODsample- the absorbance at 450 nm of the TiO2 treated cells;
ODcontrol- the absorbance at 450 nm of the untreated cells.
In vivo biocompatibility using zebrafish embryos
The biocompatibility of both TiO2 and G- TiO2 nanoparticles was tested using zebrafish embryos. The embryos were obtained and tested for cumulative hatchability and microscopic observations as mentioned earlier [Citation1,Citation3,Citation29]. After isolating healthy embryos in 6 well plates, they were treated with different doses of TiO2 and G-TiO2 and observed under a light microscope for any developmental abnormality for a time period. The cumulative hatchability was scored for the embryos and the experiment was repeated three times.
Results and Discussion
Phytochemical analysis of potato (Solanum tuberosum) peel aqueous extract
The phytochemical analysis was done for the aqueous extract of potato (Solanum tuberosum) peel for carbohydrates, alkaloids, proteins and amino acids, saponins, flavonoids, glycosides, and tannins. Figure S1 shows the colour developed after performing the different biochemical tests, namely, Fehling’s test, Benedict’s test, and Barford’s test, for the presence of carbohydrates, Ninhydrin test, for the presence of proteins and amino acids, Mayer’s test, and Hager’s test for the presence of alkaloids and Tannin’s test for the presence of tannins. Table S1 shows the different phytochemicals present in the extract.
The phytochemical analysis showed that the aqueous extract of potato (Solanum tuberosum) peels contained carbohydrate, proteins and amino acids, alkaloids and tannins at high concentrations. These compositions may contribute towards the skin pigment-lightening effect.
Absorption spectrum of chemical and green synthesized titanium dioxide nanoparticles
The absorption spectrum of TiO2 and G-TiO2 nanoparticles is shown in , respectively. The absorption maximum was obtained at 261 nm for chemically synthesized TiO2 nanoparticles. For G-TiO2 nanoparticles, the absorption maxima were at 288 nm, with a wide absorption range in the UV region of the spectrum (250 nm–400nm). The absorption peak at 288 nm and a broad absorption in the UV range of G-TiO2 nanoparticles indicated that the synthesized nanoparticles are efficient scavengers of UV light and they can be effectively used as sunscreens or for blocking UV light when added to any composition. The band gap of the synthesized nanoparticles was calculated using Tauc’s plot. The direct band gap for TiO2 and G-TiO2 nanoparticles was found to be 3.09 and 2.82 eV, respectively. This clearly indicated the semiconducting nature of both TiO2 and G-TiO2 nanoparticles.
Figure 1. The absorption spectrum of the (a) chemically synthesized TiO2 nanoparticles and (b) G-TiO2 nanoparticles. The FTIR spectrum of the (c) chemically synthesized TiO2 nanoparticles and (d) G-TiO2 nanoparticles (e) potato peel extract. The zeta potential of the (f) chemically synthesized TiO2 nanoparticles and (g) G-TiO2 nanoparticles. The XRD spectra of (h) chemically synthesized TiO2 nanoparticles and (i) G-TiO2 nanoparticles. The hydrodynamic diameter measured using dynamic light scattering of the (j) chemically synthesized TiO2 nanoparticles and (k) G-TiO2 nanoparticles.
