ABSTRACT
Pressureless sintered B4C and B4C-SiC ceramics were subjected to tests of dry sliding against SiC balls at 5 N, 10 N and 20 N. The effects of load on the respective tribological properties of B4C and B4C-SiC ceramics were studied. Meanwhile, the tribological properties and friction, wear mechanisms of B4C and B4C-SiC ceramics at different loads were compared. Both the friction coefficient and wear rate of B4C ceramics first decreased and then increased with increases in load, while both the friction coefficient and wear rate of B4C-SiC ceramics increased with increases in load. At low load, the B4C-SiC ceramics showeda lower friction coefficient and wear rate. At intermediate load, the B4C ceramics showed a lower friction coefficient, and no difference between the wear rates of B4C and B4C-SiC ceramics was apparent. At high load, the B4C ceramics showed a lower friction coefficient, while the B4C-SiC ceramics showed a lower wear rate. With increases in load from 5 N to 20 N for the B4C ceramics, the wear mechanism changed from adhesive wear to delamination and spalling of tribofilm; for the B4C-SiC ceramics, meanwhile, the wear mechanism changed from surface polishing and mild abrasion to micro-fracture.
1. Introduction
B4C and B4C-based ceramics possess such superior performance characteristics as exceptional hardness, high elastic modulus, low density, good wear resistance and good chemical inertness at room temperature [Citation1,Citation2]. B4C and B4C-based ceramics are considered to be potential candidates for tribological applications, such as mechanical seals, bearings, cutting tools, wheel dressing tools and blast nozzles [Citation3–Citation7]. Li et al. [Citation8,Citation9] studied the tribological properties of B4C-hBN composite ceramics, and obtained a decreased coefficient of friction but increased specific wear rate system as compared to monolithic B4C ceramics. Alexander et al. [Citation10] investigated a B4C-carbon nanotube system and obtained a specific wear rate of 1.06 × 10−6 mm3/N·m, which was lower than that of B4C ceramics. Murthy et al. [Citation11] added ZrO2 to B4C ceramics and noted that ZrB2 formed in situ and that sub-micron-sized round pores formed owing to the formation of CO gas could help to deflect and/or arrest cracks, improving the tribological properties of B4C ceramics. Among B4C-based composite ceramics, it has been observed that B4C-SiC composite ceramics showed better mechanical properties and oxidation resistance as compared to monolithic B4C ceramics [Citation12–Citation14]. However, little literature is available on the friction and wear performance of B4C-SiC ceramics [Citation15,Citation16].
It was reported that the tribological behaviors of ceramic materials under dry sliding conditions are complex and dependent on such external factors as humidity, temperature, sliding speed, load, counterpart, and atmosphere [Citation17]. Sharma et al. [Citation18] mentioned that the friction coefficient of SiC ceramics first increased and then decreased and that the specific wear rate decreased with increases in load. Sonber et al. [Citation19] found that the coefficient of friction of B4C ceramics decreased and the specific wear rate increased with increases in load. Micele et al. [Citation20] pointed out that the specific wear rate of SiC ceramics increased as the sliding speed increased. Gogotsi et al. [Citation21] reported that the coefficient of friction of B4C ceramics decreased with increases in sliding speed. Murthy et al. [Citation22] and Cao et al. [Citation23] found that both the friction coefficients and specific wear rates of SiC ceramics and B4C ceramics decreased with increases in humidity, respectively. To date, only a few reports are available on the friction and wear properties of B4C ceramics and no reports on those of B4C-SiC ceramics at different loads when other test conditions are the same. Furthermore, in order to explore the potential of B4C and B4C-SiC ceramics for applications in the automotive and aerospace industries as tribological structural components such as abrasion resistance linings, exhaust systems for liquid rocket engine turbopumps, and aerospace turbine blades, it is important to evaluate the tribological behaviors of these ceramics at different loads. Therefore, we decided to take a fresh look at the effects of load on the tribological properties of B4C and B4C-SiC composite ceramics when slid against SiC balls under dry sliding conditions. The tribological properties of B4C ceramics and B4C-SiC ceramics at different loads were also compared, moreover, and their friction and wear mechanisms at different loads were analyzed.
2. Experimental procedures
Commercial B4C powder (Grade HS, H. C. Starck, Germany) and SiC powder (Grade OY-15, Yakushima Denko, Japan) were used as raw materials. The typical characteristics of the B4C powder and SiC powder based on the manufacturers’ data are shown in . Monolithic B4C ceramics and B4C-40 wt% SiC composite ceramics were prepared using a pressureless sintering technique, and carbon black powder (average particle size: 20–30 nm) was used as a sintering additive for the two ceramics. First, each powder mixture was ball-milled for 24 h using SiC balls and ethanol as the milling media. After drying, the powder mixture was pressed into disks, which were then cold-isostatically pressed at 196 MPa. The disks were sintered at 2300°C in an argon atmosphere, and their dwell time was 16 h.
