Abstract
Objective
The objective of this study was to test the therapeutic effect of carbon monoxide polyhemoglobin (polyCOHb) in haemorrhagic shock/resuscitation and its underlying mechanisms.
Methods
48 rats were divided into two experimental parts, and 36 rats in the first experiment and 12 rats in the second experiment. In the first experimental part, 36 animals were randomly assigned to the following groups: hydroxyethyl starch group (HES group, n = 12), polyhemoglobin group (polyHb group, n = 12), and carbon monoxide polyhemoglobin group (polyCOHb group, n = 12). In the second experimental part, 12 animals were randomly assigned to the following groups: polyHb group (n = 6), and polyCOHb group (n = 6). Then the anaesthetised rats were haemorrhaged by withdrawing 50% of the animal’s blood volume (BV), and resuscitated to the same volume of the animal’s withdrawing BV with HES, polyHb, polyCOHb. In the first experimental part, the 72h survival rates of each groups animals were measured. In the second experimental part, the rats’ mean arterial pressure (MAP), heart rate (HR), blood gas levels and other indicators were dynamically monitored in baseline, haemorrhagic shock (HS), at 0point resuscitation (RS 0h) and after 1 h resuscitation (RS 1h). The concentrations of malondialdehyde (MDA), superoxide dismutase (SOD), tumour necrosis factor-alpha (TNF-alpha) and interleukin-6 (IL-6) were measured by ELISA kits in both groups of rats at RS 1h. Changes in pathological sections were examined by haematoxylin-eosin (HE) staining. Nuclear factor erythroid 2-related factor 2 (Nrf2) and haem oxygenase-1 (HO-1) levels were detected by immunohistochemical analysis, while myeloperoxidase (MPO) levels were detected by immunofluorescence. DHE staining was used to determine reactive oxygen species (ROS) levels.
Results
The 72h survival rates of the polyHb and polyCOHb groups were 50.00% (6/12) and 58.33% (7/12) respectively, which were significantly higher than that of the 8.33% (1/12) in the HES group (p < 0.05). At RS 0h and RS 1h, the HbCO content of rats in the polyCOHb group (1.90 ± 0.21, 0.80 ± 0.21) g/L were higher than those in the polyHb group (0.40 ± 0.09, 0.50 ± 0.12)g/L (p < 0.05); At RS 1h, the MDA (41.47 ± 3.89 vs 34.17 ± 3.87 nmol/ml) in the plasma, Nrf2 and HO-1 content in the colon of rats in the polyCOHb group were lower than the polyHb group. And the SOD in the plasma (605.01 ± 24.46 vs 678.64 ± 36.37) U/mg and colon (115.72 ± 21.17 vs 156.70 ± 21.34) U/mg and the MPO content in the colon in the polyCOHb group were higher than the polyHb group (p < 0.05).
Conclusions
In these haemorrhagic shock/resuscitation models, both polyCOHb and polyHb show similar therapeutic effects, and polyCOHb has more effective effects in maintaining MAP, correcting acidosis, reducing inflammatory responses than that in polyHb.
Introduction
Patients with acute haemorrhagic shock may suffer from multiple organ dysfunctions due to significant blood loss within a short period of time, which results in a decrease in the effective circulating blood volume, tissue ischaemia, hypoxia, metabolic disorders, and even life-threatening situations [Citation1]. In the context of acute haemorrhagic shock, it is crucial to initiate standard resuscitation procedures in the prehospital administration, which can help reduce the risk of complications from bleeding and shock [Citation2]. Therefore, timely and effective fluid resuscitation and increased oxygen supply are essential for treatment [Citation3]. Blood and blood products play a crucial role in the treatment of acute haemorrhagic shock. However, the Coronavirus Disease 2019 (COVID-19) pandemic and the ageing population have exacerbated the imbalance between blood supply and demand, presenting challenges in maintaining a sufficient blood supply [Citation4]. Therefore, prehospital first aid requires a readily available oxygen-carrying product with a capacity expansion effect. Studies [Citation5,Citation6] have shown that haemoglobin-based oxygen carriers (HBOCs) possess the necessary characteristics, such as timely availability, oxygen-carrying capacity, expandability, and a nanometre particle size, to serve as substitutes for red blood cells.
HBOCs are haemoglobin derivatives extracted from red blood cells. They lack the protection of red blood cell membranes and redox systems, making haemoglobin easily oxidised to methaemoglobin (MetHb). MetHb not only lacks oxygen-carrying function but also poses toxicity to the body. To prevent the production of MetHb, HBOCs should be prepared and stored at low temperatures without exposure to oxygen. To reduce the oxidation of HBOCs during preparation, the teams conducted research in two areas mainly. Firstly, they improved the molecular structure of HBOCs by cross-linking antioxidant enzymes and small-molecule antioxidants. Secondly, small molecule antioxidants such as vitamin C (Vc) and reduced glutathione were added to the haemoglobin solution to enhance its antioxidant capacity [Citation7–10]. The generation of MetHb during the preparation and preservation processes cannot be completely eliminated despite the implementation of the necessary measures. This can pose several challenges in both stages. To optimise the preparation process, one way to avoid oxidation of haemoglobin is to use a gas that cannot be reduced instead of the oxygen that occupies a position in haemoglobin.