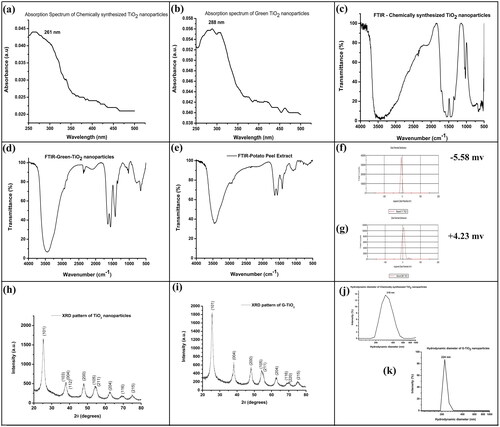
FTIR analysis of chemical and green synthesized titanium dioxide nanoparticles
The characteristic bonds present in the sample were analyzed using FTIR spectroscopy. The FTIR spectra for the TiO2, G-TiO2 nanoparticles, and potato peel extract are shown in , respectively. The FTIR spectra of TiO2 nanoparticles () showed the peaks at 3444 cm−1 representing the O-H stretching, and the 1024 cm− 1 band represented the characteristic peak of OH out-of-plane vibration [Citation30]. The peaks at 523 cm−1 and 662 cm−1 represented the vibration of the Ti- O- O bond [Citation31]. 1449 cm−1 and 1560 cm−1 peaks represented bending vibration of the functional groups CH, CH2, and CH3 [Citation32]. The FTIR spectra of G-TiO2 nanoparticles showed typical characteristic bonds of CC at 1652 cm−1 (asymmetric carboxylic vibrations) () which was visible at 1560 cm−1 for only potato peel extract FTIR spectra () [Citation33]. The peak at 1419 cm−1 for symmetric carboxylic vibrations was visible for G-TiO2 nanoparticles () which was visible at 1417 cm−1 for the only potato peel extract FTIR spectra () [Citation33]. These peak shifts indicated the incorporation of the peel extract in the nanoparticles. The O
H stretching is represented at 3440 cm−1, and the peak below 1000 cm−1 represents the presence of Ti-O-Ti for G-TiO2 nanoparticles (). In the FTIR spectrum of the potato peel extract, there was the presence of a few peaks in common with the G-TiO2 nanoparticles which indicated that the G-TiO2 nanoparticles were coated with the extract obtained from the potato peel. In , a strong peak at 3458 cm−1 showed the stretching of O-H and the C-O stretching was indicated by the presence of 1077 cm−1 peak. This ensures that the titanium dioxide nanoparticles were synthesized successfully using aqueous extract of potato (Solanum tuberosum) peel.
Zeta potential analysis
The stability of a nanoparticle in an aqueous environment is measured using the surface charge of the nanoparticle represented as zeta potential. Chemically synthesized TiO2 nanoparticles had a zeta potential of −5.58 mV whereas the zeta potential of the G-TiO2 nanoparticles was found to be +4.23 mV, as shown in , respectively. Previous reports suggested that the pH can control the size, shape, and zeta potential of TiO2 nanoparticles. As the pH increases the zeta potential becomes negative [Citation34]. The surface charge showed that both the nanoparticles were moderately stable in the aqueous environment.
XRD analysis
To confirm the crystalline structure of the synthesized nanoparticles, XRD analysis was done. The XRD spectrum of TiO2 and G-TiO2 nanoparticles are shown in , respectively. The peaks present in correspond to the hkl peaks of 101, 103, 004, 112, 200, 105, 211, 204, 116, and 215 that relate to a combination of rutile and anatase structure. The hkl peaks for G-TiO2 nanoparticles are shown in indicating the presence of 101, 004, 200, 105, 211, 204, 116, 220, and 215. All the peaks obtained for both TiO2 and G-TiO2 are in good agreement with the standard JCPDS spectrum (JCPDS 89-4921) described by previous researchers [Citation35,Citation36]. The obtained XRD pattern of these nanoparticles belongs to the anatase phase, which has a tetragonal crystal system [Citation37].
The hydrodynamic diameter of titanium dioxide nanoparticles synthesized by chemical and green route
Dynamic light scattering is differently named as quasi-elastic light scattering or Photon correlation spectroscopy. It is used to measure the scattered light of a sample in very short periods of time and correlates the data. When the light passes through the sample, the particles are illuminated and the intensity of the scattered light fluctuation rate is dependent on the size of the particle as smaller particles move more rapidly. By analyzing these intensity fluctuations, the Strokes-Einstein relationship is used to calculate the Brownian motion velocity and particle size. The hydrodynamic diameter of the chemically synthesized and green synthesized titanium dioxide nanoparticles is found to be 319 nm and 224 nm as shown in , respectively. This indicated that the titanium dioxide nanoparticles were synthesized in the nano dimension.