Table 1. Typical characteristics of B4C powder and SiC powder.
Density was determined using Archimedes’ method and the relative density evaluated. Hardness was measured for the polished surfaces by Vickers indentation with a load of 1 kg for a dwell time of 10 s. The lengths of cracks generated by Vickers indentation were measured and the fracture toughness (KIC) was estimated using the formula KIC = 0.016(E/H)0.5Pc−1.5 [Citation24]. Each measurement was repeated five times, and the data was averaged. The physical and mechanical performances of sintered B4C ceramics and B4C-SiC composite ceramics are shown in . The physical and mechanical performances of commercially available SiC balls based on the manufacturers’ data are also shown in .
Table 2. Physical and mechanical performances of sintered B4C ceramics, B4C-SiC composite ceramics and commercially available SiC balls.
Dry sliding tests were performed in a ball-on-disc tribometer (T-18-0162, NANOVEA, US). The size of the disk samples was 29 × 26 × 4.5 mm3. The surfaces of the disks were ground and polished to Ra = 15–35 nm. Commercially available SiC balls with 9.5 mm diameter were used as counterparts. Before the wear test, both the disk samples and balls were ultrasonically cleaned in acetone. The balls were kept stationary under the applied load to make a track radius of 7 mm, while the disks were rotated at a speed of 0.1 m/s for a sliding distance of 100 m. The tribological tests were carried out at 21 ± 3°C and 45 ± 5 RH in ambient air at three different loads (5 N, 10 N, 20 N).
During the tests, the friction coefficients were automatically calculated and recorded by a microprocessor-controlled data acquisition system. Wear volume losses (ΔV, mm3) for the disks and balls were evaluated by the following means: for the disks, four cross-sectional areas on the wear tracks, each at 90º with respect to the previous one, were measured using a surface profilometer and the four areas were averaged and multiplied by the wear track length to obtain the ΔV. For the balls, the radius at the wear scars was measured using a microscope and the ΔV was then calculated according to this radius at the wear scars and the original radius of the ball. The specific wear rates (WR, mm3/N·m) of the disks and balls were then calculated according to the following equation:
WR = ΔV/(Ps)
where P is the normal load and s is the sliding distance. Three repeated tests were performed for each tribopair. The worn surfaces of the B4C and B4C-SiC ceramics were observed and analyzed by SEM and EDS. The wear scars on the SiC balls were observed by microscope.
3. Results and discussion
3.1. Effects of load on the coefficients of friction of the B4C and B4C-SiC ceramics
The coefficients of friction of the B4C and B4C-SiC ceramics sliding against SiC balls with a sliding distance at different loads and average coefficients of friction of the B4C and B4C-SiC ceramics in a steady-state period at different loads are presented in . An initial run-in period followed by a steady-state period is observed for all the B4C and B4C-SiC ceramics sliding against SiC balls at different loads. For the B4C ceramics, the average coefficient of friction varied in the range of 0.24–0.44 with increases in load from 5 N to 20 N, and larger fluctuations in friction occurred at low load (5 N). The coefficient of friction of the B4C ceramics first decreased and then increased with increases in load, but the coefficient of friction at high load (20 N) was still lower than that at low load. For the B4C-SiC ceramics, the average coefficient of friction varied in the range of 0.32–0.48 as the load increased from 5 N to 20 N, and larger fluctuations in friction occurred at low load and high load. The coefficient of friction of the B4C-SiC ceramics increased with increases in load. At low load, the B4C-SiC ceramics showed a lower coefficient of friction. At intermediate load (10 N) and high load, the B4C ceramics exhibited lower coefficients of friction.
3.2. Effects of load on specific wear rates of the B4C and B4C-SiC ceramics
The average specific wear rates of the B4C and B4C-SiC ceramics sliding against SiC balls at different loads are shown in ). For the B4C ceramics, the specific wear rate varied in the range of 11.0–22.4 × 10−6 mm3/N·m as the load was increased from 5 N to 20 N. The specific wear rate of the B4C ceramics first decreased and then increased with increases in load, and the specific wear rate of the B4C ceramics reached its minimum value at a load of 10 N. For the B4C-SiC ceramics, the specific wear rate varied in the range of 1.3–7.3 × 10−6 mm3/N·m as the load was increased from 5 N to 20 N. The specific wear rate of the B4C-SiC ceramics increased with increases in load. The B4C-SiC ceramics showed a lower specific wear rate at every load, but no difference between the specific wear rates of B4C ceramics and B4C-SiC ceramics was apparent at intermediate load.