Carbon monoxide (CO) can bind to haemoglobin efficiently. It binds to the same site as oxygen and prevents the oxidation of HBOCs in vitro. This is due to its affinity for haemoglobin, which is 220 times greater than that of O2, effectively replacing the oxygen-carrying capacity of haemoglobin [Citation11]. In addition, CO has two sides: it has very promising therapeutic uses at low doses and is toxic at high doses [Citation12]. Nagao et al. [Citation13,Citation14] introduced CO-haemoglobin capsules and discovered its effectiveness in treating acute pancreatitis, colitis, and other diseases. Studies [Citation3,Citation15] have also demonstrated the ability of pegylated carboxyhaemoglobin (PEGCOHb) to enhance microcirculation and successfully maintain the oxygen supply. The researches of polymerised human cord haemoglobin (polyHb), one kind of the HBOCs, had demonstrated that it was safe and had a good capacity to carry oxygen [Citation16,Citation17]. The previous research conducted by our team discovered that using glutaraldehyde as a crosslinking agent in the preparation of carbon monoxide polymeric haemoglobin (polyCarboxyhemoglob, polyCOHb) can streamline the process of preparing HBOCs. However, polyCOHb is still unclear whether can have an anti-haemorrhagic shock effect. This article would explore the role of polyCOHb in the treatment of acute haemorrhagic shock and provide a scientific rationale for its application.
Materials and methods
General methods and design of this experiment
48 rats were divided into two experimental parts, and 36 rats in the first experiment and 12 rats in the second experiment. In the first experimental part, 36 animals were randomly assigned to the following groups: hydroxyethyl starch group (HES group, n = 12), polyhemoglobin group (polyHb group, n = 12), and carbon monoxide polyhemoglobin group (polyCOHb group, n = 12). In the second experimental part, 12 animals were randomly assigned to the following groups: polyHb group (n = 6), and polyCOHb group (n = 6). Then the anaesthetised rats were haemorrhaged by withdrawing 50% of the animal’s blood volume (BV), and resuscitated to the same volume of the animal’s withdrawing BV with HES, polyHb, polyCOHb. In the first experimental part, the 72h survival rates of each groups animals were measured. In the second experimental part, the rats’ mean arterial pressure (MAP), heart rate (HR), blood gas levels and other indicators were dynamically monitored in baseline, haemorrhagic shock (HS), at 0point resuscitation (RS 0h) and after 1 h resuscitation (RS 1h). The concentrations of malondialdehyde (MDA), superoxide dismutase (SOD), tumour necrosis factor-alpha (TNF-alpha), interleukin-6 (IL-6), Nuclear factor erythroid 2-related factor 2 (Nrf2), haem oxygenase-1 (HO-1), reactive oxygen species (ROS), myeloperoxidase (MPO) levels were measured to test the experiment effect between two groups.
This study met the review requirements of the Ethics Review Committee of the Institute of Blood Transfusion, Chinese Academy of Medical Sciences (NO.2023055).
Preparation of PolyHb
The polyHb used in this study was provided by the Institute of Blood Transfusion, Chinese Academy of Medical Sciences. In summary, the preparation procedure involved extracting human cord blood, the human umbilical cord blood used in this study was derived from waste blood that had been extracted for stem cells from SICHUAN NEO-LIFE STEMCELL BIOTECH INC. First, the red blood cells were obtained by washing the blood with a 0.9% sodium chloride solution. And then, the purified haemoglobin was obtained by rupturing red blood cells with a hypotonic solution. Finally, PolyHb was obtained by adding cross-linking agents into the haemoglobin solution, and filtering and ultrafiltering [Citation18].
Preparation of PolyCOHb
The polyCOHb used in this study was provided by the Institute of Blood Transfusion, Chinese Academy of Medical Sciences. In summary, the preparation procedure involved extracting human cord blood, the human umbilical cord blood used in this study was derived from waste blood that had been extracted for stem cells from SICHUAN NEO-LIFE STEMCELL BIOTECH INC. The carboxyhaemoglobin was first prepared by adding CO gas to the purified human cord haemoglobin solution. Subsequently, the polyCOHb was obtained by polymerising carboxyhaemoglobin, following the steps outlined for the preparation of PolyHb [Citation18].
Preparation of HES
The HES used in this study was purchased from Beijing Fresenius Kabi Co., Ltd., with the trade name of Voluven, with an average molecular weight of 130.000 and a concentration of 6%, and an approval number: Guoyao Zhun Zi H20103246.
Animal preparation
Studies were performed in 48 SPF grade male SD rats, 6-8 weeks old, weighing (245.0 ± 15.0) g. They were provided by Beijing Vital River Laboratory Animal Technology Co., Ltd. (animal production licence number: SCXK [JING] 2021-0011). The rats were growing up in a 12h light/12h dark atmosphere, keeping the temperature between 22 °C-26 °C and the humidity between 40%-70%, replenishing every day, free food and beverages. 12h of fasting prior to the trial, followed by adaptive eating for a week. These animals handing and care followed the Guide for the Care and Use of Laboratory Animals [Citation19].
Inclusion criteria
Animals were suitable for experiments if: (i) mean arterial blood pressure (MAP) was above 100 mm Hg at baseline, (ii) systemic Hb was above 12 g/dL at baseline, and (iii) animals survived the shock period.
Haemorrhagic shock/resuscitation protocol
An intraperitoneal injection of 2.0% sodium pentobarbital (Sigma, analytically pure) at a dose of 0.20 ml/100g body weight was used to anaesthetise male SD rats. After administering anaesthesia, the rats were placed in a supine position and secured to the operating table. The femoral artery and femoral vein of the rats were then separated. The animals prepared with left femoral vein, left femoral artery and right femoral artery catheterisation. After surgical instrumentation, animals were allowed to rest for 30 min to stabilise before baseline measurements were taken. Following baseline measurements, approximately 0.30 ml of heparin sodium solution (500 U/kg, Tianjin Biochemical Pharmaceutical Co., Ltd. Approval number: Guoyao Zhun Zi H12020505) was injected through the femoral artery for anticoagulation. The left femoral vein was used for fluid resuscitation, and the right femoral artery was used to monitor HR and MAP, and the left femoral artery was used to haemorrhagic. Then the anaesthetised rats were haemorrhaged by withdrawing 50% of the animal’s blood volume (BV), estimated as 6% body weight + 0.77 ml, via the femoral artery catheter into syringes. The haemorrhagic shock phase was divided by two steps. Step 1 involved withdrawing 30% of the animal’s BV from the veins. After a rest steadily for 10 min, step 2 was performed, which involved drawing an additional 20% of the animal’s BV from the veins. The withdrawing velocity during the haemorrhagic shock process is 1.0 ml/min. Hypovolemic shock was maintained for 30 min. Resuscitation was implemented by infusion of room temperature resuscitation solution through the femoral venous catheter until the animal recovered to the same volume of the animal’s withdrawing BV at a constant rate of 1.0 ml/min. A microsyringe pump (GenieTouch, USA) was used in the haemorrhagic Shock/resuscitation procedure.