Surface morphology (SEM), composition and TEM image of the synthesized TiO2 nanoparticles
The scanning electron microscopic image shows the surface morphology of the synthesized nanoparticles. EDX analysis gives the composition of the nanoparticle synthesized. From it is seen that the particle size of the as- synthesized nanoparticles is in the range of 27-45 nm with spherical morphology for chemically synthesized TiO2 nanoparticles. The morphology of G-TiO2 nanoparticles was also spherical with particle size ranging from 34-53 nm (). The TEM image shows the size of the nanoparticles with further accuracy. The size of TiO2 nanoparticles was around 5 nm as visible from , whereas the size of G-TiO2 nanoparticles was nearly 27 nm (. The size also corroborates that our nanoparticles synthesized by using the aqueous extract of potato (Solanum tuberosum) peel have a very small size below 100 nm and is expected to exhibit good photocatalytic activity. The EDX analysis also showed that the composition contains Titatnium (Ti) and oxygen (O) which is obvious from the respective peaks and percentage table depicted in for chemically synthesized TiO2 nanoparticles and for G-TiO2 nanoparticles, respectively. The surface scanned for EDX analysis is shown in and , respectively for chemically synthesized TiO2 nanoparticles and G-TiO2 nanoparticles. Thus, the synthesis was very successful. In a previous study, it was found that TiO2 nanoparticles were synthesized with a particle size of as low as 12 nm and having a tetragonal crystal structure. The particles also exhibited excellent photocatalytic activity [Citation38].
The photocatalytic activity of G-TiO2 nanoparticles
The photocatalytic activity of green synthesized titanium dioxide was exhibited by reducing bromophenol blue in its colour which was documented by a reduction in absorption at 590 nm. shows that bromophenol blue, which has its λmax at 590 nm, has reduced its absorbance after the addition of the G-TiO2 titanium dioxide nanoparticles, in a time-dependent manner. As the time of exposure to the sunlight increased the dye reduction also increased. After 20 min of exposure, the reduction of dye reached a saturation which indicated that 20 min exposure to sunlight was sufficient to reduce the dye significantly. The percentage of dye reduction after the addition of G-TiO2 nanoparticles and 40 min of sunlight exposure was 98%. This showed that the nanoparticles had high photocatalytic activity which was required for UV light scavenging activity. There have been several reports mentioning the exhilarating photocatalytic properties of TiO2 nanoparticles which have been applied for remediation of wastewater [Citation38–40].
Figure 3. Photocatalytic reduction of the dye bromophenol blue using the synthesized TiO2 nanoparticles using the aqueous extract of Solanum tuberosum (G-TiO2). (a)- the absorption spectrum of only bromophenol blue and the absorption spectrum of bromophenol blue treated with synthesized green titanium dioxide nanoparticles and exposed to sunlight for different times (10, 20, 30, and 40 min). The reduction in absorbance at the absorption maxima of bromophenol blue (590 nm) is shown in the inset. The image of the tubes containing bromophenol blue and G-TiO2 nanoparticles (b) before exposure to sunlight and (c) after exposure to sunlight at different time intervals.
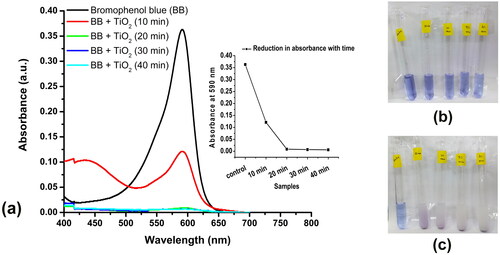
In vitro toxicity analysis of synthesized chemical and green TiO2 nanoparticles
The biocompatibility of the green synthesized titanium dioxide nanoparticles was necessary for its application in cosmetics. Two different cell lines were used for this study, a normal cell line, L132 cells, and a cancer cell line, A549 cells. The results of the XTT assay showed the percent cell viability after treatment with different doses (25 μg/ml, 50 μg/ml, 100 μg/ml, 150 μg/ml, and 200 μg/ml) of the synthesized G-TiO2 nanoparticles as documented in . There was no significant toxicity observed with respect to control in both cell types after exposure to a range of doses of the synthesized G-TiO2 nanoparticles. NR8383 cells were exposed to TiO2 NP samples in vitro and their viability was assessed using WST-1 and LDH assays in a previous study. After 24 h of exposure to TiO2 NP, the viability of NR8383 cells did not statistically decrease. The WST-1 test showed that the metabolic activity of cells exposed to TiO2 at 100 g/mL and 200 mg/mL was not statistically different from that of cells not exposed to the nanoparticles [Citation41]. The results of this study corroborate the findings of our present study.