The average specific wear rates of SiC balls sliding against B4C and B4C-SiC ceramics at different loads are presented in ). For SiC balls sliding against B4C ceramics, the specific wear rate varied in the range of 1.2–3.5 × 10−6 mm3/N·m as the load was increased from 5 N to 20 N. The specific wear rate of the SiC balls first decreased and then increased with increases in load, and the specific wear rate of the SiC balls reached its minimum value at 10 N. For SiC balls sliding against B4C-SiC ceramics, the specific wear rate varied in the range of 0.9–3.6 × 10−6 mm3/N·m as the load was increased from 5 N to 20 N. The specific wear rate of SiC balls increased with increases in load. At low load, SiC balls showed a lower specific wear rate when sliding against the B4C-SiC ceramics. At intermediate load, SiC balls showed a lower specific wear rate when sliding against the B4C ceramics. At high load, SiC balls sliding against the B4C ceramics and SiC balls sliding against the B4C-SiC ceramics revealed similar specific wear rates.
The average systematic specific wear rates of B4C/SiC and B4C-SiC/SiC tribopairs at different loads are summarized in ). The value of the systematic average specific wear rate of every tribopair is the sum of the B4C disk’s or B4C-SiC disk’s and the corresponding SiC ball’s average specific wear rate. For the B4C/SiC tribopair, the average systematic specific wear rate first decreased and then increased with increases in load, and the average systematic specific wear rate reached its minimum value at a load of 10 N. For the B4C-SiC/SiC tribopair, the average systematic specific wear rate increased with increases in load. The B4C-SiC/SiC tribopair showed a lower average systematic specific wear rate at every load, but no difference between the systematic specific wear rates of the B4C/SiC tribopair and B4C-SiC/SiC tribopair was apparent at intermediate load.
Figure 2. Average specific wear rates of (a) the B4C and B4C-SiC ceramics sliding against SiC balls at different loads, (b) SiC balls sliding against the B4C and B4C-SiC ceramics at different loads, and (c) average systematic specific wear rates of the B4C/SiC and B4C-SiC/SiC tribopairs at different loads.
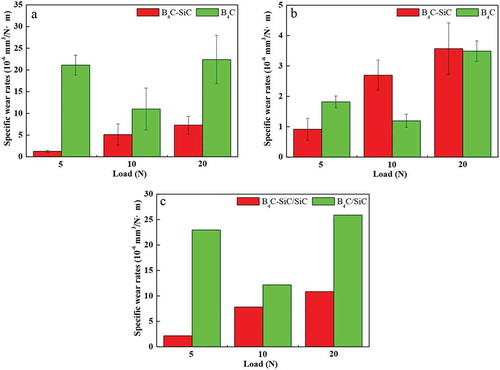
3.3. Friction and wear mechanisms
SEM images of worn surfaces of the B4C and B4C-SiC ceramics at 5 N as well as EDS analyses of the corresponding worn surfaces are presented in . It can be observed that severe adhesive wear was dominant on the B4C ceramics, and the wear surface was quite rough. EDS analysis of the wear surface of the B4C ceramics showed the presence of a small amount of oxygen (2.17 at%), which was induced by a tribochemical reaction. Other scholars [Citation19,Citation25] observed that the formation of B2O3 or H3BO3 induced by a tribochemical reaction could decrease friction on B4C ceramics, but the coefficient of friction for the B4C ceramics was not reduced owing to the presence of a small amount of oxide in this study. One possible reason is that there was only a small amount of oxygen, which resulted in formation of too few oxide layers to reduce the coefficient of friction of the B4C ceramics at 5 N. The other possible reason is that the beneficial influence of the oxide layers was weaker than the deterioration influence of adhesion, resulting in a higher coefficient of friction and specific wear rate for B4C ceramics. In the case of B4C-SiC ceramics, the wear surface was quite smooth. Surface polishing and mild abrasion were dominant in the B4C-SiC ceramics. EDS analysis of the wear surface showed little oxygen (0.43 at%), which was considered to be an impurity from the raw materials. In our previous work [Citation26,Citation27], it was found that a relief structure was formed on the surface of B4C-SiC ceramics owing to differences in hardness between the B4C grains and SiC grains under the same experimental conditions. This relief structure can not only decrease the real contact area between the B4C-SiC ceramics and SiC ball at the sliding interface, reducing the adhesion effect, but can also trap wear pieces formed by mild abrasion, reducing abrasive wear [Citation28]. Therefore, the coefficient of friction and specific wear rate of B4C-SiC ceramics were lower than those of B4C ceramics.
