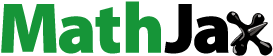
ABSTRACT
Alzheimer’s disease (AD) is the most common cause of dementia. Patients are generally to forget taking pills because of memory loss or to skip medication due to side effects; that might stop the medication and affect the results of the treatment. In this study, we combine donepezil with hydroxyapatite (HAP-DPZ) to deliver by intramuscular (IM) injection, which may prevent from patient to skip the daily medication and keep the medicine at a constant level in the blood to achieve a constant release. HAP particles synthesized by using the new oil-in-water method, the XRD pattern, and the FTIR spectrum were proved the synthesized of HAP. SEM and DLS showed the particle size of synthesized HAP-DPZ was in the range of 0.9–3 μm, which all in the optimal range for cellular uptake. In the drug release profile, DPZ could be released from HAP-DPZ by endosome/lysosome complex to achieve control releasing. In vitro study, there was no cytotoxicity found in HAP-DPZ. In the animal study, only one injection of HAP-DPZ administration, rats revealed well-focused searching strategies with the longest swimming time and most finding times in the quadrant where the platform was initially after three weeks.
1. Introduction
Dementia is a syndrome, usually of a chronic or progressive nature, in which there is deterioration in cognitive function; that is beyond what might be expected from normal aging. It affects memory, thinking, orientation, comprehension, calculation, language, judgment, and learning capacity. The impairment in cognitive function is commonly accompanied, and occasionally preceded, by deterioration in emotional control, social behaviors, or motivation. Dementia is a syndrome characterized by acquired deficits in multiple domains of cognition. For the dementia, the daily life of patients is mainly affected by these severe deficits, not by impaired consciousness or the effects of a systemic illness [Citation1]. Memory is usually the most severely affected domain initially. Progression is evident with increasing impairment of memory developing gradually into completed impairment of cognition, including orientation, language, judgment, perceptual ability, and praxis (the ability to carry out complex actions). These cognitive impairments are accompanied by progressive deterioration in the ability to carry out daily live activities and often by the appearance of challenging behaviors and other psychiatric features. The clinical course is associated with growing disability and dependency on care givers. A characteristic feature of the disease is the widely variable rate of progression in different patients [Citation2].
Alzheimer’s disease (AD) is the most common cause of dementia and may be involved in as many as 80% of the dementia cases. It is a primary degenerative disease of the brain of unknown cause, which leads to dementia of insidious onset, most commonly in later life. AD is a chronic and irreversible neurodegenerative disease characterized by progressive decline in cerebral functions, especially in memory. The brain pathology features progressive loss of neural function, the appearance of amyloid plaques, and neurofibrillary tangles in the brain [Citation3]. The World Health Organization recognizes AD as a global public health priority. The global prevalence of AD will reach 106.2 million by 2050; so that it is urgently needed to develop promising methods to prevent from AD onset or progress. Although the AD onset and pathogenesis were gradually understood since 1907 [Citation4], Alois Alzheimer reported the first case, there were no adequate treatments to be developed to slow- down the AD progress so far. It can be divided into two categories for AD treatment: nonmedical treatment and medical treatment. No matter nonmedical or medical treatment, they are all to improve the quality of life or to maintain the cognitive and daily activity abilities of patients.
Nonmedical treatments, such as coconut oil [Citation5], omega-3 fatty acids [Citation6], coenzyme Q10 [Citation7], and coral calcium [Citation8], etc., though have more safety and cheaper comparing to prescription drugs, there are lack of strong scientific evidence from extensive clinical trial or being approved by health authorities. For the medical treatment, there are many kinds of FDA-approved prescription drugs to treat AD including cholinesterase inhibitors (Razadyne® (galantamine), Exelon® (rivastigmine), and Aricept® (donepezil)), memantine (Namenda® (memantine)), etc. They all have a good effect on AD treatment; however, these drugs can only relieve symptoms temporarily, and none of them has proven the ability to cure or stop the progression of the disease [Citation9]. In addition, the efficiency of the drugs varies from person to person and from stage to stage, and the drugs are always accompanied with side effects such as nausea, diarrhea, vomiting, vertigo, etc [Citation10].
As shown previously, the medical treatments had a very promising and instant results to relieve AD symptoms, but the side effects of the medicine always troubled the researchers and patients. Patients are generally to forget taking pills because of memory loss or to skip medication due to side effects; that might stop the medication and affect the results of the treatment. Furthermore, some of the side effect comes from the way of administration by overdose to keep effective drug concentration in blood. As previous description, the current treatments are all focused on the patient of AD already developed, in which the treatment results are not so promising. We do believe that to develop a way to prevent from AD onset might be the best way to skip the later-on AD treatment by high-dose therapy coming up with less effectiveness [Citation2]. If we could combine a medication with a carrier to deliver by intramuscular (IM) injection one shot one month in clinics outpatient, that may prevent from patient to skip the daily medication and keep the medicine in constant level in blood to achieve as so-called constant release to overcome the mentioned obstacles.
