Abstract
Lung cancer is a dangerous disease that is lacking in an ideal therapy. Here, we evaluated the anti-lung cancer effect in nude mice of a fully human single-chain antibody (scFv) against the associated antigen 7 transmembrane receptor (Ts7TMR), which is also called G protein-coupled receptor, between A549 cells and Trichinella spiralis (T. spiralis). Our data showed that anti-Ts7TMR scFv could inhibit lung cancer growth in a dose-dependent manner, with a tumour inhibition rate of 59.1%. HE staining did not reveal any obvious tissue damage. Mechanistically, immunohistochemical staining revealed that the scFv down-regulated the expression of PCNA and VEGF in tumour tissues. Overall, this study found that anti-Ts7TMR scFv could inhibit A549 lung cancer growth by suppressing cell proliferation and angiogenesis, which may provide a new strategy for treating lung cancer.
Introduction
Lung cancer presents the highest mortality and the number of lung cancer patients has been increasing yearly [Citation1]. Surgery, chemotherapy, and radiotherapy are commonly used therapies for lung cancer. However, these therapies either have significant adverse effects or do not act in some patients [Citation2], unveiling the need to develop novel therapies to treat lung cancer.
Emerging immunotherapies, such as monoclonal antibody therapy, cell therapy, and tumour vaccines, have been found to inhibit tumour growth by enhancing the body’s immune response. Most immunotherapies are currently in the research or preclinical testing phase, and few have been formally used in the clinic. The role of immunotherapies is limited by differences in tumour antigen expression in different patients [Citation3, Citation4]. Moreover, these therapies are often accompanied by adverse effects like cytokine release syndrome and neurotoxicity, as well as infections [Citation5].
Recent studies have found that some parasites, including Toxoplasma gondii (T. gondii), Trichinella spiralis (T. spiralis), and Plasmodium spp. can inhibit tumour growth [Citation6, Citation7]. Among them, the antitumour ability of T. spiralis is of great interest. So far, it has been found that T. spiralis suppresses the growth of melanoma, sarcoma, lung cancer, breast cancer, and liver cancer via mechanisms such as induction of apoptosis, regulation of immunity, or secretion of active substances [Citation8, Citation9]. T. spiralis excretory/secretory products (ESPs) inhibit tumour growth by promoting the maturation of immune cells such as dendritic cells and inducing apoptosis in tumour cells [Citation10, Citation11]. T. spiralis-derived antigens also have antitumour effects like crude extracts and A200711 protein [Citation12, Citation13]. In addition, T. spiralis infection can alter gene expression in tumours. One study found that the expression of cancer suppressor genes RpL41, NKTR, Rbbp4, and ANXA2 was significantly elevated in Sp2/0 myeloma in mice infected with T. spiralis [Citation14].
Several reports suggest that the antitumour effects of T. spiralis are related to the associated antigens between tumour cells and T. spiralis [Citation15,Citation16]. Additionally, some researches unveil that mouse-derived polyclonal antibodies and monoclonal antibodies against these associated antigens can also inhibit tumour growth [Citation17, Citation18]. However, the therapeutic effect of murine-derived antibodies in humans is attenuated owing to inducing human anti-mouse antibody (HAMA) responses [Citation19], making their future clinical application challenging. Therefore, using humanized antibodies to avoid HAMA responses appears to be a favourable alternative. ScFv has the advantages of small molecular weight, high penetration, short half-life in vivo, low immunogenicity, and the ability to be prepared in large quantities in prokaryotic expression systems. This specificity and low immunogenicity make scFv a positive alternative to traditional forms of therapy, improving the accuracy of targeting specific molecules and avoiding adverse side effects [Citation20]. Most CAR-T therapies target cancer cells by coupling scFvs that recognize and bind antigens on the surface of cancer cells [Citation21, Citation22]. However, the antitumour activity of scFv against associated antigens between tumour cells and T. spiralis remains to be studied.
The associated antigen 7 transmembrane receptor (7TMR), which is also known as G protein-coupled receptor (GPCR), between T. spiralis and A549 cells, was screened in our previous study. In addition, we previously prepared anti-Ts7TMR serum and scFv and found that anti-Ts7TMR scFv binds to the cell membrane of A549 cells, which also indicates that 7TMR is expressed in the cell membrane of A549 cells [Citation23]. In this study, the effects of anti-Ts7TMR serum on the growth of A549 lung cancer were firstly investigated in A549 tumour-bearing nude mice, and then the effects of anti-Ts7TMR scFv on the proliferation of A549 cells in vitro and on the growth of A549 lung cancer in vivo were further investigated. This study may pave the way for further exploration and development of targeted therapies against lung cancer and other cancers.
Materials and methods
Parasites, cells, and animal
Trichinella spiralis (strain ISS 534) was gifted by Prof. Mingxin Song of Northeast Agricultural University and was passaged in three Wistar rats in our laboratory. A549 cells (purchased from the Cell Bank of the Chinese Academy of Sciences and cryopreserved in liquid nitrogen in our laboratory) were cultured in the RPMI-1640 medium containing 10% foetal bovine serum and 100 µg penicillin- streptomycin. Thirty-six BALB/c nude mice (6–8 weeks old) were purchased from Beijing Vital River Laboratory Animal Technology Co. Ltd (Beijing, China). Mice were housed in isolation cages in the animal house of Jilin University Laboratory Animal Centre and provided with sterile food and water, as well as light and darkness for 12 h. Mice were euthanized by intraperitoneal injection of Sodium phenobarbital (50 mg/Kg) to relieve suffering.