Experimental grouping and protocol
48 rats were divided into two experimental parts, and 36 rats in the first experiment and 12 rats in the second experiment.
The first experiment
36 animals were randomly assigned to the following groups: hydroxyethyl starch group (HES group, n = 12), polyhemoglobin group (polyHb group, n = 12), and carbon monoxide polyhemoglobin group (polyCOHb group, n = 12). After the hypovolemic shock maintained for 30 min, resuscitation was implemented by infusion of room temperature resuscitation solution. Regimen for resuscitation: ①The HES group: 6.0% HES was infused for resuscitation following the preparation of a 50% haemorrhagic shock model. ②The polyHb group: 3.0% polyHb (Hb30g/L) resuscitation solution was prepared by mixing 6.0% polyHb and 6.0% HES 1:1 by volume. ③The polyCOHb group prepared a 3.0% poly-COHb (COHb30g/L) resuscitation solution by mixing 6.0% poly-COHb and 6.0% HES 1:1 by volume. The 72-h survival ratio was recorded. At the end of this studies, the animals were euthanized by CO2 inhalation euthanasia method.
The second experiment
In the second experimental part, 12 animals were randomly assigned to the following groups: polyHb group (n = 6), and polyCOHb group (n = 6). The haemorrhagic shock/resuscitation protocol was same to the first experiment.
Using a multi-channel physiological recorder (Powerlab ML880, Australia), the mean arterial pressure (MAP) and heart rate (HR) were dynamically monitored at baseline (Baseline), end shock (SH), 0 point of resuscitation (RS 0h), and 1 h of resuscitation (RS 1h). Furthermore, 0.2 ml of blood was drawn at baseline, SH, RS 0h, and RS 1h. A blood gas analyser (GEM Premier 3000, USA) was used to detect the blood gas indices, including pH, glucose (Glu), lactic acid (Lac), carbon dioxide partial pressure (PaCO2), oxygen partial pressure (PaO2), and base excess in extracellular fluid (BEecf). A blood oximeter (GEM-OPL, USA) was used to assess the blood oxygen levels in the two groups of rats, which included THb (total haemoglobin concentration), HbO2 (oxygenated haemoglobin content), HbCO (carboxyhaemoglobin content), HbO2% (percentage of HbO2 to total haemoglobin), and HbCO% (proportion of HbCO to total haemoglobin). In the plasma and colon of the rats, the following parameters were measured: malondialdehyde (MDA), superoxide dismutase (SOD), tumour necrosis factor-α (TNF-α), and interleukin-6 (IL-6) using the enzyme-linked immunosorbent assay (ELISA) kit. from BioRender.com depicts the schematic diagram of the experimental grouping and experimental procedure.
Haematoxylin and eosin (HE) staining of rat Colon tissue
Rat colon tissue was fixed with a 4% paraformaldehyde solution for 24 h after 1 h of resuscitation following haemorrhagic shock. The tissue was then dehydrated and embedded in wax. The wax block was cut into sections that were 3 μm thick. Afterward, the tissue was placed on a glass slide, which was then heated at 60 °C. After dehydration, paraffin sections were sealed, dewaxed in water, and stained with haematoxylin and eosin. The pathological changes in the colons of the rats in both groups were analysed.
Detection of heme Oxygenase-1 (HO-1) and Nuclear factor erythroid 2-related factor 2 (Nrf2) by immunohistochemistry
The immunohistochemistry procedure involved fixing fresh colon tissue for over 24 h with a 4% paraformaldehyde fixative solution, dehydrating the tissue, embedding it in paraffin, cutting it into 3 μm thick sections, and immersing the sections in xylene for 30 min and anhydrous ethanol for 15 min. To facilitate antigen healing, the tissue slices were then cleaned. Subsequently, the sections were incubated for 25 min at room temperature in a 3.0% hydrogen peroxide solution. Lastly, the sections were incubated overnight after the primary antibodies (HO-1 and Nrf2) were added dropwise. Dropwise additions of secondary antibodies matching the species of the primary antibody were made, and the mixture was incubated for 50 min at room temperature. The pieces were then allowed to dry before being mixed with a freshly prepared diaminobenzidine (DAB) colour development solution. The samples were then sealed, dried, and prepared for analysis.
Detection of reactive oxygen species (ROS) by frozen section staining assay method
The four basic phases in the frozen section staining assay method are staining, nucleation, sealing, and microscopic imaging. These processes are necessary to determine the concentration of ROS. The samples were incubated in the dark for 30 min at 37.0 °C. Afterward, the slides were submerged in Phosphate-Buffered Saline (PBS), shaken, and shielded from light for three separate 5-min wash cycles on a decolorisation shaker. Once the slices have dried, stain the nuclei by adding drops of 4′,6-diamidino-2-phenylindole (DAPI) staining solution. After that, seal the slides by submerging them in PBS and rinsing them three times on a shaker to remove discolouration. The tablets were sealed with an anti-fluorescence quenching mounting solution after the slices had been partially spin-dried. Using an inverted fluorescent microscope from Nikon, the sections were examined, and pictures were taken.