In the study, the hydroxyapatite (HAP, Ca10(PO4)6(OH)2) was synthesized would serve as drug carrier loaded with donepezil as a new formula delivered by IM administration to prevent from AD onset or to slow down the AD progress with less side effect.
HAP is a major inorganic component in bone and teeth. It has been widely used in clinical applications, such as carrier for drug/gene delivery, hyperthermia therapy for cancer treatment, biding to antigen for immunotherapy, and biodegradable MRI contrast agent [Citation11–15], due to excellent biocompatibility and bioactivity [Citation16]. Donepezil is a reversible noncompetitive acetylcholinesterase inhibitor (AChEI), which approved for medical use in the United States in 1996. It is the only prescription drugs till now approved to treat all stages of Alzheimer’s disease [Citation17]; so that it would be used as model drug in the study to combine with HAP to prevent from AD onset and slow down the AD progress.
In this study, the HAP was synthesized by coprecipitation method and then to do the surface modification for better donepezil loading efficiency; where calcium hydroxide (Ca(OH)2), orthophosphoric acid (H3PO4), and foaming agent was added into oil-phase of n-Hexane (CH3(CH2)4CH3) with Tween 80 as surfactant to prepare mesoporous nanosized HAP particles. The new oil-in-water method could create more space and surface area for donepezil loading, which was indicated by previous study [Citation18]. Emulsion processing of HAp particles was initially introduced to refine the clustering and restrict the formation of hard agglomerates [Citation19]. However, this technique is now a subject of great interest not only to prepare an agglomerate-free ceramic powder but also to control the resulting particles’ microstructure and morphology. Indeed, among many different wet processes developed for HAp synthesis, the emulsion method is more efficient in reducing the particle size, controlling the morphology, and limiting the agglomeration of HAp particles [Citation20,Citation21]. Moreover, simplicity and mild synthesis conditions without any high-temperature requirement during the primary procedure, make the emulsion technique an appropriate method compared to other methods.
The donepezil-loaded HAP (HAP-DPZ) were characterized by x-ray diffractometer (XRD), Fourier transform infrared (FTIR), scanning electron microscope (SEM), dynamic light scattering (DLS), Brunauer-Emmett-Teller (BET) surface area analysis, and thermogravimetric analysis (TGA) for crystal structure identification, functional group analysis, microstructure examination, particle size distribution, pore size and porosity, and donepezil loading efficiency, respectively. The in-vitro releasing profile was checked by the solution with pH value of 7.4 and 4.5 to mimic the environment in normal physiological IM tissue and lysosome/endosome complex. WST-1 and LDH assay would be used to evaluate the cytotoxicity and cell survival rate, respectively. Finally, the animal study was to further prove the efficacy and safety of the DPZ-HAP in vivo by Morris water maze and blood analysis, respectively.
The constant release of donepezil would be achieved by cellular activity of endocytosis process. The overall experimental design was schemed as .
2. Materials and methods
2.1. Materials
Calcium hydroxide (Ca(OH)2, Cat. No. 1305–62-0), hydrochloric acid (HCl, No. 7647–01-0), Tween 80, and n-Hexane (CH3(CH2)4CH3, Cat. No. 270504) were obtained from Sigma-Aldrich (St. Louis, MO, USA). Orthophosphoric acid (H3PO4, Cat. No. 7664–38-2) was obtained from T. Baker (Center Valley, PA, USA). Sodium hydroxide (NaOH, Cat. No. 1310–73-2) was obtained from SHOWA (Meguroku, Tokyo, Japan).
2.2. Preparation of HAP-DPZ
In this study, HAP was synthesized by coprecipitation using calcium hydroxide and orthophosphoric acid with stoichiometric molar ratio. In brief, 3.665 g (0.25 M) of calcium hydroxide was added to 95 mL of deionized water. Then, a stoichiometric quantity of 1.95 mL (0.15 M) of orthophosphoric acid solution was added drop-wise at a rate of 0.3 mL/min at 85ºC and magnetically stirred for 24 h. After the addition of the orthophosphoric acid solution, the pH was adjusted to 9 using 1 N NaOH solution to ensure pure HAP precipitation and the desired morphology. For fabrication of HAP-DPZ, 10 mg DPZ (Biopharm international Co., Ltd., Taiwan), 10% hexane, and 0.5% Tween 80 were added into the HAP mixture overnight. Finally, the resulting material was washed with deionized water three times, and dried in an oven overnight at 60ºC.