Bioinformatics analysis of Ts7TMR
The Ts7TMR gene sequence screened in our previous work was identical to that in the NCBI database, so the amino acid sequence of the Ts7TMR protein was downloaded from NCBI for prediction and analysis (Appendix A). The molecular weight, isoelectric point, and other basic physicochemical properties of Ts7TMR protein were analyzed using ExpasyPro Param.
Therapeutic assays of anti-Ts7TMR serum
A suspension containing 2 × 106 A549 cells was injected subcutaneously into the axilla of the forelimb of nude mice for tumour challenge. After the tumours became palpable, we categorized the nude mice into three groups based on tumour volume, and there was no significant difference in tumour volume among the groups. One group was subcutaneously injected with 200 µL anti-Ts7TMR serum, and the other two groups were subcutaneously injected with anti-T. spiralis serum and PBS, respectively. Treatments were performed at one-day intervals for a total of five doses. Two days after the last treatment, the mice were executed and the tumour tissue was sampled. The tumour inhibition rate was calculated as Inhibition rate = (1-T/C) 100%, where T and C were the mean tumour weight of the experimental and PBS groups, respectively.
Screening of anti-Ts7TMR scFv
Anti-Ts7TMR scFv was obtained by screening the Tomlinson I + J phage human single-chain antibody library (MRC-LMB, Cambridge, UK) in our laboratory previously, which was found to bind to the A549 cell membrane [Citation23] (Appendix B).
Cell proliferation assay
6 × 103 A549 cells were co-incubated with different doses of scFvs (5, 15, and 45 μg). The equal volume of the RPMI-1640 medium was regarded as a negative control. After 48 h incubation, 10 µL Cell Counting Kit-8 (CCK-8, FC101-02, TransGen Biotech) solution was added and incubated for 4 h. The optical density (OD) at 490 nm was measured. The proliferation inhibition rate was calculated by the formula: inhibition rate = (ODexperimental −ODblank)/(ODcontrol −ODblank). This experiment was repeated three times.
Therapeutic assays of anti-Ts7TMR scFv
A suspension containing 2 × 106 A549 cells was injected subcutaneously into the axilla of the forelimb of nude mice for tumour challenge. When the tumours were palpable, the nude mice were divided into six groups based on tumour volume, and there was no significant difference in tumour volume among the groups. The scFvs (25, 50, and 100 μg), an equal volume of anti-T. spiralis serum (titer 1:200000), negative serum, and PBS were injected subcutaneously around the tumours continuously for 10 days, respectively. Two days after the last treatment, nude mice were executed and tumour tissues were taken. Tumour volume was calculated by applying the formula: Volume (mm3) = 1/2 × (length × width2). The tumour inhibition rate was calculated as Inhibition rate = (1-T/C) 100%, where T and C were the mean tumour volume or weight of the experimental and PBS groups, respectively.
Immunohistochemical staining
Tumour tissues fixed in 4% formaldehyde solution were prepared into 5 µm paraffin sections. Sections were treated sequentially with 3% H2O2, sodium citrate buffer, and 5% goat serum. Then mouse anti-human PCNA monoclonal antibody (60097-1-Ig, Proteintech), mouse anti-human BCL-2 monoclonal antibody (60178-1-Ig, Proteintech), and mouse anti-human VEGF monoclonal antibody (66828-1-Ig, Proteintech) were added separately dropwise and incubated at 37 °C for 30 min. Then the sections were incubated with biotin-conjugated Goat anti-mouse IgG at 37 °C for 30 min. Next, Streptavidin Immunohistochemistry kits (KIT-9710, maxim biotechnologies) and diaminobenzidine (DAB-0031, maxim biotechnologies) were added dropwise in sequence. After staining with haematoxylin, the sections were sealed with neutral resin and observed under the microscope (OLYMPUS). The samples were scored depending on the intensity of the colour and the number of positive cells [Citation24]. The grade of staining intensity was divided into 0 (no colour), 1 (light yellow), 2 (light brown), or 3 (brown), and the grade of positive cells number was divided into 0 (<5%), 1 (5–25%), 2 (25–50%), 3 (51–75%), or 4 (>75%). The two grades are combined into four levels for judgement: 0-1 score (negative), 2 scores (positive), 3–4 scores (moderately positive), and over 5 scores (strongly positive).
Haematoxylin-eosin staining
Tissue samples were prepared into paraffin sections. The sections were soaked in haematoxylin for 5 min and rinsed with water. Then, it was immersed in 1% hydrochloric acid alcohol for 30 s and soaked in eosin for 3 min, and rinsed slowly with water. They were soaked in 70%, 80%, 90%, 100% alcohol, and xylene for 5 min. Finally, sections were sealed with neutral resin and observed under the microscope. Pathological damage was assessed on a scale from 0 to 3 based on the lesion (mild, moderate, and severe) using the method documented by Landmann M [Citation25].
Statistics analysis
All results were presented as mean ± SD. Statistical differences were completed by one-way ANOVA. All statistical analyses and figures were made by GraphPad Prism 8.00 (GraphPad InStat Software).
Results
Detailed characteristics of Ts7TMR
Ts7TMR protein consists of 320 amino acids. ExpasyPro Param analysis showed that Ts7TMR protein has a theoretical molecular weight of 36.88 KDa, a theoretical isoelectric point of 5.95, an aliphatic index of 92.66, and an instability index of 39.02, which makes it a stable protein. The average hydrophilic index was −0.127, which was a hydrophilic protein.