Detection of myeloperoxidase (MPO) by immunofluorescence method
The immunofluorescence method involves seven steps for detecting MPO content: dewaxing the paraffin section, antigen retrieval, application of primary and secondary antibodies, nuclear sealing with DAPI counterstain, and microscopic inspection.
Statistical analysis
The Grandpad 8.0.2 software was used to analyse the data. Before experiments were initiated, the required number of animals for each experiment was determined based on α = 0.05 and a power of 0.9 to detect differences in primary end points (MAP, Glu, and THbc) greater than 10%. The continuous variables data, including HR, MAP, PaCO2, PaO2, pH, and other indicators, were analyse by Two-way ANOVA to compare the different groups. The 72-h survival rate of rats in the three groups was analysed as categorical variables using the Log-rank test. p < 0.05 indicates a statistically significant difference.
Results
Comparison of 72h survival rates of rats in 3 groups
illustrates the statistical significance (p < 0.05) of the 72-h survival rates of rats in the polyHb and polyCOHb groups, which were 50.00% (6/12) and 58.33% (7/12), respectively, compared to 8.33% (1/12) in the HES group.
PolyCOHb can be maintained the MAP
The MAP and HR at Baseline, SH, RS 0h, and RS 1h did not change significantly. As shown in , the MAP of rats in the polyHb group was considerably higher at RS 0h compared to the polyCOHb group, and the difference was statistically significant (p < 0.05).
PolyCOHb has an oxygen supply function similar to that of polyHb
and demonstrate that there were no appreciable variations in PaO2, PaCO2, Glu, THb, Lac, BEecf, and pH at Baseline, SH, RS 0h, and RS 1h(p > 0.05).
PolyCOHb can increase CO content of the plasm
The polyCOHb group’s HbO2(%) at RS 0h was substantially lower than the polyHb group’s (p < 0.05). Furthermore, as illustrates, at RS 0h and RS 1h, the polyCOHb group’s rats had higher HbCO content and HbCO (%) than the polyHb group’s rats, and these differences were statistically significant (p < 0.05).
PolyCOHb can reduce inflammatory factors in plasma and colon tissue
illustrates that rats in the polyCOHb group had lower plasma MDA at RS 1h compared to the rats in the polyHb group, and the polyCOHb group had more SOD in both the plasma and colon tissue (p < 0.05). And there were no statistically significant differences in the levels of IL-6 and TNF-α in colon tissue between the polyCOHb group and the polyHb group (p > 0.05).
PolyCOHb can reduce inflammation in colon tissue
illustrates that rats in the polyCOHb group showed decreased colonic tissue cell contents at RS 1h, along with edoema in the lamina propria and submucosa, vasodilation, proliferation of inflammatory cells in the intestinal lumen, shedding of necrotic epithelial cells, neutrophil infiltration, necrosis of glandular epithelial cells, rupture of goblet cells, and mucus exudation, in comparison to the rats in the polyHb group.
Figure 8. Representative figures of rat colonic pathological slides with HE stained in two groups. (A–D) Represent pathological sections of colonic tissue of polyHb, while (E–H) represent pathological sections of colonic tissue of polyCOHb. (A,B,E,F) Are at 10X magnification, while (C,D,G,H) are at 40X magnification. The green arrows indicate cell contents, the yellow arrows indicate edoema of the lamina propria and submucosa, the blue arrows indicate vasodilation, the red arrows indicate inflammatory cell growth in the intestinal lumen, the dark blue arrow indicates epithelial cell necrosis shedding, the black arrow indicates neutrophil infiltration, the orange arrow indicates glandular epithelial cell necrosis, and the grey arrow indicates goblet cell rupture and mucus exudation.
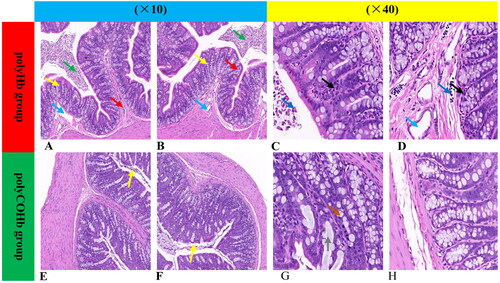
PolyCOHb can activate the Nrf2-HO-1-ROS pathway in colon tissue
Rats in the polyCOHb group showed higher levels of HO-1 and Nrf2 in their colonic tissue at RS 1h than did the rats in the polyHb group. For further information, see and which demonstrate that the rats in the polyCOHb group had colonic tissue with fewer ROS than the rats in the polyHb group, this difference was statistically significant (p < 0.05).
Figure 9. Nrf2 And HO-1 of paraffin sections immunohistochemistry, the (A–D) diagrams belong to the Nrf2 of rat colon tissue chart, (E–H) belong to the HO-1 of rat colon tissue, and the (I,J) charts belong to the comparison of the IOD values of the two groups of rat colon tissue (n = 3).
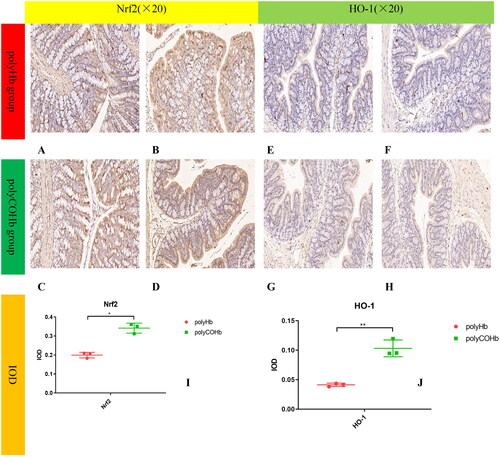
Figure 10. Comparison of ROS of the rat colon tissue in two groups. (A,B) Show staining plots of ROS frozen sections in polyHb rats, (C,D) of ROS + DAPI in polyHb rats, (E,F) of ROS in polyCOHb rats, (G,H) of ROS + DAPI in polyCOHb rats, and for comparison of IOD values of frozen sections of colonic tissue in the two groups (n = 3).