2.3. Characterization
2.3.1. Crystal structure identification
X-ray diffractometry (XRD, TTRAX III, Rigaku, TX, USA) was used to determine the crystal structure of the synthesized HAP and DZP-HAP. The synthesized HAP particles passing through 200-mesh sieve were collected and mounted onto the sample holder of the X-ray powder diffractometer under Cu KαI radiation (λ = 0.15406 nm), using a Ni filter with a potential of 30 kV and current of 15 mA. Each specimen was scanned in the range from 10° to 70° at a speed of 2°/min. The patterns were analyzed using a model automatched to the international center for the diffraction database using Jade 6.0 software. JCPDS Card No. 09–0432 was used as the standard pattern.
2.3.2. FTIR analysis of HAP-DPZ
Under infrared excitation, different molecules will produce different types of vibration at a specific frequency. In this study, Fourier transform infrared (FTIR) spectra of the synthesized materials, including pure HAP, and HAP-DPZ, were recorded in a FTIR spectrophotometer (Spectrum 100 FTIR Spectrometer, PerkinElmer, USA) at the wavenumber range of 450 to 4000 cm−1.
2.3.3. Morphology of HAP-DPZ
Scanning electron microscopy (SEM) was used to examine the microstructure of the HAP-DPZ. The specimens were gold-sputtered and then mounted onto an adhesive copper stub. SEM analyses were performed using a JSM-7600 F (JEOL, Japan) electron microscope with current and voltage of 20 mA and 10 kV, respectively.
2.3.4. Particle size identification
The particle size of the HAP-DPZ were measured by dynamic light scattering (DLS) at 25°C and incident angle 90°. The zeta potential was determined by DLS associated with electrophoretic mobility at pH 7.4 and incident angle 90°. About 5 mg HAP-DPZ particles were dispersed in 5 mL deionized water for measurements of mean size. Then, the obtained dispersion was vortexed for 30 seconds with five repeats. After complete homogenization, the samples were placed in a cuvette for measurements in a Zeta-sizer Nano ZS (Malvern Instruments Ltd, Worcestershire, UK).
2.3.5. Surface area analysis
The specific surface area and pore size distribution of HAP was determined by BET (Micromeritics ASAP2010, Georgia, USA) using nitrogen gas adsorption−desorption isotherms. The porosity of HAP was also determined by a mercury porosimeter (Micromeritics Autopore 9520, Georgia, USA).
2.3.6. Drug loading capacity and release profile
Thermogravimetric analysis or thermal gravimetric analysis (TGA) is a method of thermal analysis in which the mass of a sample is measured over time as the temperature changes. This measurement provides information about physical phenomena, such as phase transitions, absorption, and desorption, as well as chemical phenomena, including chemisorptions, thermal decomposition, and solid-gas reactions (e.g. oxidation or reduction) [Citation22]. In this study, we compared the weight loss percentage of free DPZ with that of HAP-DPZ (temperature range, 30 ~ 800°C; heating rate, 5°C/min) to examine the amount of drug loaded [Citation23].
In vitro release of DPZ was carried out in phosphate buffer solution (PBS) at pH 4.5 and 7.4, defined as two distinct dissolution media. In brief, the 1 g HAP-DPZ was suspended in 5 mL of PBS in a centrifugation tube (15 mL). The temperature was maintained at 37 ± 0.5°C, with the rotation frequency maintained at 100 rpm/min. Supernatants were quantified the release every 4 h in the first 24 h, and collected after centrifugation every day over a period of 7 days. Then, the DPZ concentration was quantified in an UV spectrometer (V-630, Jasco; Easton, MD, USA) at 264 nm.