Anti-Ts7TMR serum inhibited the growth of A549 lung cancer in nude mice
To clarify the effect of anti-Ts7TMR serum on the growth of A549 lung cancer, we constructed tumour-bearing nude mice with A549 cells and assessed the effect of antiserum by comparing the tumour weight. The results showed that the tumour weight was significantly reduced in the anti-Ts7TMR serum treatment group compared with the PBS group (), and the inhibition rate reached up to 64.77% (), suggesting that the anti-Ts7TMR serum could inhibit the development of A549 lung cancer in vivo.
Table 1. Effect of anti-Ts7TMR serum on the growth of A549 lung cancer in nude mice.
Anti-Ts7TMR scFv inhibited the proliferation of A549 cells
The effect of anti-Ts7TMR scFv on A549 lung cancer cell proliferation was detected using the CCK-8 assay. The results showed that 5 μg anti-Ts7TMR scFv could significantly inhibit the proliferation of A549 cells. Furthermore, the inhibitory effect was more obvious when the dose of anti-Ts7TMR scFv was increased to 15 μg and 45 μg, indicating that the inhibition of A549 cell proliferation by anti-Ts7TMR scFv was dose-dependent ().
Figure 2. The effect of anti-Ts7TMR scFv on A549 lung cancer and its preliminary mechanism. A. The effect of anti-Ts7TMR scFv on the proliferation of A549 cells in vitro. A549 cells were co-incubated with different doses of anti-Ts7TMR scFv (5, 25, and 45 μg) for 48h to detect cell proliferation by CCK-8 assay. B. The effect of anti-Ts7TMR scFv on A549 lung cancer growth in nude mice. Nude mice were injected subcutaneously with A549 cells and then treated subcutaneously with PBS, negative serum (Ns), anti-T. spiralis serum (Ts), and different doses of anti-Ts7TMR scFv (25, 50, and 100 μg) around the tumour for 10 consecutive days. C. Scoring of PCNA, BCL-2, and VEGF expression in tumour tissues by immunohistochemistry (400 ×). Expression of PCNA, BCL-2, and VEGF in tumours treated with PBS, negative serum (Ns), anti-T. spiralis serum (Ts), and different doses of anti-Ts7TMR scFv (25, 50, and 100 μg). Scale bar = 20 μm. *p < 0.05, **p < 0.01.

Anti-Ts7TMR scFv inhibited the growth of A549 lung cancer in nude mice
The effect of anti-Ts7TMR scFv on lung cancer was evaluated by measuring the volume and weight of tumours. The results demonstrated that anti-Ts7TMR scFv could markedly suppress the growth of A549 lung cancer in a dose-dependent manner (). The mean tumour volume and weight inhibition rates were 13.25% and 8.02% in the 25 μg group, 38.73% and 40.56% in the 50 μg group, and 51.2% and 59.1% in the 100 μg group (). In addition, anti-T. spiralis serum also showed significant inhibition of A549 lung cancer growth ().
Table 2. Effect of anti-Ts7TMR scFv on the growth of A549 lung cancer in nude mice.
Anti-Ts7TMR scFv down-regulated the expression of PCNA and VEGF in tumour
Immunohistochemical staining was used to detect the expression of PCNA, VEGF, and Bcl-2 in tumours, and the expression scores are shown in . The results showed that 25 μg anti-Ts7TMR scFv had no meaningful effect on the expression of PCNA and VEGF (). While 50 and 100 μg anti-Ts7TMR scFv could dramatically down-regulate the expression of PCNA and VEGF (), suggesting that anti-Ts7TMR scFv could inhibit cell proliferation and angiogenesis to inhibit the growth of A549 lung cancer. However, anti-Ts7TMR scFv has no significant effect on the expression of Bcl-2. In addition, mouse anti-T. spiralis serum could significantly down-regulate the expression of PCNA, VEGF, and Bcl-2 ().
Anti-Ts7TMR scFv did not cause significant pathological damage
Haematoxylin and eosin staining was performed on the heart, liver, spleen, lung, and kidney of nude mice to observe whether anti-Ts7TMR scFv caused histopathological damage. The results are shown in . No distinct pathological changes such as haemorrhage, congestion, hyperaemia, and necrosis were observed in the heart, liver, spleen, lung, and kidney of nude mice in all groups, underscoring that anti-Ts7TMR scFv has certain safety.
Figure 3. Pathological observations in nude mice (100 ×). Pathological observation of heart, liver, spleen, lung, and kidney in tumour-bearing nude mice treated with PBS, negative serum (Ns), anti-T. spiralis serum (Ts), and different doses of anti-Ts7TMR scFv (25, 50, and 100 μg). Scale bar = 100 μm.