Figure 4. The biocompatibility of the G- TiO2 nanoparticles was monitored using XTT assay in normal cell lines (L132) and cancer cell lines (A549). The synthesized G-TiO2 nanoparticles were treated at different concentrations in these cells and the cell viability was assessed using XTT assay 24 h after exposure. The percentage of cell viability for (a)L132 cells and (b) A549 cells after exposure to different concentrations of G-TiO2 nanoparticles.
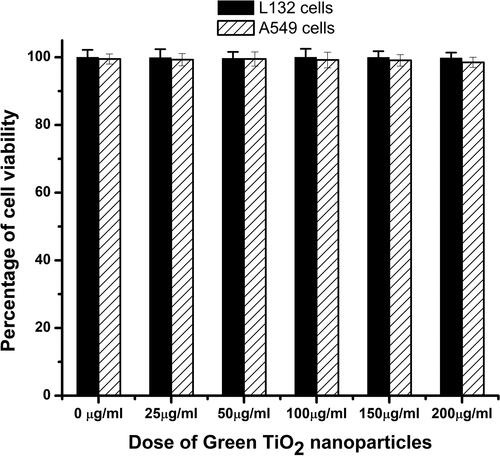
In vivo toxicity analysis of synthesized chemical and green TiO2 nanoparticles
It is known that the TiO2 nanoparticles are mostly used for photocatalysis in cosmetics, paints, and also in wastewater remediation. The fate of these nanoparticles in water bodies needs to be explored because these nanoparticles from most of the sources after use get released into the water bodies. In aquatic environments, whether the synthesized TiO2 nanoparticles were causing any developmental abnormalities, or delayed hatching in zebrafish embryos, it is important to see the effect on these embryos. We have sorted out healthy embryos of nearly 10 hpf, treated them with chemically synthesized TiO2 nanoparticles and G-TiO2 nanoparticles at a sublethal dose (50 μg/ml), and observed their development under a microscope (). The cumulative hatchability was calculated by the number of embryos hatched at a particular time hours post fertilization (hpf) divided by the total number of embryos taken initially for the study. shows the cumulative hatchability of the zebrafish embryos in control and after treatment with 50 μg/ml of chemically synthesized TiO2 nanoparticles and G-TiO2 nanoparticles. No morphological abnormality was observed in either of the treatments, but the chemically synthesized TiO2 nanoparticles showed delayed hatching of the embryos. It has been shown earlier that TiO2 nanoparticles synthesized using Sesbania grandiflora elicited toxicity in zebrafish embryos [Citation42]. In our study we could see that the green synthesized TiO2 did not show any abnormality up to a dose of 50 μg/ml.
Conclusion
In this study, we have attempted to synthesize TiO2 nanoparticles using a kitchen waste, potato peel for its application in sunscreens. The mechanism of synthesis is sol- gel method which is a very well-known process. The process involves hydrolysis, condensation, sol formation, ageing, and calcination. The calcination temperature decides the crystal structure that is formed. In this case, the crystals were predominantly anatase in structure for both TiO2 and G-TiO2 nanoparticles as evidenced by the XRD analysis. It is known that TiO2 is a known photocatalyst that can scavenge harmful UV light and on the other hand, potato peel has a skin- lightening effect. Combined together, this green synthesized nanoparticle can exert its effect on cosmetics. The study results have shown that compared to the chemically synthesized TiO2 nanoparticles, the green synthesized nanoparticles exerted no toxicity on cell lines, both normal cell lines and cancer cell lines as well as zebrafish embryos. These nanoparticles have also exhibited excellent photocatalytic activity as visible from the bromophenol dye reduction. The synthesized nanoparticles have shown excellent ultra-small size in the range of 27-30 nm, were biocompatible, and exhibited excellent photocatalytic activity. It is a dual way to contribute towards sustainability-firstly kitchen waste is utilized and reduces solid waste; secondly, green synthesis reduces the use of harmful chemicals, which remediates liquid waste and decreases pollution. In conclusion, we can state that the synthesized TiO2 nanoparticles using potato peel extract have excellent photocatalytic activity along with high biocompatibility. This can be used in the future for cosmetic use at limited dosages.