The friction and wear mechanisms of B4C and B4C-SiC ceramics at 5 N can be further understood by observing the wear scars on their counterpart SiC balls (). Extensive micro-fracture and obvious grooves were observed on SiC balls sliding against B4C ceramics, while polishing and slight grooves were observed on SiC balls sliding against B4C-SiC ceramics. Because of a relief structure on the B4C-SiC ceramics, on the one hand, the extent of abrasive wear on the SiC balls sliding against B4C-SiC ceramics was less than that on the SiC balls sliding against B4C ceramics, while, on the other hand, micro-fracture induced by adhesion was obvious on the SiC balls sliding against B4C ceramics, whereas no adhesive wear occurred on the SiC balls sliding against B4C-SiC ceramics. Therefore, due to the severe adhesive wear and abrasive wear on the B4C/SiC tribopair, the coefficient of friction of the B4C/SiC tribopair was higher than that of the B4C-SiC/SiC tribopair, and the specific wear rates of B4C ceramics and their corresponding SiC balls were higher than those of B4C-SiC ceramics and their corresponding SiC balls. Lörcher et al. [Citation29] stated that wear caused by adhesion is greater than that caused by abrasion; thus, the specific wear rate of the B4C ceramics was an order of magnitude higher than that of the B4C-SiC ceramics.
Figure 3. SEM images of worn surfaces of B4C (a) and B4C-SiC (c) ceramics at 5 N. EDS analyses of Figure 3 (a): (b) and Figure 3(c): (d).
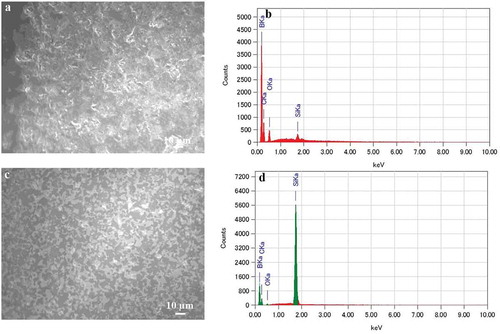
Figure 4. Microscope images of wear scars on SiC balls after sliding against B4C (a) and B4C-SiC (b) ceramics at 5 N.
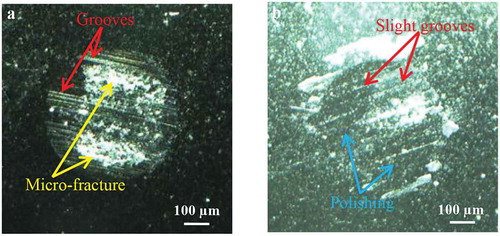
SEM images of the worn surfaces of B4C and B4C-SiC ceramics at 10 N as well as EDS analyses and elemental distributions of the corresponding worn surfaces are presented in . Microscope images of wear scars on SiC balls sliding against B4C and B4C-SiC ceramics at 10 N are shown in . Compared with the worn surface of the B4C ceramics at 5 N, the worn surface of the B4C ceramics at 10 N had become relatively smooth. The EDS analysis of the worn surfaces of B4C ceramics showed the presence of a small amount of oxygen (1.50 at%), which means the number of oxide layers formed did not increase with increases in load. Although other scholars [Citation19,Citation25] found that the number of oxide layers formed on B4C ceramics increased as the load increased, resulting in a gradually decreasing coefficient of friction with increases in load, different results were observed in the present study. The lower coefficient of friction of B4C ceramics at 10 N than that at 5 N was attributed to greater coverage by tribofilm formed on the worn surface. It can be observed from ) that the worn surface of B4C ceramics at 10 N was covered by more tribofilms compared with that in ). It can be seen from ) that obvious grooves were observed on the SiC ball after sliding against B4C ceramics. It can therefore be inferred that there were some hard wear particles at the interface when the B4C ceramics were slid at 5 N. When the load was increased to 10 N, however, those wear particles could be crushed into smaller pieces, which can be aggregated together to form a tribofilm and better compacted on the worn surface due to increased frictional heat. According to the results for elemental distributions (-)), the main composition of tribofilm formed on the worn surface of B4C ceramics was a mixture of B4C, SiC and a small amount of silicon oxide. The phenomenon that increased the extent of coverage by forming a protective tribofilm at the interface with increases in load was also observed by Zhu et al. [Citation30] for B4C coating deposited on a stainless-steel substrate sliding against a WC-Co ball, and the coefficient of friction of the B4C coating was reduced with increases in the extent of coverage by protective tribofilm. Because of the removal of hard wear particles at the sliding interface of the B4C/SiC tribopair, no obvious grooves were observed on the SiC ball sliding against B4C ceramics at 10 N ()), and microfracture was observed only on the SiC ball sliding against B4C ceramics. On the one hand, abrasive wear was reduced owing to the removal of wear particles, and, on the other hand, the formed tribofilm could not only protect the underlying substrate from further wear, but could also separate and prevent direct contact between the B4C ceramics and their SiC counterparts, decreasing adhesive wear. Therefore, the coefficient of friction of the B4C/SiC tribopair at 10 N was lower than that at 5 N, and the specific wear rates of the B4C ceramics and the corresponding SiC balls at 10 N were lower than those at 5 N. The main wear mechanism of B4C ceramics changed from adhesive wear at 5 N to delamination of tribofilm at 10 N.