Discussion
Tumour antigens include tumour-specific antigens (TSAs) and tumour-associated antigens (TAAs). TAAs have abnormally elevated expression in tumour cells or are produced only at specific stages of differentiation, but are also present in normal tissues [Citation26]. The widespread presence of TAAs in cancer patients makes them a frequently used target for immunotherapy, but TAAs can trigger central and peripheral immune tolerance and thus be ineffective in some patients [Citation26]. TSAs are antigens that can be generated in tumours by various mechanisms such as genomic mutations, aberrant transcriptomic variants, and post-translational modifications and are not expressed in normal tissues [Citation27]. However, TSAs are patient-specific and cannot be used in numerous patients [Citation27]. Moreover, TSAs are difficult to predict and screen. Ts7TMR, used in this study, is an associated antigen between T. spiralis and A549 cells. It has been reported to identify a variety of tumour cell-associated antigens from T. spiralis, such as Sp2/0 cells, LLC cells, and MG-63 cells, which are xenoantigens that do not induce central and peripheral immune tolerance, and show good immunogenicity, and biologic agents like antiserums, and Lactobacillus vaccines prepared with these antigens have shown promising antitumour effects in mice [Citation7,Citation16,Citation17]. This study also found that anti-Ts7TMR serum had a significant inhibitory effect on the growth of A549 lung cancer. However, these antisera are of murine origin and their antitumour effect is diminished in humans.
Fully human scFvs have intact antigen-binding sites, which not only do not induce the HAMA response but also are easy to obtain and mass-produce via screening phage antibody library in vitro [Citation28,Citation29]. Hence, it is widely applied in the diagnosis of tumours. A study reports that the CDR region of the anti-human Hsp70 scFv is specifically bound to Hsp70 expressed in lung cancer, indicating that it could be used for diagnosis and immunotherapy of lung cancer [Citation30]. Lu et al. found that scFvC9 against bisecting N- glycans expressed by cancer cells can be used for diagnosis, imaging, and treatment of cancers expressing this antigen [Citation31]. Studies have also reported that scFv has also shown significant efficacy in the treatment of tumours. Anti-NCL scFv inhibited the proliferation and migration of MDA-MB-231 triple-negative breast cancer cells in vitro and growth in vivo by binding to nucleolin on the surface of cancer cells [Citation32]. Another study showed that anti-Reg3a scFv inhibited the survival, proliferation, migration, and invasion of LoVo and RKO cells in vitro, and inhibited the growth of colon cancer in nude mice [Citation33]. Comparably, our study showed that anti-Ts7TMR scFv could inhibit the proliferation of A549 cells in vitro and the growth of A549 lung cancer in vivo.
We found that anti-Ts7TMR scFv down-regulated VEGF expression in A549 lung cancer, unveiling that it is a potential VEGF inhibitor. Angiogenesis is a key hallmark of tumour growth and metastasis and is regulated by VEGF, vascular endothelial growth factor receptor, integrin αVβ3, and integrin α5β1 [Citation34]. VEGF can enhance endothelial cell survival and promote further development of new blood vessels by inducing endothelial cell proliferation, activation, and differentiation as well as up-regulating the expression of anti-apoptotic proteins such as Bcl-2, XIAP, and survivin [Citation35, Citation36]. Similar to our results, the scFv Fv-LDP-D3, targeting the epidermal growth factor receptor, inhibited oesophageal cancer by inducing apoptosis and inhibiting cell proliferation [Citation37]. Other mechanisms by which anti-Ts7TMR scFv inhibits the growth of A549 lung cancer, such as the regulation of natural and adaptive immunity, deserve further exploration. Monoclonal antibody therapy induces a few mild adverse reactions like mucositis and gastrointestinal symptoms, as well as life-threatening symptoms such as cytokine release syndrome, angiogenesis inhibition, immune-related adverse reactions, and central nervous system toxicity [Citation38, Citation39]. Lin et al. found that adenovirus KGHV500 carrying anti-p21ras scFv treating A549 lung cancer was not toxic to normal tissue [Citation40]. Similarly, this study found that no significant tissue damage was observed in anti-Ts7TMR scFv-treated nude mice, but changes at the molecular level deserve further research.
It is well known that tumour cells can escape immunity and develop drug resistance through various mechanisms, such as loss of tumour antigens through mutation and alternative splicing, induced T-cell exhaustion, and the creation of an immune-suppressive tumour microenvironment [Citation41,Citation42]. Therefore, antibodies against a single antigen tend to weaken in efficacy in these situations. There are three strategies to address these issues. First, the use of bi-specific antibodies, which are antibodies that target two different antigens, synergize to enhance anti-tumour immunity and reduce immune escape and drug resistance. The second strategy is to construct antibody-drug conjugates by conjugating highly potent cytotoxic drugs to highly specific monoclonal antibodies directed against cancer cell membrane antigens, which must be directed against antigens specific to lung cancer that are not expressed in normal tissues [Citation43]. The third is combination therapy, which has favourable efficacy in lung cancer. Combination therapies, such as platinum plus third-generation chemotherapeutic agents with or without bevacizumab, are now available for clinical first-line treatment of lung cancer [Citation44]. Similarly, we can utilise the above three strategies to enhance the efficacy of anti- Ts7TMR scFv in the future.
The anti-Ts7TMR scFv used in this study is of full human origin, which not only can be prepared in large quantities in vitro but also has a bright application prospect because of its small molecular weight and high tissue penetration. However, some problems still need to be solved. First, this study initially found that anti-Ts7TMR scFv could inhibit tumour angiogenesis, but the in-depth mechanism needs to be further investigated. Second, the safety of anti-Ts7TMR scFv also needs to be further determined. After these issues are resolved in the future, anti-Ts7TMR scFv will be available for clinical translation.