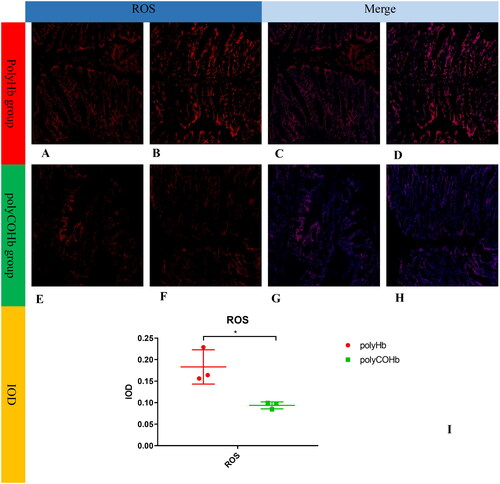
PolyCOHb can reduce the content of MPO in colon tissue
provides further information about that at RS 1h, the rats in the polyCOHb group had a lower MPO content in their colonic tissue than the rats in the polyHb group, and this difference was statistically significant (p < 0.05).
Figure 11. Immunofluorescence map of MPO paraffin sections of rat colon tissue. (A) Showed the immunofluorescence map of MPO in rat colon tissue in the polyHb group(×200). (B) Displays the DAPI image of rat colon tissue in the polyHb group(×200). (C) Presents the combined image of MPO and DAPI in rat colon tissue in the polyHb group(×200). (D) Shows the individual immunofluorescence map of MPO in rat colon tissue in the polyHb group(×400). (E) Displays the DAPI image of rat colon tissue in the polyHb group(×400). (F) Shows the combined image of colon tissue MPO and DAPI in the polyHb group(×400). (G) Presents the immunofluorescence map of MPO alone in rat colon tissue. Colon tissue in the polyCOHb group(×200). (H) Shows the DAPI picture of rat colon tissue in the polyCOHb group (×200). shows the picture of the coincidence of MPO and DAPI in the polyCOHb group(×200). (J) Shows the MPO colon tissue of the polyCOHb group(×400). (K) Shows the DAPI picture of the rat colon tissue in the polyCOHb group(×400). (L) Shows the coincidence of the colon tissue MPO and DAPI of the polyCOHb group (×400). The M plot compares the IOD values of MPO in the colon tissue of two groups of rats(n = 3).
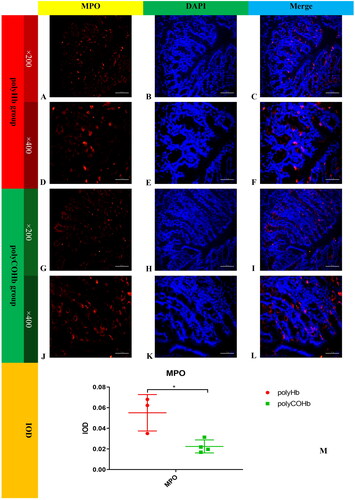
Figure 12. Diagram of the mechanism of CO relieving inflammation in colonic tissue in rats with haemorrhagic shock. This figure was drawn by the authors. CO: carbon monoxide, MDA: malondialdehyde, Nrf2: nuclear factor erythroid 2-related factor 2, HO-1: haem oxygenase-1, ROS: reactive oxygen species, MPO myeloperoxidase, SOD: superoxide dismutase, NO: Nitric oxide, eNOS: endothelial nitric oxide synthase, OONO-: peroxynitrite.
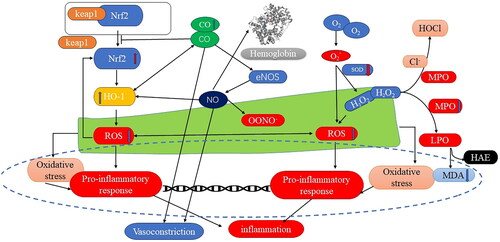
Diagram illustrating the mechanism of polyCOHb in reducing inflammation in colonic tissue in rats with haemorrhagic shock
A diagram showing the mechanism of CO alleviating inflammation in colonic tissue in rats with haemorrhagic shock was produced based on the differences in the treatment of polyCOHb and polyHb. The figure demonstrated decreased levels of ROS and MPO, increased levels of Nrf2, HO-1, and SOD content, and decreased pathological verification in colonic tissue. It also indicated elevated levels of CO and MDA in plasma. Since polyCOHb is a carbon monoxide-releasing molecule, it can effectively raise Nrf2 levels, which raise HO-1 levels. The efficient reduction of ROS levels brought about by HO-1 overexpression decreases pro-inflammatory response frequency, which in turn reduces inflammation. Additionally, CO can directly impact vascular smooth muscle contraction and enhance nitric oxide synthase’s ability to produce NO. The MAP drops as a result of this enzyme’s action on vascular smooth muscle. Moreover, CO raises the SOD concentration, which lowers the ROS content. It also raises the content of MDA while decreasing MPO levels via the H2O2 pathway. Highly oxidising chemicals that can trigger inflammatory responses in the body include ROS, OONO-, and H2O2.