In the case of B4C-SiC ceramics, it can be observed from ) that grain pullout and micro-fracture were observed. B4C-SiC ceramic is a brittle material, and the fracture of B4C-SiC ceramic would be increased as the load is increased. Increased fracture was also observed for monolithic SiC ceramics at high load by Cho et al. [Citation31]. Therefore, the grain pullout from the matrix not only resulted in a certain degree of damage to the relief structure of B4C-SiC ceramic, but it also roughened the worn surface. On the other hand, tribofilm was also found on the B4C-SiC ceramics. It can be observed from ) that the oxygen content (2.73 at%) was slightly increased compare with ). According to the results for elemental distributions (), the main composition of tribofilm formed on the B4C-SiC ceramics was a mixture of SiC and SiO2, which was different from the main composition of tribofilm on the B4C ceramics. The formation of tribofilm induced by tribochemical reaction was beneficial to improving the tribological properties. Therefore, the friction and wear of B4C-SiC ceramics were controlled by the simultaneous occurrence and competition of mechanical fracture and tribofilm formation. From ), it is known that the specific wear rate of the SiC balls sliding against B4C-SiC ceramic at 10 N was higher than that at 5 N. It is reported that the wear on SiC ceramics induced by fatigue increased as the load was increased, leading to the formation of a number of wear particles [Citation32,Citation33]. Thus, microfracture was observed on the SiC balls sliding against B4C-SiC ceramics at 10 N ()). It can be observed from ) that obvious grooves were found on the SiC balls compared with the case in ). Therefore, it was considered that grain pullout from the B4C-SiC ceramics and the formation of a number of wear particles owing to microfracture of the SiC balls increased abrasive wear. Although some wear particles formed owing to microfracture of SiC balls could be compacted and oxidized to form tribofilm, it was considered that the beneficial influence of this tribofilm was less than the deterioration influence of the microfracture and abrasive wear. Therefore, both the coefficient of friction of the B4C-SiC/SiC tribopair and the specific wear rates of the B4C-SiC ceramics and corresponding SiC balls were increased as the load was increased from 5 N to 10 N. The main wear mechanism of B4C-SiC ceramics changed from surface polishing and mild abrasion at 5 N to surface polishing, mild abrasion and micro-fracture at 10 N.
It can be observed from ) that no obvious grooves were formed on the SiC balls sliding against B4C ceramics compared with the case in ). This means that abrasive wear had little effect on the B4C/SiC tribopair at 10 N. Meanwhile, the formed tribofilm can not only protect the underlying B4C substrate from further wear, but it can also separate and prevent direct contact between the B4C ceramics and their SiC counterparts, thus decreasing adhesive wear. Therefore, the coefficient of friction of the B4C/SiC tribopair was lower than that of the B4C-SiC/SiC tribopair, and no difference between the specific wear rates of B4C ceramics and B4C-SiC ceramics was apparent at 10 N.
Figure 5. SEM images of worn surfaces of the B4C (a) and B4C-SiC (g) ceramics at 10 N. EDS analyses of Figure 5 (a): (b) and Figure 5 (g): (h). Elemental distributions of Figure 5 (a): (c) B; (d) C; (e) Si; (f) O, and of Figure 5 (g): (i) B; (j) C; (k) Si; (l) O.
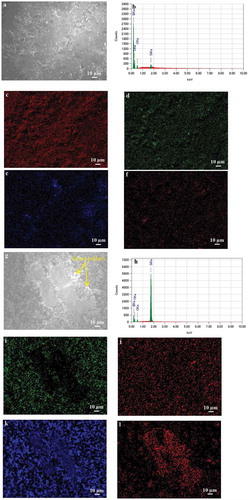
Figure 6. Microscope images of wear scars on SiC balls after sliding against B4C (a) and B4C-SiC (b) ceramics at 10 N.
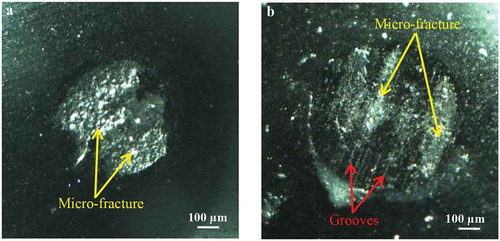
SEM images of the worn surfaces of B4C and B4C-SiC ceramics at 20 N as well as an EDS analysis of the worn surface of the B4C ceramics are presented in . Microscope images of wear scars on SiC balls after sliding against B4C and B4C-SiC ceramics at 20 N are shown in . It can be observed from ) that some spalling pits were formed on the B4C ceramics compared with the worn surface of the B4C ceramics at 10 N ()). Also, the wear on the corresponding SiC ball was further increased, and traces of abrasive wear were still not found on the wear scars of the SiC ball against B4C ceramics. The EDS analysis of worn surface of B4C ceramics showed decreased oxygen content (0.79 at%) at 20 N as compared with at 10 N, which means that increasing the load from 10 N to 20 N still did not promote the tribochemical reaction of B4C ceramics. It is considered, therefore, that the main composition of tribofilm on the B4C ceramics at 20 N was similar to that at 10 N. Because of the occurrence of spalling pits, however, which resulted from exceeding the load capacity of the tribofilm, the worn surface was rougher than that at 10 N, but still smoother than that at 5 N. Therefore, the coefficient of friction of B4C/SiC tribopair was higher at 20 N than at 10 N and lower than at 5 N, and specific wear rates of B4C ceramics and the corresponding SiC balls were higher at 20 N than at 10 N. The wear on the B4C ceramics at 20 N was caused mainly by delamination and spalling of tribofilm.