In conclusion, we first found that anti-Ts7TMR fully human scFv could inhibit the proliferation of A549 cells in vitro and the growth of A549 lung cancer in vivo in a dose-dependent manner by inhibiting cell proliferation and angiogenesis. Importantly, anti-Ts7TMR scFv did not induce significant pathological damage. This study may provide a basis for the screening and application of tumour-associated antigens in other parasites with tumour-suppressive ability, such as T. gondii, Neospora caninum, and Echinococcus granulosus, as well as a new strategy for the screening of other tumour- associated antigens and the treatment of tumours in the future.
Ethical approval
All animal experimental procedures were performed by the Regulations on the Administration of Affairs Concerning Experimental Animals approved by the State Council of the People’s Republic of China (1988.11.1) and approved by the Animal Welfare and Research Ethics Committee of Jilin University (SY202201109). This study adhered to the ARRIVE guidelines.
Authors’ contributions
TY, JW, FL, PG, JL, NZ, and XZ designed and carried out the experiments. PG, JL, and XZ performed the data analysis, result validation, and visualization. TY, JW, and FL drafted the manuscript. NZ and XZ revised and edited the manuscript. All authors approved the final version of the manuscript. In addition, I confirm that all the authors meet the criteria for authorship as per the ICMJE criteria.
Acknowledgements
We thank Prof. Mingxin Song of Northeastern Agricultural University for providing the T. spiralis and Dr. Haiying Zhang of the Key Laboratory of Pathobiology, Ministry of Education, Jilin University, for her guidance during the experiment.
Disclosure of interest
No potential conflict of interest was reported by the author(s).
Data availability statement
The datasets generated during the current study are available from the corresponding author upon reasonable request.
Additional information
Funding
References
- Sung H, Ferlay J, Siegel RL, et al. Global cancer statistics 2020: GLOBOCAN estimates of incidence and mortality worldwide for 36 cancers in 185 countries. CA Cancer J Clin. 2021;71(3):209–249. PMID: 33538338. doi: 10.3322/caac.21660.
- Skribek M, Rounis K, Tsakonas G, et al. Complications following novel therapies for non-small cell lung cancer. J Intern Med. 2022;291(6):732–754. PMID: 35032058. doi: 10.1111/joim.13445.
- Orlando EJ, Han X, Tribouley C, et al. Genetic mechanisms of target antigen loss in CAR19 therapy of acute lymphoblastic leukemia. Nat Med. 2018;24(10):1504–1506. PMID: 30275569. doi: 10.1038/s41591-018-0146-z.
- de Charette M, Marabelle A, Houot R. Turning tumour cells into antigen presenting cells: the next step to improve cancer immunotherapy? Eur J Cancer. 2016;68:134–147. PMID: 27755997. doi: 10.1016/j.ejca.2016.09.010.
- Sadek NL, Costa BA, Nath K, et al. CAR T-cell therapy for multiple myeloma: a clinical Practice-Oriented review. Clin Pharmacol Ther. 2023;114(6):1184–1195. PMID: 37750399. doi: 10.1002/cpt.3057.
- Asghari A, Nourmohammadi H, Majidiani H, et al. Promising effects of parasite-derived compounds on tumor regression: a systematic review of in vitro and in vivo studies. Environ Sci Pollut Res Int. 2022;29(22):32383–32396. PMID: 35146610. doi: 10.1007/s11356-021-17090-5.
- Yue T, Zhang X, Gong P, et al. Antitumor effect of invasive Lactobacillus plantarum delivering associated antigen gene sHSP between Trichinella spiralis and lewis lung cancer cells. Int Immunopharmacol. 2023;115:109708. PMID: 36638662. doi: 10.1016/j.intimp.2023.109708.
- Kang YJ, Jo JO, Cho MK, et al. Trichinella spiralis infection reduces tumor growth and metastasis of B16-F10 melanoma cells. Vet Parasitol. 2013;196(1–2):106–113. doi: 10.1016/j.vetpar.2013.02.021.PMID: 23499484.
- Liao C, Cheng X, Liu M, et al. Trichinella spiralis and tumors: cause, coincidence or treatment? Anticancer Agents Med Chem. 2018;18(8):1091–1099. PMID: 29173187. doi: 10.2174/1871520617666171121115847.
- Ding J, Liu X, Tang B, et al. Murine hepatoma treatment with mature dendritic cells stimulated by Trichinella spiralis excretory/secretory products. Parasite. 2020;27:47. PMID: 32692308. doi: 10.1051/parasite/2020045.
- Ding J, Tang B, Liu X, et al. Excretory- secretory product of Trichinella spiralis inhibits tumor cell growth by regulating the immune response and inducing apoptosis. Acta Trop. 2022;225:106172. PMID: 34627760. doi: 10.1016/j.actatropica.2021.106172.
- Wang XL, Fu BQ, Yang SJ, et al. Trichinella spiralis–a potential anti-tumor agent. Vet Parasitol. 2009;159(3-4):249–252. PMID: 19041180. doi: 10.1016/j.vetpar.2008.10.052.
- Wang XL, Liu MY, Sun SM, et al. An anti-tumor protein produced by Trichinella spiralis induces apoptosis in human hepatoma H7402 cells. Vet Parasitol. 2013;194(2-4):186–188. PMID: 23433602. doi: 10.1016/j.vetpar.2013.01.052.
- Deng B, Gong P, Li J, et al. Identification of the differentially expressed genes in SP2/0 myeloma cells from balb/c mice infected with Trichinella spiralis. Vet Parasitol. 2013;194(2-4):179–182. PMID: 23473833. doi: 10.1016/j.vetpar.2013.01.050.