Discussion
HBOCs, as derivatives of haemoglobin, are easily oxidised to MetHb in the presence of oxygen. This oxidation not only results in the loss of their crucial oxygen-carrying function but also poses toxicity to the body [Citation6,Citation20]. According to research [Citation13], CO can bind to polyethylene glycolated haemoglobin and increase its stability, which is beneficial for its preservation. When CO enters the body quickly and in significant doses, poisoning may result. Contrary to popular belief, CO is a significant endogenous signalling molecule as well, and the data indicate that CO has a high safety thresh [Citation21,Citation22]. Another opinion suggests that haemoglobin and CO have a higher binding affinity than oxygen. PolyCOHb loses its ability to deliver oxygen when CO binds to haemoglobin, as it can no longer effectively bind oxygen. According to our research, polyCOHb can continue to release CO and bind O2 when there is sufficient oxygen in the lungs. PolyCOHb was used to observe the success of resuscitation in rats experiencing 50% haemorrhagic shock to test this theory. This observation laid the scientific foundation for subsequent research.
In this study, a 50% rat model of haemorrhagic shock was established to investigate the therapeutic effects of polyCOHb on haemorrhagic shock. In haemorrhagic shocked rats, the results indicated a difference between polyCOHb and polyHb in terms of improving MAP after resuscitation. The recovery MAP was closer to the baseline, while the recovery MAP was slightly higher. The body can, however, absorb a certain quantity of haemoglobin tetramer, which then quickly binds to nitric oxide (NO), a vasodilating component present in blood and vascular endothelial cells after infusing the polyCOHb and polyHb. This binding causes vasoconstriction and raises MAP [Citation21]. CO, as one of the endogenous signalling molecules, has a vasodilating effect similar to NO [Citation23], and study [Citation19] have pointed out that CO can also stimulate the production of NO synthase, increase NO levels, and subsequently dilate blood vessels, leading to a decrease in MAP close to the baseline.
The tension created by physically dissolved oxygen molecules in arterial blood is known as PaO2, and it is a crucial metric for determining whether an organism is oxygen-poor or oxygen-deficient. The blood’s physically dissolved oxygen can be transported by polyHb to the hypoxic tissues and organs, reducing PaO2 levels. Nevertheless, following resuscitation, there is no statistically significant difference in the PaO2 of rats between polyHb and polyCOHb. This implies that polyCOHb can maintain polyHb’s ability to carry oxygen, release CO quickly after entering the body, and transport O2 to the tissues and organs that require oxygen. Our theory that polyCOHb enters the body and releases CO is supported by this data.
After 0 and 1 h of resuscitation, the rats in the polyHb and polyCOHb groups had significantly lower levels of Lac and Beecf than HS. The difference in Lac content between the polyHb and polyCOHb groups was not statistically significant. These findings suggest that polyCOHb and polyHb play similar roles in oxygen supply, facilitating the transport of oxygen to hypoxic tissues and organs, promoting energy metabolism, reducing the proportion of anaerobic respiration, and decreasing lactic acid levels.
Blood oxygen analysis can visually reflect the distribution of various types of haemoglobin (HbO2, HbCO, Hb) in the body. The results showed that after 0 h and 1 h of polyCOHb resuscitation, the body’s HbCO content and HbCO (%) were significantly higher than that in the polyHb. Additionally, the HbCO content at RS 1h was lower than at RS 0h. This provides direct evidence that polyCOHb can release CO into the blood and that polyCOHb can combine with O2 again after releasing CO, thereby completing the function of oxygen transport in the body. Additionally, rats with 50% acute haemorrhagic shock showed a similar 72-h survival rate.
The levels of HO-1 and Nrf2 in the colons of rats treated with polyCOHb for haemorrhagic shock were also investigated in this study. The findings showed that polyCOHb significantly increased the levels of CO in rats suffering from haemorrhagic shock compared to polyHb. Additionally, it increased the levels of HO-1 and Nrf2 through carbon monoxide (CO). Consequently, there was a reduction in ROS levels, which are mainly responsible for the inflammation in the colons of rats. After reperfusion, several pathways generate reactive oxygen species (ROS) in rats experiencing acute severe haemorrhagic shock. First, the ROS produced due to blood loss during the haemorrhagic shock phase. Secondly, the body produces ROS when haemoglobin-based oxygen carriers (HBOCs) are being reperfused. During the body’s reperfusion, there is an abundance of oxygen, leading to increased levels of reactive oxygen species. An inflammatory response in the organism can be triggered by several pathways of ROS formation during shock resuscitation in rats suffering from haemorrhagic shock. Nevertheless, extra O2 can replace the CO in polyCOHb once it has been infused into the body. Next, the free CO functions within the body as a signalling molecule. One approach could involve reducing the ROS concentration through the Nrf2-HO-1 pathway, leading to a decrease in the inflammatory response of the rat organism. Influencing Nitric Oxide Synthase (NOS) through CO may be another pathway [Citation24,Citation25], which affects the synthesis of NO and increases the content of NO. This ultimately results in vasodilation, which reduces the organism’s blood pressure. Furthermore, CO directly influences vasodilation, which further lowers blood pressure. MAP decreases during the resuscitation of rats suffering from haemorrhagic shock due to the combined action of these two processes.
HO-1 is the rate-limiting enzyme that degrades haem into biliverdin, iron, and CO [Citation26]. Studies [Citation26,Citation27] have indicated that HO-1 can elevate the CO levels in the kidneys and provide protection against inflammatory cytotoxic responses. Remarkably, it has been demonstrated that CO administered in gaseous [Citation28] or CORM [Citation29] form increases HO-1 expression in a dose-dependent way. In the process of resuscitating rats suffering from haemorrhagic shock, polyCOHb, a carbon monoxide-releasing molecule (CORM), can effectively increase the concentration of HO-1 in the rat colon. This result lends more credence to the association between CO and HO-1 in haemorrhagic shock and is consistent with the previously stated conclusions.