Ethical approval Consent to participate
Yes
Authors’ contribution
Conceptualization: K.G.
Experimentation: K.G., D.B., A.K.P., and A.G. Article search, Writing first draft: K.G. Finalizing the manuscript: K.G., D. B., A.K.P., and A.G.
Supplemental Material
Download MS Word (1.6 MB)Acknowledgment
The authors are grateful to Chettinad Academy of Research and Education for providing funding (Grant Number-Ref No. 004/Regr./AR-Research/2022-06). B.D. acknowledge Chettinad Academy of Research and Education for providing the PhD fellowship.
Disclosure statement
No potential conflict of interest was reported by the author(s). All authors certify that they have no affiliations with or involvement in any organization or entity with any financial interest or non-financial interest in the subject matter or materials discussed in this manuscript.
Data availability statement
The data that support the findings of this study are available from the corresponding author, [K.G.], upon reasonable request.
Additional information
Funding
References
- Girigoswami K, Viswanathan M, Murugesan R, et al. Studies on polymer-coated zinc oxide nanoparticles: UV-blocking efficacy and in vivo toxicity. Mater Sci Eng C Mater Biol Appl. 2015;56:501–510. doi: 10.1016/j.msec.2015.07.017.
- Kumar, Y.; Sudhaik, A.; Sharma, K.; Raizada, P.; Khan, A.A.P.; Nguyen, V.-H.; Ahamad, T.; Singh, P.; Asiri, A.M. Construction of magnetically separable novel arrow down dual S-scheme ZnIn2S4/BiOCl/FeVO4 heterojunction for improved photocatalytic activity. J Photochem Photobiol A. 2023; 435: 114326. doi: 10.1016/j.jphotochem.2022.114326.
- Keerthana V, Girigoswami A, Jothika S, et al. Synthesis, characterization and applications of GO–TiO2 nanocomposites in textile dye remediation. Iran J Sci Technol Trans Sci. 2022;46(4):1149–1161. doi: 10.1007/s40995-022-01337-y.
- Jacobs JF, Van de Poel I, Osseweijer P. Sunscreens with titanium dioxide (TiO2) nano-particles: a societal experiment. Nanoethics. 2010;4(2):103–113. doi: 10.1007/s11569-010-0090-y.
- Le AQH, Nguyen NNT, Tran HD, et al. A TiO2@ MWCNTs nanocomposite photoanode for solar-driven water splitting. Beilstein J Nanotechnol. 2022;13:1520–1530. doi: 10.3762/bjnano.13.125.
- Rajaraman T, Gandhi VG, Nguyen V-H, et al. Aluminium foil-assisted NaBH4 reduced TiO2 with surface defects for photocatalytic degradation of toxic fuchsin basic dye. Appl Nanosci. 2022;13(6):3925–3944. doi: 10.1007/s13204-022-02628-x.
- Verma V, Al-Dossari M, Singh J, et al. A review on green synthesis of TiO2 NPs: photocatalysis and antimicrobial applications. Polymers. 2022;14(7):1444. doi: 10.3390/polym14071444.
- Kashale AA, Gattu KP, Ghule K, et al. Biomediated green synthesis of TiO2 nanoparticles for lithium ion battery application. Compos B: eng. 2016;99:297–304. doi: 10.1016/j.compositesb.2016.06.015.
- Sett A, Gadewar M, Sharma P, et al. Green synthesis of gold nanoparticles using aqueous extract of dillenia indica. Adv Nat Sci: nanosci Nanotechnol. 2016;7(2):025005. doi: 10.1088/2043-6262/7/2/025005.
- Roopan SM, Bharathi A, Prabhakarn A, et al. Efficient phyto-synthesis and structural characterization of rutile TiO2 nanoparticles using annona squamosa peel extract. Spectrochim Acta A Mol Biomol Spectrosc. 2012;98:86–90. doi: 10.1016/j.saa.2012.08.055.
- Ismael M, Wark M. Perovskite-type LaFeO3: photoelectrochemical properties and photocatalytic degradation of organic pollutants under visible light irradiation. Catalysts. 2019;9(4):342. doi: 10.3390/catal9040342.