- Duan L, Li J, Cheng B, et al. Identification of a novel gene product expressed by Trichinella spiralis that binds antiserum to Sp2/0 myeloma cells. Vet Parasitol. 2013;194(2-4):183–185. PMID: 23465747. doi: 10.1016/j.vetpar.2013.01.051.
- Gong P, Zhang J, Cao L, et al. Identification and characterization of myeloma-associated antigens in Trichinella spiralis. Exp Parasitol. 2011;127(4):784–788. PMID: 21232537. doi: 10.1016/j.exppara.2010.12.001.
- Yue TT, Zhang N, Li JH, et al. Anti-osteosarcoma effect of antiserum against cross antigen TPD52 between osteosarcoma and Trichinella spiralis. Parasit Vectors. 2021;14(1):498. PMID: 34565443. doi: 10.1186/s13071-021-05008-6.
- Zhang TJ, Pang DX, Zhang N, et al. Preparation of monoclonal antibody against Trichinella spiralis selenoprotein T and its inhibitory effect on hepatoma H7402 cells in nude mice. Chin J Biologicals January. 2020;33:42–44. (in Chinese).
- Sangpheak K, Waraho-Zhmayev D, Haonoo K, et al. Investigation of interactions between binding residues and solubility of grafted humanized anti-VEGF IgG antibodies expressed as full-length format in the cytoplasm of a novel engineered E. coli SHuffle strain. RSC Adv. 2021;11(11):6035–6048. PMID: 35423148. doi: 10.1039/D0RA08534K.
- Lu L, Wang X, Zhang A, et al. Selection and expression of CD40 single chain variable fragment by phage display and evaluation of tumor specific immune activation. Int Immunopharmacol. 2019;71:224–232. PMID: 30925323. doi: 10.1016/j.intimp.2019.03.020.
- Murayama Y, Kawashima H, Kubo N, et al. Effectiveness of 4-1BB-costimulated HER2-targeted chimeric antigen receptor T cell therapy for synovial sarcoma. Transl Oncol. 2021;14(12):101227. PMID: 34555727. doi: 10.1016/j.tranon.2021.101227.
- Noh KE, Lee JH, Choi SY, et al. TGF-β/IL-7 chimeric switch receptor-Expressing CAR-T cells inhibit recurrence of CD19-Positive B cell lymphoma. Int J Mol Sci. 2021;22(16):8706. PMID: 34445415. doi: 10.3390/ijms22168706.
- Wang JP, Zhang GL, Zhang HY, et al. Screening of A549 cells-associated scFv antibody against Trichinella spiralis antigen protein 7TR. Chin J Biol. 2017;30:488–492. (in Chinese).
- Ma Y, Ma L, Guo Q, et al. Expression of bone morphogenetic protein-2 and its receptors in epithelial ovarian cancer and their influence on the prognosis of ovarian cancer patients. J Exp Clin Cancer Res. 2010;29(1):85. PMID: 20587070. doi: 10.1186/1756-9966-29-85.
- Landmann M, Scheibner D, Graaf A, et al. A semiquantitative scoring system for histopathological and immunohistochemical assessment of lesions and tissue tropism in avian influenza. Viruses. 2021;13(5):868. PMID: 34065126. doi: 10.3390/v13050868.
- Xie N, Shen G, Gao W, et al. Neoantigens: promising targets for cancer therapy. Signal Transduct Target Ther. 2023;8(1):9. PMID: 36604431. doi: 10.1038/s41392-022-01270-x.
- Li J, Xiao Z, Wang D, et al. The screening, identification, design and clinical application of tumor-specific neoantigens for TCR-T cells. Mol Cancer. 2023;22(1):141. PMID: 37649123. doi: 10.1186/s12943-023-01844-5.
- Mahati S, Fu X, Ma X, et al. Delivery of miR-26a using an exosomes-based nanosystem inhibited proliferation of hepatocellular carcinoma. Front Mol Biosci. 2021;8:738219. PMID: 34552961. doi: 10.3389/fmolb.2021.738219.
- Peng X-H, Wang Y, Huang D, et al. Targeted delivery of cisplatin to lung cancer using ScFvEGFR-heparin-cisplatin nanoparticles. ACS Nano. 2011;5(12):9480–9493. PMID: 22032622. doi: 10.1021/nn202410f.
- Vostakolaei MA, Molavi O, Hejazi MS, et al. Isolation and characterization of a novel scFv antibody fragments specific for Hsp70 as a tumor biomarker. J Cell Biochem. 2019;120(9):14711–14724. PMID: 30998271. doi: 10.1002/jcb.28732.
- Lu Z, Kamat K, Johnson BP, et al. Generation of a fully human scFv that binds tumor-specific glycoforms. Sci Rep. 2019;9(1):5101. PMID: 30911061. doi: 10.1038/s41598-019-41567-6.
- Palmieri D, Richmond T, Piovan C, et al. Human anti-nucleolin recombinant immunoagent for cancer therapy. Proc Natl Acad Sci U S A. 2015;112(30):9418–9423. PMID: 26170308. doi: 10.1073/pnas.1507087112.
- Du P, Wang X, Yin T, et al. Anti- tumor effect of single-chain antibody to Reg3a in colorectal cancer. Exp Cell Res. 2020;396(1):112278. PMID: 32918897. doi: 10.1016/j.yexcr.2020.112278.
- Scott AM, Wolchok JD, Old LJ. Antibody therapy of cancer. Nat Rev Cancer. 2012;12(4):278–287. PMID: 22437872. doi: 10.1038/nrc3236.