2.4. In vitro study
2.4.1. Cell viability
The biocompatibility of the HAP-DPZ was evaluated using the water-soluble tetrazolium (WST-1) assay in the 3T3 cell line from BCRC, Taiwan. The biocompatibility of the as-prepared composite was tested according to the ISO 10993–5 standard. An extract medium was prepared by adding 0.2 g/mL of the HAP, 0.2 g/mL of the HAP-DPZ, and 46.5 ng/mL of DPZ [Citation24] to high-glucose DMEM (Sigma, USA), followed by incubation at 37ºC for 24 h. The cells were then seeded in 96-well plates at a cell density of 5 × 103 cells/well and incubated at 37ºC for 1 day. The culture medium was then replaced with the extract medium, and samples and cells were incubated for 1–3 days. Before the assay, 10 μL WST-1 reagent was added into each well for 4 h. After incubation, the plate was placed in a spectrophotometric plate reader (ELISA reader, Tecan Sunrise, Switzerland) set to read the absorbance at 450 nm (with a reference filter at 600 nm) to determine the amount of Formazan formed, which directly correlates to the number of metabolically active cells. The percentage of cell viability was calculated by the following EquationEquation (1)(1)
(1) :
2.4.2. Cytotoxicity
Cytotoxicity was evaluated by measuring the extracellular lactate dehydrogenase (LDH) content using the CytoTox 96 Assay Kit (Promega Corporation, USA). Briefly, suspension medium was transferred to a new enzymatic assay plate and LDH substrate solution was added. After 30 min, stop solution was added to each well in the plate, and the absorbance at 490 nm was measured on a spectrophotometric plate reader (ELISA reader, Tecan Sunrise, Switzerland). The percentage of cytotoxicity was calculated by the following EquationEquation (2)(2)
(2) :
2.5. In vivo study
2.5.1. Animals
Adult male Wistar rats (8 weeks of age, 200–250 g) were stayed in a room with temperature and humidity at 23°C and 30%–70%, respectively, under 12 h-light/12 h-darkness cycle, standard diet and water ad libitum. All animals were purchased from BioLASCO Taiwan Co., Ltd. All animal experiments were carried out in compliance with the guidelines of National Taiwan University, College of Medicine, Institutional Animal Care and Use Committee (IACUC). Prior to the experiments, the experimental protocol was approved by the IACUC (no. 20130429). The animals were maintained following the Guide for the Care and Use of Laboratory Animals. The surgical procedures performed in this study were approved by the Animal Ethics Committee, National Taiwan University Hospital, Taiwan.
2.5.2. Induction of experimental AD dementia by i.c.v. administration of STZ in rats
The rats were first anesthetized with intraperitoneal injection of Zoletil and Rompum (1 mL/kg, Virbac, France) at a 1:2 concentration ratio. Then, STZ was dissolved in normal saline shortly before being injected bilaterally into the ventricles (3 mg/kg, 10 μL/injection site) by stereotaxic surgery through a Hamilton syringe. The following coordinates were used for intracerebroventricular injection: 0.8 mm posterior to the bregma, 1.5 mm lateral to the sagittal suture, and 3.6 mm ventral from the surface of the brain. Then, the behavior test was performed 14–20 days after AD induction.
2.5.3. HAP-DPZ treatment in vivo
The rats were divided into three groups: (1) normal rat (control), (2) Alzheimer’s disease induced rat (ADI), and (3) the rat treated with HAP-DPZ followed by AD induction (HD-ADI).
In the ADI group, the rats were injected by intracerebroventricular (i.c.v.) streptozotocin (STZ) at day-1 and day-3 as mentioned in section 2.5.2.
In the HD-ADI group, 0.5 g of HAP-DPZ suspended in 1 mL of PBS was firstly injected into the muscle of the rats prior to STZ i.c.v treatment to prevent from AD development.
2.5.4. Morris water maze
The Morris water maze is a test of spatial learning for rats [Citation25]. A circular pool is divided into four quadrants, with a submerged platform placed in one of the quadrants. For each training session, the rat was gently placed in water at a different drop location and allowed to find the submerged platform; the rat was guided toward the platform if it could not find the platform within 2 minutes. Once the platform was reached, the rat was allowed to stay on it for 30 seconds. After six training sessions, the time took by the rat to reach the escape platform was recorded. Moreover, on the last training day, the memory study was conducted with the platform hidden. The time spent at the quadrant without platform was also recorded. The design of animal study as showed in .
2.6. Statistical analysis
Statistical significance was determined by one-way ANOVA and Tukey’s test for post hoc multiple comparison A p < 0.05 was considered as statistically significant.
3. Results
3.1. Crystal structure identification of synthesized HAP-DPZ by XRD
was the x-ray pattern of the synthesized HAP-DPZ; where the characteristic diffraction peaks on 2θ of 25.9°, 28.1°, 30°, 31.8o, 32.2°, 32.9°, 34°, 39.8°, 46.7°, and 49.5° corresponding to (002), (102), (210), (211), (112), (300), (202), (310), (222), and (213), respectively, was fully matched to standard HAP pattern of JCPDS No. 090432. The crystal structure was identified as pure hydroxyapatite without second phase to be traced in the pattern. From the results, we could tell that the crystal structure of synthesized HAP-DPZ by the new water-in-oil method was exact the same with that of HAP prepared by conventional method.
3.2. FTIR analysis
shows the FTIR spectrum of hydroxyapatite, DPZ, and HAP-DPZ. The characteristic absorption bands of HAP located in the 600–1100 cm−1 region. The asymmetric stretching bending of the (PO4)3- group was found at 1089 cm−1, 910–1040 cm−1, and 560 cm−1. The absorption band of C = O at 1687 cm−1 and absorption band of C-N at 1317 cm−1 were specifically observed on the DPZ spectrum. Additionally, there is no characteristic absorption bands of Tween 80 found in 2926 cm−1, 2868 cm−1, and 1742 cm−1.