In this study, a rat model of acute 50% haemorrhagic shock was used. The process of ischaemia and reperfusion results in damage to the body. Succinate accumulated during ischaemia promotes complex I of the electron transport chain, significantly increasing the production of ROS. This leads to protein carbonylation and lipid peroxidation [Citation30], ultimately promoting apoptosis and necrosis. ROS is an important cause of inflammation in the body, and some studies [Citation28,Citation29] have pointed out that CO can effectively reduce ROS through HO-1.
CO predominantly interacts to A2 receptors through CD39 to protect against renal ischaemia-reperfusion damage. Adenosine triphosphate (ATP) synthesis is stimulated by this interaction, and A2 receptors are connected to G-proteins, which modify HIF-α expression and increase the synthesis of EPO. When it comes to the kidneys’ ischaemia-reperfusion injury, EPO is crucial. In contrast to the previously described mechanism, in which CO guards against visceral ischaemia-reperfusion injury, HO-1 and SOD are essential components of the mechanism via which CO shields the colon in rats suffering from haemorrhagic shock.
This work performed an initial investigation into the management of acute 50% haemorrhagic shock with polyCOHb. The findings showed that polyCOHb can release CO quickly during resuscitation in rats that are hypoxic and ischaemia. In addition, it can bind to O2 during pulmonary circulation quickly, displaying the same oxygen-carrying function as polyHb.
Rats in haemorrhagic shock who were given HES resuscitation had a much lower 72-h survival rate than the two experimental groups. The ability of polyHb and polyCOHb to sufficiently oxygenate the organism’s hypoxic tissues increases the organism’s overall survival rate, which could be one explanation for this. Furthermore, it has been noted that HES may result in haemorrhagic complications [Citation31], which raises the mortality rate when using HES to resuscitate haemorrhagic shock. Although thorough research has not been done, the addition of polyHb and polyCOHb may have improved bleeding problems. It is highly speculative to address the possible release of CO and the following molecular signalling by CO or the oxygen transporting activity by the polyCOHb. The results of more animal tests are required to support the final conclusions, as the current study used a small number of animals and only produced preliminary findings, which are the study’s faults and limitations.
To summarise, polyCOHb and polyHb showed comparable effectiveness in rat models of anti-haemorrhagic shock. They were able to maintain MAP, correct acidosis, and successfully reduce the inflammatory response in the colon of rats suffering from haemorrhagic shock by the use of CO.
Authors’ contributions
Shasha Hao and Huan Wang: Conceptualisation, Implementation research and conduction the analysis data, Writing-original draft. Shen Li: Implementation research, Conduction research and Sample preparation. Honghui Zhang: Conduction research and Sample preparation. Xintong Xie: Conduction research, Sample detection, Data curation. Jiaxin Liu: Conceptualisation and Supervision. Chengmin Yang: Conceptualisation and Supervision. Wentao Zhou and Hong Wang: Conceptualisation and Supervision, Interpretation of the data.
Ethical statement
All experimental research on animals were carried out in accordance with the ARRIVE guidelines (https://arriveguidelines.org/).
Disclosure statement
No potential conflict of interest was reported by the author(s).
Data availability statement
The data supporting the findings of this study are available within the article and its Supplementary Materials.
Additional information
Funding
References
- Kuo K, Palmer L. Pathophysiology of hemorrhagic shock. J Vet Emerg Crit Care (San Antonio). 2022;32(S1):22–31. doi: 10.1111/vec.13126.
- Sperry JL, Guyette FX, Brown JB, et al. Prehospital plasma during air medical transport in trauma patients at risk for hemorrhagic shock. N Engl J Med. 2018;379(4):315–326. doi: 10.1056/NEJMoa1802345.
- Nugent WH, Jubin R, Buontempo PJ, et al. Microvascular and systemic responses to novel PEGylated carboxyhaemoglobin-based oxygen carrier in a rat model of vaso-occlusive crisis. Artif Cells Nanomed Biotechnol. 2019;47(1):95–103. doi: 10.1080/21691401.2018.1543197.
- Yu X, Wang Z, Shen Y, et al. Population-based projections of blood supply and demand, China, 2017-2036. Bull World Health Organ. 2020;98(1):10–18. doi: 10.2471/BLT.19.233361.
- Wang Z, Chen T, Li X, et al. Oxygen-releasing biomaterials for regenerative medicine. J Mater Chem B. 2023;11(31):7300–7320. doi: 10.1039/d3tb00670k.
- Sen Gupta A. Hemoglobin-based Oxygen Carriers: current State-of-the-art and Novel Molecules. Shock. 2019;52(1S Suppl 1):70–83. doi: 10.1097/SHK.0000000000001009.
- D’agnillo F, Chang TM. Cross-linked hemoglobin-superoxide dismutase-catalase scavenges oxygen-derived free radicals and prevents methemoglobin formation and iron release. Biomater Artif Cells Immobilization Biotechnol. 1993;21(5):609–621. doi: 10.3109/10731199309117385.
- Chen G, Duan Y, Liu J, et al. Antioxidant effects of vitamin C on hemoglobin-based oxygen carriers derived from human cord blood. Artif Cells Nanomed Biotechnol. 2016;44(1):56–61. doi: 10.3109/21691401.2015.1111239.
- Cooper CE, Silaghi-Dumitrescu R, Rukengwa M, et al. Peroxidase activity of hemoglobin towards ascorbate and urate: a synergistic protective strategy against toxicity of Hemoglobin-Based Oxygen Carriers (HBOC). Biochim Biophys Acta. 2008;1784(10):1415–1420. doi: 10.1016/j.bbapap.2008.03.019.
- Chang EJ, Lee TH, Mun KC, et al. Effects of polyhemoglobin-antioxidant enzyme complex on ischemia-reperfusion in kidney. Transplant Proc. 2004;36(7):1952–1954. doi: 10.1016/j.transproceed.2004.08.146.