- Santhoshkumar T, Rahuman AA, Jayaseelan C, et al. Green synthesis of titanium dioxide nanoparticles using psidium guajava extract and its antibacterial and antioxidant properties. Asian Pac J Trop Med. 2014;7(12):968–976. doi: 10.1016/S1995-7645(14)60171-1.
- Hudlikar M, Joglekar S, Dhaygude M, et al. Green synthesis of TiO2 nanoparticles by using aqueous extract of jatropha curcas L. latex. Mater Lett. 2012;75:196–199. doi: 10.1016/j.matlet.2012.02.018.
- Hunagund SM, Desai VR, Kadadevarmath JS, et al. Biogenic and chemogenic synthesis of TiO 2 NPs via hydrothermal route and their antibacterial activities. RSC Adv. 2016;6(99):97438–97444. doi: 10.1039/C6RA22163G.
- Sundrarajan M, Bama K, Bhavani M, et al. Obtaining titanium dioxide nanoparticles with spherical shape and antimicrobial properties using M. citrifolia leaves extract by hydrothermal method. J Photochem Photobiol B. 2017;171:117–124. doi: 10.1016/j.jphotobiol.2017.05.003.
- Rajakumar G, Rahuman AA, Jayaseelan C, et al. Solanum trilobatum extract-mediated synthesis of titanium dioxide nanoparticles to control pediculus humanus capitis, hyalomma anatolicum anatolicum and anopheles subpictus. Parasitol Res. 2014;113(2):469–479. doi: 10.1007/s00436-013-3676-9.
- Sankar R, Rizwana K, Shivashangari KS, et al. Ultra-rapid photocatalytic activity of azadirachta indica engineered colloidal titanium dioxide nanoparticles. Appl Nanosci. 2015;5(6):731–736. doi: 10.1007/s13204-014-0369-3.
- Velayutham K, Rahuman AA, Rajakumar G, et al. Evaluation of catharanthus roseus leaf extract-mediated biosynthesis of titanium dioxide nanoparticles against hippobosca maculata and bovicola ovis. Parasitol Res. 2012;111(6):2329–2337. doi: 10.1007/s00436-011-2676-x.
- He F, Yu W, Fan X, et al. In vitro cytotoxicity of biosynthesized titanium dioxide nanoparticles in human prostate cancer cell lines. Trop J Pharm Res. 2018;16(12):2793–2799. doi: 10.4314/tjpr.v16i12.2.
- Rao KG, Ashok C, Rao KV, et al. Synthesis of TiO2 nanoparticles from orange fruit waste. Synthesis. 2015;2:1.
- Chatterjee A, Nishanthini D, Sandhiya N, et al. Biosynthesis of titanium dioxide nanoparticles using vigna radiata. Asian J Pharm Clin Res. 2016;9(4): 76–80.
- Samotyja U. Potato peel as a sustainable resource of natural antioxidants for the food industry. Potato Res. 2019;62(4):435–451. doi: 10.1007/s11540-019-9419-2.
- Garg P, Garg R. Qualitative and quantitative analysis of leaves and stem of tinospora cordifolia in different solvent extract. J Drug Delivery Ther. 2018;8(5-s):259–264. doi: 10.22270/jddt.v8i5-s.1967.
- Roghini R, Vijayalakshmi K. Phytochemical screening, quantitative analysis of flavonoids and minerals in ethanolic extract of citrus paradisi. Int J Pharm Sci Res. 2018;9:4859–4864.
- Ifemeje J, Egbuna C, Eziokwudiaso J, et al. Determination of the anti-nutrient composition of ocimum gratissimum, corchorus olitorius, murraya koenigii spreng and cucurbita maxima. Int J Innov Sci Res. 2014;3:127–133.
- Usman H, Abdulrahman FI, Usman A. Qualitative phytochemical screening and in vitro antimicrobial effects of methanol stem bark extract of ficus thonningii (moraceae). Afr J Trad Compl Alt Med. 2009; 6(3): 289–295. doi: 10.4314/ajtcam.v6i3.57178.