- Ludwig DL, Pereira DS, Zhu Z, et al. Monoclonal antibody therapeutics and apoptosis. Oncogene. 2003;22(56):9097–9106. PMID: 14663488. doi: 10.1038/sj.onc.1207104.
- Muratori L, Gnavi S, Fregnan F, et al. Evaluation of vascular endothelial growth factor (VEGF) and its family member expression after peripheral nerve regeneration and denervation. Anat Rec. 2018;301(10):1646–1656. PMID: 29710417. doi: 10.1002/ar.23842.
- He S, Zhao C, Tao H, et al. A recombinant scFv antibody-based fusion protein that targets EGFR associated with IMPDH2 downregulation and its drug conjugate show therapeutic efficacy against esophageal cancer. Drug Deliv. 2022;29(1):1243–1256. PMID: 35416106. doi: 10.1080/10717544.2022.2063454.
- Bugelski PJ, Achuthanandam R, Capocasale RJ, et al. Monoclonal antibody-induced cytokine-release syndrome. Expert Rev Clin Immunol. 2009;5(5):499–521. PMID: 20477639. doi: 10.1586/eci.09.31.
- Kroschinsky F, Stölzel F, von Bonin S, et al. New drugs, new toxicities: severe side effects of modern targeted and immunotherapy of cancer and their management. Crit Care. 2017;21(1):89. PMID: 28407743. doi: 10.1186/s13054-017-1678-1.
- Lin XR, Zhou XL, Feng Q, et al. CIK cell- based delivery of recombinant adenovirus KGHV500 carrying the anti-p21Ras scFv gene enhances the anti-tumor effect and safety in lung cancer. J Cancer Res Clin Oncol. 2019;145(5):1123–1132. PMID: 30796510. doi: 10.1007/s00432-019-02857-8.
- Li YR, Halladay T, Yang L. Immune evasion in cell-based immunotherapy: unraveling challenges and novel strategies. J Biomed Sci. 2024;31(1):5. PMID: 38217016. doi: 10.1186/s12929-024-00998-8.
- Rabinovich GA, Gabrilovich D, Sotomayor EM. Immunosuppressive strategies that are mediated by tumor cells. Annu Rev Immunol. 2007;25(1):267–296. PMID: 17134371. doi: 10.1146/annurev.immunol.25.022106.141609.
- Passaro A, Jänne PA, Peters S. Antibody-drug conjugates in lung cancer: recent advances and implementing strategies. J Clin Oncol. 2023;41(21):3747–3761. PMID: 37224424. doi: 10.1200/jco.23.00013.
- Reck M, Garassino MC, Imbimbo M, et al. Antiangiogenic therapy for patients with aggressive or refractory advanced non-small cell lung cancer in the second-line setting. Lung Cancer. 2018;120:62–69. doi: 10.1016/j.lungcan.2018.03.025.
Appendix A
1 Ts7TMR mRNA (transcript_id: XM_003370198.1)
ATGACTTCCGAATTGAATTGTGATGCTGTTTTTGAAATGAAAAACTTGTATTACACGGGAAATTACTATGACTGCTCATTGAAAGCGACAAAATTAAAGGAGAAAACGACGTCTATCGATGTGAAATTGCAGTGCGATTATTTTATCCATCGTAGCAATATAGCACAAGGAATGTCTTCTATTACACTCGCAGATATTTCATCCAGTGAAACATCTCCGGAATTGTTTGCAGTTCGCATGGTAGCAGAATATTTAGAAAAGACAGAAAAGAGGCAATGCACTTATTTATACATTATATTACTTTCACATTACATTGTAATTCATGTCTATTATAGAGCAGATATTGTCCAGAATTTTACAAAAATAGCGAATCGTTTTCACTGGAATTCAGCTGTCATTTTTGCTATTGCAAAAATTTTGCATCACGAACAAAAATATGAAGATAGTCTGCGTTATCTTCATGTTTGTGGGAAATCATTAGACTGCTTGTACTTGACTACACTTTCACTTTTGGCAATTGGTCAAGTAGTTGAAGCAAAGGAAACGGTCGCGAAAATGCAGAGACTAGATGATGATAATGTCATTGCTCACATGGCTTCAGCTGCAGTGAAAATGTTTTTGGGAAATGAAAATATCCAAGACGCATTTTACATTTTTAAAGAGTTACAAGAAAAATATGGTTCTTCTGCGCTGTTGCAAAATGCAGTTTTGTGTTGTTTTGTATTACAAGGGAAATATGGGGAAGCGGAGGAAATAGCCAATGACAATATGAAAAACTTTTCTCAGAACCCCGAAGTATTCATCAACGCAATTGTTGTATCTCTTATTCAAGGAAAATCTTATGAGACTGTCAAACGCTACGTGCATTTGATGAAAGAAAAATTCGCATGCCATCTGTGGACAACAGATTTAAAGGAAAAAGAAAACGAATTTGATAGACTTTGTGAAACGTTCACTACATAG
2 The amino acid sequence of Ts7TMR (XP_003370246.1)
MTSELNCDAVFEMKNLYYTGNYYDCSLKATKLKEKTTSIDVKLQCDYFIHRSNIAQGMSSITLADISSSETSPELFAVRMVAEYLEKTEKRQCTYLYIILLSHYIVIHVYYRADIVQNFTKIANRFHWNSAVIFAIAKILHHEQKYEDSLRYLHVCGKSLDCLYLTTLSLLAIGQVVEAKETVAKMQRLDDDNVIAHMASAAVKMFLGNENIQDAFYIFKELQEKYGSSALLQNAVLCCFVLQGKYGEAEEIANDNMKNFSQNPEVFINAIVVSLIQGKSYETVKRYVHLMKEKFACHLWTTDLKEKENEFDRLCETFTT
Appendix B
1 Prokaryotic expression of Ts7TMR (Reference [Citation23]: Wang JP, Zhang GL, Zhang HY, et al. Screening of A549 cells-associated scFv antibody against Trichinella spiralis antigen protein 7TR. Chin J Biol. 2017;30:488–492 (in Chinese).).