In the case of B4C-SiC ceramics, it can be observed from ) and () that more micro-fracture was found as compared to that at 10 N. Accordingly, the relief structure of B4C-SiC ceramics was further damaged, and the worn surface was further roughened. On the one hand, the bending force between the tribofilm and B4C-SiC ceramics could not bear the shear force from friction, causing the exfoliation of some tribofilm. This phenomenon in which tribofilm peeled off as the load increased was also observed by Pan et al. [Citation34] on the worn surface of B4C-hBN ceramics. On the other hand, the micro-fracture of B4C-SiC ceramics induced by fatigue was increased, causing more wear particles. Meanwhile, the degree of micro-fracture of the corresponding SiC ball increased progressively as the load was increased from 10 N to 20 N ()), and abrasive wear was still dominant on the wear scars of the SiC ball. Therefore, the coefficient of friction of the B4C-SiC/SiC tribopair was higher at 20 N than at 10 N, and the specific wear rates of B4C-SiC ceramics and the corresponding SiC balls were higher at 20 N than at 10 N. The wear on B4C-SiC ceramics at 20 N was caused mainly by micro-fracture.
Because of the rougher worn surfaces of B4C-SiC ceramics, the coefficient of friction of the B4C-SiC/SiC tribopair was higher than that of the B4C/SiC tribopair. On the other hand, the delamination and spalling of tribofilm on the B4C ceramics occurred more easily than the grain pullout and fracture on the B4C-SiC ceramics owing to the clean grain boundaries formed between B4C grains and SiC grains [Citation27], and the wear on B4C ceramics was therefore higher than on B4C-SiC ceramics at 20 N.
Figure 7. SEM images of worn surfaces of B4C (a) and B4C-SiC (c-d) ceramics at 20 N. EDS analysis of Figure 7 (a): (b).
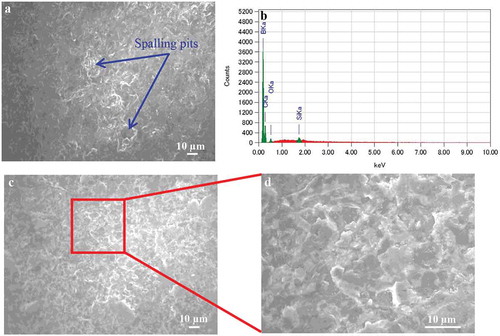
Figure 8. Microscope images of wear scars on SiC balls after sliding against B4C (a) and B4C-SiC (b) ceramics at 20 N.
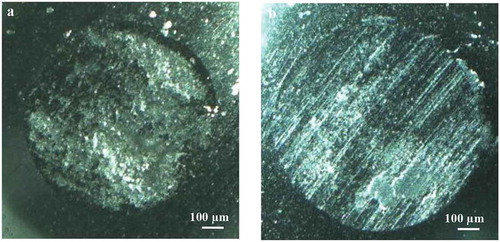
The major wear types for the B4C/SiC and B4C-SiC/SiC tribopairs at different loads are summarized in . For the B4C ceramics, the wear mechanism changed from adhesive wear to delamination and spalling of tribofilm as the load was increased from 5 N to 20 N. For the B4C-SiC ceramics, the wear mechanism changed from surface polishing and mild abrasion to micro-fracture as the load was increased from 5 N to 20 N. At low load, due to the severe adhesive wear and abrasive wear of the B4C/SiC tribopairs and the beneficial influence of the relief structure on the B4C-SiC ceramics, the coefficient of friction of the B4C/SiC tribopair was higher than that of the B4C-SiC/SiC tribopair, and the specific wear rate of B C ceramics was higher than that of B4C-SiC ceramics. At intermediate load, due to the formation of tribofilm on the B4C ceramics, adhesive wear and abrasive wear on the B4C/SiC tribopair were significantly reduced. On the other hand, grain pullout from the B4C-SiC ceramic matrix and micro-fracture caused deterioration of the relief structure to some extent and roughened the worn surface of the B4C-SiC ceramics. The coefficient of friction of the B4C/SiC tribopair was therefore lower than that of the B4C-SiC/SiC tribopair, and no difference between the specific wear rates of B4C ceramics and B4C-SiC ceramics was apparent. At high load, more micro-fracture was caused and the worn surface of B4C-SiC ceramics further roughened. Thus, the coefficient of friction of the B4C-SiC/SiC tribopair was higher than that of the B4C/SiC tribopair. Meanwhile, delamination and spalling of the tribofilm on the B4C ceramics was increased, which occurred easier than the grain pullout and micro-fracture of B4C-SiC ceramics, and the specific wear rate of the B4C ceramics was therefore higher than that of the B4C-SiC ceramics. Finally, based on the findings of this study, the following design basis and future work can be given for B4C and B4C-SiC ceramics sliding against SiC balls under dry sliding conditions: (1) at low load (5 N), B4C-SiC/SiC tribopairs can show a lower coefficient of friction and lower systematic specific wear rate; (2) at intermediate load (10 N), B4C/SiC and B4C-SiC/SiC tribopairs can reveal similar systematic specific wear rates, as a result of which, from the viewpoint of durability and reliability of a tribosystem, B4C/SiC tribopairs with a lower coefficient of friction are more suitable for intermediate load; (3) at high load (20 N), B4C-SiC/SiC tribopairs can exhibit a lower systematic specific wear rate, but a higher coefficient of friction restricts their wide application at high loads. Ways of decreasing the coefficient of friction of B4C-SiC/SiC tribopairs at high loads should therefore be studied further.