- Nishakavya S, Girigoswami A, Gopikrishna A, et al. Size attenuated copper doped zirconia nanoparticles enhances in vitro antimicrobial properties. Appl Biochem Biotechnol. 2022;194(8):3435–3452. doi: 10.1007/s12010-022-03875-y.
- Pallavi P, Harini K, Crowder S, et al. Rhodamine-conjugated anti-Stokes gold nanoparticles with higher ROS quantum yield as theranostic probe to arrest cancer and MDR bacteria. Appl Biochem Biotechnol. 2023;195(11):6979–6993. doi: 10.1007/s12010-023-04475-0.
- Agraharam G, Girigoswami A, Girigoswami K. Nanoencapsulated myricetin to improve antioxidant activity and bioavailability: a study on zebrafish embryos. Chemistry. 2021;4(1):1–17. doi: 10.3390/chemistry4010001.
- Stagner WC, Gaddam S, Parmar R, et al. Sucrose octaacetate. Profiles Drug Subst Excip Relat Methodol. 2019;44:267–291. doi: 10.1016/bs.podrm.2019.02.002.
- Rajakumar G, Rahuman AA, Roopan SM, et al. Fungus-mediated biosynthesis and characterization of TiO2 nanoparticles and their activity against pathogenic bacteria. Spectrochim Acta A Mol Biomol Spectrosc. 2012;91:23–29. doi: 10.1016/j.saa.2012.01.011.
- León A, Reuquen P, Garín C, et al. FTIR and Raman characterization of TiO2 nanoparticles coated with polyethylene glycol as carrier for 2-methoxyestradiol. Applied Sciences. 2017;7(1):49. doi: 10.3390/app7010049.
- Ibrahim TH, Chehade Y, Abou Zour M. Corrosion inhibition of mild steel using potato peel extract in 2M HCL solution. Int J Electrochem Sci. 2011;6(12):6542–6556. doi: 10.1016/S1452-3981(23)19699-4.
- Liao D, Wu G, Liao B. Zeta potential of shape-controlled TiO2 nanoparticles with surfactants. Colloids Surf, A. 2009;348(1–3):270–275. doi: 10.1016/j.colsurfa.2009.07.036.
- Homann T, Bredow T, Jug K. Adsorption of small molecules on the anatase (1 0 0) surface. Surf Sci. 2004;555(1-3):135–144. doi: 10.1016/j.susc.2003.12.039.
- El-Sherbiny S, Morsy F, Samir M, et al. Synthesis, characterization and application of TiO 2 nanopowders as special paper coating pigment. Appl Nanosci. 2014;4(3):305–313. doi: 10.1007/s13204-013-0196-y.
- Alonizan N, Chouiref L, Omri K, et al. Photocatalytic activity, microstructures and luminescent study of Ti-ZS: m nano-composites materials. J Inorg Organomet Polym. 2020;30(11):4372–4381. doi: 10.1007/s10904-020-01598-3.
- Roy J. The synthesis and applications of TiO2 nanoparticles derived from phytochemical sources. J Ind Eng Chem. 2022;106:1–19. doi: 10.1016/j.jiec.2021.10.024.
- Nunzi F, De Angelis F. Modeling titanium dioxide nanostructures for photocatalysis and photovoltaics. Chem Sci. 2022;13(33):9485–9497. doi: 10.1039/d2sc02872g.
- Anucha CB, Altin I, Bacaksiz E, et al. Titanium dioxide (TiO2)-based photocatalyst materials activity enhancement for contaminants of emerging concern (CECs) degradation: in the light of modification strategies. J Chem Eng Adv. 2022;10:100262. doi: 10.1016/j.ceja.2022.100262.
- Leroux MM, Doumandji Z, Chézeau L, et al. Toxicity of TiO2 nanoparticles: validation of alternative models. Int J Mol Sci. 2020;21(14):4855. doi: 10.3390/ijms21144855.
- Srinivasan M, Venkatesan M, Arumugam V, et al. Green synthesis and characterization of titanium dioxide nanoparticles (TiO2 NPs) using sesbania grandiflora and evaluation of toxicity in zebrafish embryos. Process Biochem. 2019;80:197–202. doi: 10.1016/j.procbio.2019.02.010.