Primers were designed according to the Ts7TMR gene sequence accessed in GenBank (XM003370198. 1). Forward primer sequence: 5′-CCTGGATCCATGACTTCCGAATTGAAT-3′ (Italics represent digestion site BamHI), Reverse primer sequence: 5′-CCGGAATTCTATGTAGTGAACGTTTCAC-3′ (Italics represent digestion site EcoRI). The target fragment was amplified by PCR using Trichinella spiralis muscle larvae cDNA as a template. The PCR product was purified and ligated to pET-32a and transformed into E. coli BL21, and then sequenced. After induction of the sequenced correct recombinant strain with 1 mM IPTG, the recombinant protein Ts7TMR was purified by Ni purification column.
A 900-bp gene was amplified by PCR, which was consistent with expectations (). It was inserted into the expression plasmid pET-32a and transformed into competent E. coli BL-21 cells. Restriction digestion and sequencing showed that the expression vector was successfully constructed (). SDS-PAGE revealed that the size of the fusion protein Ts7TMR was approximately 50 KDa ().
Figure B1. Construction of the Ts7TMR prokaryotic expression vector. A. Using T. spiralis cDNA as a template, the Ts7TMR gene was amplified by PCR and identified. B. The recombinant pET-32a- Ts7TMR plasmid was characterised by restriction digestion Lane 1: pET-32a- Ts7TMR. Lane 2: pET-32a. C. The purified fusion protein Ts7TMR was identified by SDS-PAGE. Lanes 1: Crude purification of Ts7TMR. Lane 2: Refined purification of Ts7TMR.

2 Screening of anti-Ts7TMR scFv (Reference [Citation23]: Wang JP, Zhang GL, Zhang HY, et al. Screening of A549 cells-associated scFv antibody against Trichinella spiralis antigen protein 7TR. Chin J Biol. 2017;30:488–492. (in Chinese).
The scFv against the associated antigen Ts7TMR was obtained by screening the Tomlinson I + J phage human single-chain antibody library (MRC-LMB, Cambridge, UK) in our laboratory previously. The primary antibody library was 1.51 × 107CFU/mL, and the primary antibody library was screened in 4 rounds by microtiter plate screening method using Ts7TMR as antigen. The phage output after each round of screening was 4.68 × 105 PFU/mL, 2.43 × 106 PFU/mL, 5.77 × 105 PFU/mL and 4.73 × 106 PFU/mL, respectively. IPTG-induced expression of the strains obtained from the fourth round of screening was performed to obtain scFv, PBS was used as a negative control, and scFv bound to A549 cell lysate was screened by ELISA using A549 cell lysate as an antigen. The final screening obtained a positive strain, which was induced by IPTG and purified by affinity chromatography with rProtein-A Sepharose FF (GE Healthcare, Little Chalfont, UK). SDS-PAGE and Western blot were then performed to characterise the scFv. The results indicated that the relative molecular mass of anti-Ts7TMR scFv was about 31 KDa and that it could bind specifically to Ts7TMR ().
Figure B2. SDS-PAGE and western blot analysis of anti-Ts7TMR scFv. A. SDS-PAGE analysis of molecular weight and purity of anti-Ts7TMR scFv. B. Western blot identification of binding of anti-Ts7TMR scFv to Ts7TMR.
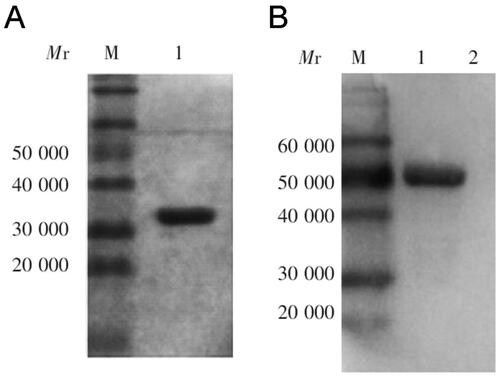
3 Anti-Ts7TMR scFv binds to the cell membrane of A549 cells (Reference [Citation23]: Wang JP, Zhang GL, Zhang HY, et al. Screening of A549 cells-associated scFv antibody against Trichinella spiralis antigen protein 7TR. Chin J Biol. 2017;30:488–492. (in Chinese).
A549 cells were seeded into 24-well plates, cultured overnight, then fixed in methanol, softened with 0.5% Triton X-100 with 0.5% Triton X-100 and blocked with 5% skim milk powder. Cells were incubated overnight with anti-Ts7TMR scFv as the primary antibody and then treated with Protein A full-length protein-FITC. Cells were then stained with DAPI and visualised by laser confocal microscopy. Laser confocal results show that anti-Ts7TMR scFv binds to the cell membrane of A549 cells ().