Table 3. Wear types for the B4C/SiC and B4C-SiC/SiC tribopairs as a function of load.
4. Conclusions
B4C and B4C-SiC ceramics were studied for dry sliding friction and wear against commercially available SiC balls at 5 N, 10 N and 20 N. The following results were obtained:
When the load was increased from 5 N to 20 N, the average coefficient of friction of the B4C ceramics varied in the range of 0.24–0.44, while the average coefficient of friction of the B4C-SiC ceramics varied in the range of 0.32–0.48; the average specific wear rate of the B4C ceramics varied in the range of 11.0–22.4 × 10−6 mm3/N·m, while the average specific wear rate of the B4C-SiC ceramics varied in the range of 1.3–7.3 × 10−6 mm3/N·m. Both the coefficient of friction and specific wear rate of the B4C ceramics first decreased and then increased with increases in load, while both the coefficient of friction and specific wear rate of the B4C-SiC ceramics increased with increases in load.
At low load, the B4C ceramics showed a higher coefficient of friction and higher specific wear rate due to severe adhesive wear and abrasive wear, while the B4C-SiC ceramics exhibited a lower coefficient of friction and lower specific wear rate due to the relief structure. The specific wear rate of the B4C ceramics was an order of magnitude higher than that of the B4C-SiC ceramics.
At intermediate load, the B4C ceramics showed a lower coefficient of friction due to the formation of tribofilm, while the B4C-SiC ceramics exhibited a higher coefficient of friction due to a certain degree of damage of the relief structure and roughened worn surface. No difference between the specific wear rates of B4C ceramics and B4C-SiC ceramics was apparent.
At high load, B4C-SiC ceramics showed a higher coefficient of friction due to greater micro-fracture. Meanwhile, the delamination and spalling of tribofilm on the B4C ceramics was increased, occurring more easily than the grain pullout and micro-fracture of B4C-SiC ceramics. Clearly then, the specific wear rate of B4C ceramics was higher than that of B4C-SiC ceramics.
Disclosure statement
No potential conflict of interest was reported by the authors.
References
- Thévenot F. Boron carbide- a comprehensive review. J Eur Ceram Soc. 1990;6(4):205–225.
- Zhang W, Yamashita S, Kita H. Progress in pressureless sintering of boron carbide ceramics-A review. Adv Appl Ceram. 2019;118(4):222–239.
- Shipway PH, Hutchings IM. The influence of particle properties on the erosive wear of sintered boron carbide. Wear. 1991;149:85–98.
- Deng JX. Erosion wear of boron carbide ceramic nozzles by abrasive air-jets. Mater Sci Eng A. 2005;408(1–2):227–233.
- Shipway PH, Hogg JJ. Wear of bulk ceramics in micro-scale abrasion-The role of abrasive shape and hardness and its relevance to testing of ceramic coatings. Wear. 2007;263(7):887–895.
- Ortiz AL, Candelario VM, Borrero-López O, et al. Sliding-wear resistance of pure near fully-dense B4C under lubrication with water, diesel fuel, and paraffin oil. J Eur Ceram Soc. 2018;38(4):1158–1163.
- Pan W, Gao YM. Tribological behavior of B4C/hBN ceramic composites coupled with grey iron under the lubrication of emulsion. Mater Res Express. 2018;5(6):066512.
- X Q L, Gao YM, Pan W, et al. Effect of hBN content on the friction and wear characteristics of B4C-hBN ceramic composites under dry sliding condition. Ceram Int. 2015;41(3):3918–3926.
- X Q L, Gao YM, Wei SZ, et al. Dry sliding tribological properties of self-mated couples of B4C-hBN ceramic composites. Ceram Int. 2017;43(1):162–166.