- Martins PN, Reutzel-Selke A, Jurisch A, et al. Induction of carbon monoxide in donor animals prior to organ procurement reduces graft immunogenicity and inhibits chronic allograft dysfunction. Transplantation. 2006;82(7):938–944. doi: 10.1097/01.tp.0000232716.91887.c5.
- Yang X, Lu W, Hopper CP, et al. Nature’s marvels endowed in gaseous molecules I: carbon monoxide and its physiological and therapeutic roles. Acta Pharm Sin B. 2021;11(6):1434–1445. doi: 10.1016/j.apsb.2020.10.010.
- Nagao S, Taguchi K, Sakai H, et al. Carbon monoxide-bound hemoglobin vesicles ameliorate multiorgan injuries induced by severe acute pancreatitis in mice by their anti-inflammatory and antioxidant properties. Int J Nanomedicine. 2016;11:5611–5620. doi: 10.2147/IJN.S118185.
- Kwong AM, Luke PPW, Bhattacharjee RN. Carbon monoxide mechanism of protection against renal ischemia and reperfusion injury. Biochem Pharmacol. 2022;202:115156. doi: 10.1016/j.bcp.2022.115156.
- Nugent WH, Sheppard FR, Dubick MA, et al. Microvascular and Systemic Impact of Resuscitation with PEGylated Carboxyhemoglobin-Based Oxygen Carrier or Hetastarch in a Rat Model of Transient Hemorrhagic Shock. Shock. 2020;53(4):493–502. doi: 10.1097/SHK.0000000000001370.
- Zhou W, Li S, Hao S, et al. Protective effect and mechanism of low P50 haemoglobin oxygen carrier on isolated rat heart. Artif Cells Nanomed Biotechnol. 2022;50(1):121–129. doi: 10.1080/21691401.2021.2017947.
- Li Y, Yan D, Hao S, et al. Polymerized human placenta hemoglobin improves resuscitative efficacy of hydroxyethyl starch in a rat model of hemorrhagic shock. Artif Cells Nanomed Biotechnol. 2015;43(3):174–179. doi: 10.3109/21691401.2015.1024846.
- Zhou W, Zhao L, Wang J, et al. An optimal polymerization conditions for poly-human placenta hemoglobin with lower mean molecular weight. Artif Cells Nanomed Biotechnol. 2013;41(5):289–292. doi: 10.3109/21691401.2012.744995.
- National Research Council (US) Committee for the Update of the Guide for the Care and Use of Laboratory Animals.Guide for the Care and Use of Laboratory Animals. Washington (DC); 8th ed. Washington (DC): National Academies Press (US);2011. PMID: 21595115.
- Alayash AI. Blood substitutes: why haven’t we been more successful?. Trends Biotechnol. 2014;32(4):177–185. doi: 10.1016/j.tibtech.2014.02.006.
- Yang XX, Ke BW, Lu W, et al. CO as a therapeutic agent: discovery and delivery forms. Chin J Nat Med. 2020;18(4):284–295. doi: 10.1016/S1875-5364(20)30036-4.
- Yang X, DE Caestecker M, Otterbein LE, et al. Carbon monoxide: an emerging therapy for acute kidney injury. Med Res Rev. 2020;40(4):1147–1177. doi: 10.1002/med.21650.
- Choi YK, Kim YM. Regulation of endothelial and vascular functions by carbon monoxide via crosstalk with nitric oxide. Front Cardiovasc Med. 2021;8:649630. doi: 10.3389/fcvm.2021.649630.
- Kaczara P, Proniewski B, Lovejoy C, et al. CORM-401 induces calcium signalling, NO increase and activation of pentose phosphate pathway in endothelial cells. Febs J. 2018;285(7):1346–1358. doi: 10.1111/febs.14411.
- Yang PM, Huang YT, Zhang YQ, et al. Carbon monoxide releasing molecule induces endothelial nitric oxide synthase activation through a calcium and phosphatidylinositol 3-kinase/Akt mechanism. Vascul Pharmacol. 2016;87:209–218. doi: 10.1016/j.vph.2016.09.010.
- Maines MD. The heme oxygenase system: a regulator of second messenger gases. Annu Rev Pharmacol Toxicol. 1997;37:517–554. doi: 10.1146/annurev.pharmtox.37.1.517.
- Hull TD, Kamal AI, Boddu R, et al. Heme oxygenase-1 regulates myeloid cell trafficking in AKI. J Am Soc Nephrol. 2015;26(9):2139–2151. doi: 10.1681/ASN.2014080770.
- Correa-Costa M, Gallo D, Csizmadia E, et al. Carbon monoxide protects the kidney through the central circadian clock and CD39. Proc Natl Acad Sci U S A. 2018;115(10):E2302–E2310. doi: 10.1073/pnas.1716747115.
- Lin CC, Hsiao LD, Cho RL, et al. Carbon monoxide releasing molecule-2-upregulated ROS-dependent heme oxygenase-1 axis suppresses lipopolysaccharide-induced airway inflammation. Int J Mol Sci. 2019;20(13):3157-3179. doi: 10.3390/ijms20133157.
- Martin JL, Gruszczyk AV, Beach TE, et al. Mitochondrial mechanisms and therapeutics in ischaemia reperfusion injury. Pediatr Nephrol. 2019;34(7):1167–1174. doi: 10.1007/s00467-018-3984-5.
- Haase N, Wetterslev J, Winkel P, et al. Bleeding and risk of death with hydroxyethyl starch in severe sepsis: post hoc analyses of a randomized clinical trial. Intensive Care Med. 2013;39(12):2126–2134. doi: 10.1007/s00134-013-3111-9.