3.3. Morphology and particle size of HAP-DPZ
The synthesized HAP-DPZ were examined under SEM. All the grains showed an spheroid shape with grain size of 100 nm in average as shown in ). There were lots of pores in-between the grains left more surface area and space for DPZ accommodation.
) is the DLS analysis of the HAP-DPZ. The size distribution is in the range of 0.9 μm and 3 μm with average diameter of 1.78 μm due to aggregations. The average particle size falls in the optimal particle size of 0.5–5 μm for cellular endocytosis. We could expect that the HAP-DPZ particles would be gradually uptake by defense cells to achieve constant release.
The BET analysis was shown in . The average pore size and porosity of HAP-DPZ was in 229.9316 nm and 50.1%, respectively; that was higher than the that of HAP prepared by traditional method, for better DPZ loading efficiency.
Figure 5. (a) SEM images of HAP-DPZ at 20 k ×. The images show an open and interconnected porous structure within the HAP-DPZ. (b) Size distribution of HAP-DPZ particles analyzed by DLS. Data indicate that the particle size was in the range of 900−2000 nm
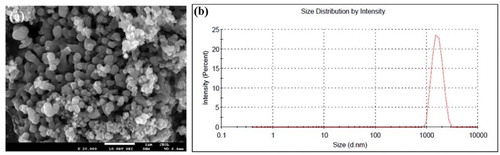
Table 1. BET surface area and porosity of meso-HAP. Where the BET surface area was 26.0947 m2/g, langmuir surface area was 40.5799 m2/g, and the porosity was 50.1%
3.4. The measurement of loading capacity of DPZ on HAP particles
TGA was used to evaluate how much of DPZ loaded on HAP particles. The flash point of DPZ is 273.1 ± 30.1°C [Citation26]. The weight loss of the synthesized HAP-DPZ around 250°C would be in terms of the amount of DPZ loaded on HAP particles. As shown in , there was no weight loss in the temperature range of 25°C–800°C. On the contrary, the weight loss of HAP-DPZ around the 250°C was 13% approximately; that would be the amount of DPZ loaded on HAP particles. The loading capacity of DPZ by meso-HAP also compared to HAP synthesizing by a simple coprecipitation method, which indicated in supplementary Figure 1.
3.5. The release profile of DPZ from HAP-DPZ
In vitro release of DPZ was carried out using PBS at pH 4.5 and 7.4. The cumulative DPZ release from HAP-DPZ was shown in .
In the pH 4.5 to mimic the environment of endosome/lysosome complex, the HAP-DPZ was dissolved completely and released all the DPZ within 1 day.
At the environment of pH 7.4 similar to normal physiological condition, the HAP-DPZ was relatively stable without dissolution. At the first 2 days, DPZ released from HAP-DPZ something like an initial burst due to physical adsorption. Thereafter, there was no DPZ to be released. We believe that DPZ absorbed to and keep in the pores in-between the HAP nanograins; that might not be released until stayed in acid environment and dissolved, such as go through endocytic process to stay in the endosome/lysosome complex.
Figure 7. Cumulative DPZ release from HAP particles. DPZ absorbance was measured spectrophotometrically at 264 nm. At pH 7.4, 15% of DPZ was released from HAP-DPZ in the first day, and no further DPZ was released from HAP-DPZ thereafter. When in pH 4.5, HAP-DPZ was dissolved, and 90% of DPZ was released in the first day
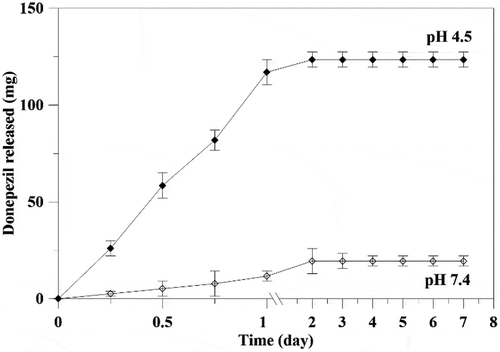
3.6. The evaluation of cell viability and cytotoxicity of HAP-DPZ
WST-1 and LDH assays were used to evaluate the cell viability and cytotoxicity of HAP-DPZ by 3T3 fibroblasts, respectively, at day 1, day 2, and day 3.
The ) was the results of the cell viability for 3 days by WST-1 assay. There was no significant difference in cell viability for the three test groups of HAP, DPZ, and HAP-DPZ.
The ) was the results of cytotoxicity by LDH assay. We could see that the cytotoxicity of the test groups was all less than 4%.
Based on the regulation of ISO-10993, we believe that the synthesized HAP-DPZ would be no toxicity to the 3T3 fibroblasts.