- Alexander R, Ravikanth KV, Bedse RD, et al. Effect of carbon fiber on the tribo-mechanical properties of boron carbide: comparison with carbon nanotube reinforcement. Int J Refract Metals Hard Mater. 2019;85:105055.
- Murthy TSR, Ankata S, Sonber JK, et al. Microstructure, thermo-physical, mechanical and wear properties of in-situ formed boron carbide-zirconium diboride composite. Ceram-Silik. 2018;62(1):15–30.
- Moshtaghioun BM, Ortiz AL, Gómez-García D, et al. Toughening of super-hard ultra-fine grained B4C densified by spark-plasma sintering via SiC addition. J Eur Ceram Soc. 2013;33(8):1395–1401.
- Tkachenko YG, Britun VF, Prilutskii ÉV, et al. Structure and properties of B4C-SiC composites. Powder Metall Met Ceram. 2005;44(3–4):196–201.
- Zhang YL, Song XG, G J L, et al. Oxidation behavior research of the SiC/B4C multiphase ceramics. Appl Mech Mater. 2015;727–728:288–291.
- Zhang W, Yamashita S, Kumazawa T, et al. Influence of surface roughness parameters and surface morphology on friction performance of ceramics. J Ceram Soc Jpn. 2019;127(11):837–842.
- Zhang W, Yamashita S, Kumazawa T, et al. Study on friction behavior of SiC-B4C composite ceramics after annealing. Ind Lubr Tribol. 2020;7. DOI:10.1108/ILT-08-2019-0350.
- Zhang W, Yamashita S, Kita H. Progress in tribological research of SiC ceramics in unlubricated sliding-A review. Mater Des. 2020;190:108528.
- Sharma SK, Kumar BVM, Kim YW. Effect of WC addition on sliding wear behavior of SiC ceramics. Ceram Int. 2015;41(3):3427–3437.
- Sonber JK, Limaye PK, Murthy TSR, et al. Tribological properties of boron carbide in sliding againstWC ball. Int J Refract Metals Hard Mater. 2015;51:110–117.
- Micele L, Palombarini G, Guicciardi S, et al. Tribological behaviour and wear resistance of a SiC-MoSi2 composite dry sliding against Al2O3. Wear. 2010;269(5):368–375.
- Gogotsi YG, Koval’chenko AM, Kossko IA. Tribochemical interactions of boron carbides against steel. Wear. 1992;154:133–140.
- Murthy VSR, Kobayashi H, Tsurekawa S, et al. Influence of humidity and doping elements on the friction and wear of SiC in unlubricated sliding. Tribol Int. 2004;37(5):353–364.
- Cao XQ, Shang LL, Liang YM, et al. The tribological performances of the boron carbide films tested under wet air and wet N2 conditions. Tribol Lett. 2019;67(3):70.
- Anstis GR, Chantikul P, Lawn BR, et al. A critical evaluation of indentation techniques for measuring fracture toughness: I, direct crack measurements. J Am Ceram Soc. 1981;64(9):533–538.
- Wu F, Wang LS, Zhang JS, et al. Tribological properties of hot-pressed boron carbide ceramic. Trans Nonferrous Met Soc China. 2001;11(1):119–122.
- Zhang W, Yamashita S, Kumazawa T, et al. Effect of nanorelief structure formed in situ on tribological properties of ceramics in dry sliding. Ceram Int. 2019;45(11):13818–13824.
- Zhang W, Yamashita S, Kumazawa T, et al. A study on formation mechanisms of relief structure formed in situ on the surface of ceramics. Ceram Int. 2019;45(17):23143–23148.
- Zhang W, Yamashita S, Kita H. Tribological properties of SiC-B4C ceramics under dry sliding condition. J Eur Ceram Soc. 2020;40(8):2855–2861.
- Lörcher R, Telle R, Petzow G. Influence of mechanical and tribochemical properties on the wear behaviour of B4C-TiB2-W2B5 composites. Mater Sci Eng A. 1988;105–106:117–123.
- Zhu HY, Niu YR, Lin CC, et al. Fabrication and tribological evaluation of vacuum plasma-sprayed B4C coating. J Therm Spray Technol. 2012;21(6):1216–1223.
- Cho SJ, Um CD, Kim SS. Wear and wear transition in silicon carbide ceramics during sliding. J Am Ceram Soc. 1996;79(5):1247–1251.
- Dong X, Jahanmir S, Ives LK. Wear transition diagram for silicon carbide. Tribol Int. 1995;28(8):559–572.
- Fischer TE, Zhu Z, Kim H, et al. Genesis and role of wear debris in sliding wear of ceramics. Wear. 2000;245(1):53–60.
- Pan W, Gao YM, X Q L, et al. Tribological behavior of B4C/hBN ceramic composites sliding against gray cast irons without lubrication. Tribol Lett. 2015;60(1):10.