Figure 8. (a) Cell viability and cytotoxicity tests for HAP-DPZ. WST-1 test in 3T3 cells was performed using HAP-DPZ at days 1, 2, and 3 (n = 6). Data suggest no significant effect on cell viability by HAP-DPZ. (b) LDH test was performed in 3T3 cells at days 1, 2, and 3 (n = 6). The results suggest that HAP-DPZ did not induce cytotoxicity
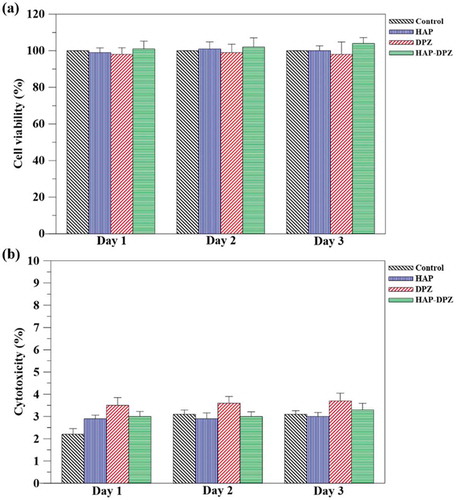
3.7. Morris water maze
Morris water maze was performed to evaluate the spatial memory of rats induced with AD by i.c.v. administration of STZ. The rats of the HAP-DPZ group quickly found the escape platform in Morris water maze. Furthermore, when we hide the platform, the rats of the HAP-DPZ group had longer retention time at the quadrant in which the platform was formerly located compared to the retention time of the AD group (). Relative to the control group (without AD), was 50% of the HAP-DPZ retention time.
Figure 9. Learning and memory ability of rats by Morris water maze test. The times of spatial retention in the section with the hidden platform during the probe trial (n = 6). The time spent by rats of the HAP-DPZ group in the section with the hidden platform was much lower compared with that of rats of the AD group. (*** is p < 0.001 comparing to control, ** is p < 0.01 comparing to control, # is p < 0.05 comparing to ADI)
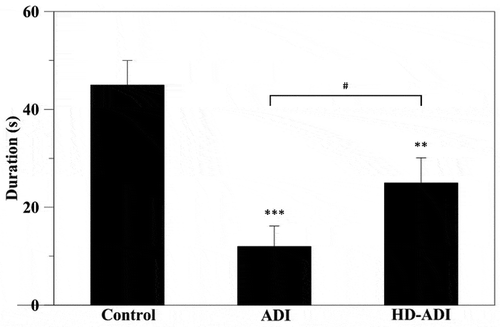
4. Discussion
In this study, calcium hydroxide (Ca(OH)2), orthophosphoric acid (H3PO4), and foaming agent was added into oil-phase of n-Hexane (CH3(CH2)4CH3) with Tween 80 as surfactant to prepare meso-porous nanosized HAP particles. The new oil-in-water method could create more space and surface area for donepezil loading. The HAP was then to do the surface modification for better donepezil loading efficiency. The XRD pattern and FTIR spectrum were proved the synthesized HAP-DPZ were hydroxyapatite [Citation27–29], as shown in . We also prove that the synthesized HAP could have more space for DPZ accommodation due to high porosity and surface area based on the results of BET (). The crystallinity is no significance between the traditional HAP and developed HAP-DPZ. The traditional HAP mixed with DPZ is just a physical adsorption that would be very easy to be released out in short-term and no function on control release. The DPZ in developed HAP-DPZ is not only surface adsorption, but also enclosed in-between the grain boundary and entrapped in lattice; the later DPZ is responsible for the control and slow release. The DPZ entrapped in grain boundary and crystal lattice was not possible to be released just based on diffusion control. The release of the DPZ in grain boundary and crystal lattice have to combine with the process of cellular endocytosis; where the HAP-DPZ particles in the endosome/lysosome complex would be quickly dissolved due to the extremely low pH value in the complex environment. The DPZ would escape from the complex and then pump out to the extra-cellular matrix to deliver the DPZ to the whole body by the nearest blood vessel.
According to S. Zhang et al. [Citation30] reported that provides a thermodynamic model for the many nanoparticles systems, with which they demonstrate that the cellular uptake is strongly size dependent and the optimum particle size for cellular uptake or endocytosis is 0.5–5 μm [Citation31].
The results of the analysis of SEM and DLS all showed that the particle size of synthesized HAP-DPZ was in the range of 0.9–3 μm as shown in (a,b), respectively. The range of the synthesized HAP-DPZ particles fall in the optimal range for cellular uptake.
After the HAP-DPZ particles uptake by the cells, the particles would be entrapped in the endosome and then promptly merged with lysosome turned into endosome/lysosome complex with pH value of 3–5. In the complex, the HAP-DPZ particles would be fully dissolved; where the high osmotic pressure would be created due to extremely high concentration of Ca+2 and PO4−3 in the complex. The complex was then broken off because of the osmotic pressure. DPZ would release to cytoplasm and pump-out to intercellular space to deliver to the nearest blood vessels and circulate to brain to relive the symptoms from AD disease. In the study of DPZ releasing profile (), we could know that the DPZ is not so easily to release in the generally physiological environment (pH 7.4), instead, it was completely released in the acidic environment (pH 4.5) such as in endosome/lysosome complex. The similar mechanism has been used in the other applications in our lab, for instance, anti-depression, diabetes mellitus, osteoporosis, etc. [Citation32,Citation33]. Supplementary Figure 2 presents the mechanism of HAP-DPZ, which would be uptake by endocytosis and released by the endosome/lysosome complex.
In this study, we successfully synthesized HAP particles using the new oil-in-water method, which is the main mineral component of bones and teeth. As a biomaterial, it is widely used for bone repair, being biocompatible and nontoxic to the human body () [Citation34,Citation35]. Significant differences in the morphological properties as a result from different manufacturing methods should be taken into consideration: HAP nanoparticles with lower crystallinity and high purity are reported to be more biocompatible for long-term use in the body [Citation36]. The controlled release mechanism of DPZ from HAP has been presented in our previous section and study [Citation27].
Most drugs for AD treatment, including DPZ, are designed to be highly hydrophobic because the target region of these drugs is usually inside the brain; therefore, these drugs are designed to pass through the blood-brain barrier (BBB). Only hydrophobic and small molecule drugs can go through the BBB by blood circulation system [Citation37,Citation38]. DPZ is the leading compound for the treatment of AD in more than 50 countries. Compared with other conventional acetylcholinesterase inhibitors (AChEIs), DPZ is a highly selective and reversible piperidine derivative with AChEI activity that exhibits the best pharmacological profile in terms of cognitive improvement, responders rate (40–58%), dropout cases (5–13%), and side effects (6–13%) in AD [Citation39].
The Morris water maze test was used to assess the spatial learning and memory of rats (). Compared with the control rats, ADI rats displayed a significant difference in the escape latency, demonstrating that the STZ injected bilaterally into ventricles by stereotaxic surgery through a Hamilton syringe could effectively induce deficits in learning performance. In contrast, HD-ADI rats presented significantly shorter escape latency than ADI rats and presented no significant difference in learning behavior to the rats in the control group, demonstrating significant improvement on the rats’ performances after HAP-DPZ administration. Moreover, on the last day, the memory study was conducted with the platform hidden. ADI rats demonstrated poorly focused platform searching performance, whereas control rats and HD-ADI rats revealed well-focused searching strategies with the longest swimming time and most finding times in the quadrant where the platform was initially.
5. Conclusion
As previous description, the current AD treatments are all focused on the patients those AD already developed, in which the treatment results are not so promising. We do believe that to develop a way to prevent from AD onset might be the best way to skip the later-on AD treatment by high-dose therapy coming up with less effectiveness. In this study, we developed a new oil-in-water process to synthesize HAP as DPZ carrier for AD disease. The average grain size of the synthesized HAP were 100 nm. The synthesized HAP grains would aggregate into particles left lots of pores in-between the grains for DPZ loading with particle size in the range of 0.9–3 μm that was an optimum size for cellular uptake. The particles would be dissolved in acidic environment to make DPZ fully released out to the intercellular space and deliver through the blood circulation system to relieve AD symptoms. The study was to deliver HAP-DPZ particles by intramuscular (IM) injection with one shot one month. The result of Morris Water Maze proved that the designed HAP-DPZ could prevent rats from AD development.
In the future, the combination may prevent from patient to skip the daily medication and keep the medicine in constant level in blood to achieve as so-called constant release to overcome the obstacles mentioned in Introduction.
Author contributions
CHF collected the data, executed the majority of the experiments, and drafted the manuscript. YWL contributed to the active discussion of experimental design and performing of the animal study. FHL supervised the study, assisted with study conceptualization, and made a substantial contribution to the revision of the manuscript. All authors have read and approved the final submitted manuscript.
Ethics approval
All animal procedures and experiments were performed following a protocol approved by the National Taiwan University College of Medicine and Public Health Institutional Animal Care and Use Committee (IACUC no. 20130429).
Supplemental Material
Download MS Word (127.6 KB)Acknowledgments
The authors would like to express their immense gratitude to the Institute of Biomedical Engineering, National Taiwan University, Ministry of Science and Technology, and the National Taiwan University Hospital for the space support.
Disclosure statement
The authors declare that they have no competing interests.
Supplementary material
Supplemental data for this article can be accessed here.
Availability of data and material
The datasets used and/or analyzed in the current study are available from the corresponding author on reasonable request.
Additional information
Funding
References
- Chertkow H, Feldman HH, Jacova C, et al. Definitions of dementia and predementia states in Alzheimer’s disease and vascular cognitive impairment: consensus from the Canadian conference on diagnosis of dementia. Alzheimer’s Res Ther. 2013;5(1):S2.
- Ritchie CW, Russ TC, Banerjee S, et al. The Edinburgh Consensus: preparing for the advent of disease-modifying therapies for Alzheimer’s disease. Alzheimer’s Res Ther. 2017;9(1):85.
- Ryan NS, Rossor MN, Fox NC. Alzheimer’s disease in the 100 years since Alzheimer’s death. Brain. 2015;138(12):3816–3821.
- Alzheimer A, Stelzmann RA, Schnitzlein HN, et al. An english translation of Alzheimer’s 1907 paper, “Uber eine eigenartige Erkankung der Hirnrinde”. Clin Anat. 1995;8(6):429–431.
- de la Rubia Orti JE, Garcia-Pardo MP, Drehmer E, et al. Improvement of main cognitive functions in patients with Alzheimer’s disease after treatment with coconut oil enriched mediterranean diet: a pilot study. J Alzheimers Dis. 2018;65(2):577–587.
- Canhada S, Castro K, Perry IS, et al. Omega-3 fatty acids’ supplementation in Alzheimer’s disease: a systematic review. Nutr Neurosci. 2018 Oct;21(8):529–538.
- Yang X, Zhang Y, Xu H, et al. Neuroprotection of coenzyme Q10 in neurodegenerative diseases. Curr Top Med Chem. 2016;16(8):858–866.
- Marcason W. What is the lowdown on coral calcium? J Acad Nutr Diet. 2003;103(10):1319.
- Zhang M, Schmitt-Ulms G, Sato C, et al. Drug repositioning for Alzheimer’s disease based on systematic ‘omics’ data mining. PLoS One. 2016;11(12):e0168812.
- Lleó A. Current therapeutic options for Alzheimer’s disease. Curr Genomics. 2007;8(8):550–558.
- Hu J, Liu Z-S, Tang S-L, et al. Effect of hydroxyapatite nanoparticles on the growth and p53/c-Myc protein expression of implanted hepatic VX2 tumor in rabbits by intravenous injection. World J Gastroenterol. 2007;13(20):2798.
- Chen X, Deng C, Tang S, et al. Mitochondria-dependent apoptosis induced by nanoscale hydroxyapatite in human gastric cancer SGC-7901 cells. Biol Pharm Bull. 2007;30(1):128–132.
- Yen K-C, Chen I-H, Lin F-H. Preparation of calcium phosphate nanoparticles as non-viral vectors for gene delivery system. Biomed Eng Appl Basis Commun. 2017;29(4):1750027.
- Chang K-C, Li C-Y, Hsu C-K, et al. Synthesis and properties of Fe-modified calcium-deficient hydroxyapatite nanocrystal for MRI contrast agent. Biomed Eng Appl Basis Commun. 2011;23(5):393–401.
- Wu H-C, Lin F-H. Evaluation of magnetic-hydroxyapatite nanoparticles for gene delivery carrier. Biomed Eng Appl Basis Commun. 2010;22(1):33–39.
- Matsumoto T, Okazaki M, Nakahira A, et al. Modification of apatite materials for bone tissue engineering and drug delivery carriers. Curr Med Chem. 2007;14(25):2726–2733.
- Zhang N, Gordon ML. Clinical efficacy and safety of donepezil in the treatment of Alzheimer’s disease in Chinese patients. Clin Interv Aging. 2018;13:1963–1970.
- Lim GK, Wang J, Ng SC, et al. Processing of hydroxyapatite via microemulsion and emulsion routes. Biomaterials. 1997 Nov;18(21):1433–1439.
- Murray MGS, Wang J, Ponton CB, et al. An improvement in processing of hydroxyapatite ceramics. J Mater Sci. 1995 Jun 15;30(12):3061–3074.
- Zhou WY, Wang M, Cheung WL, et al. Synthesis of carbonated hydroxyapatite nanospheres through nanoemulsion. J Mater Sci-Mater M. 2008 Jan;19(1):103–110.
- Pradeesh TS, Sunny MC, Varma HK, et al. Preparation of microstructured hydroxyapatite microspheres using oil in water emulsions. B Mater Sci. 2005 Aug;28(5):383–390.
- Coats A, Redfern J. Thermogravimetric analysis. A review. Analyst. 1963;88(1053):906–924.
- Elati CR, Vankawala PJ, Chalamala SR, et al. Polymorphic study of donepezil hydrobromide. 2005.
- Shin CY, Kim HS, Cha KH, et al. The effects of donepezil, an acetylcholinesterase inhibitor, on impaired learning and memory in rodents. Biomol Ther (Seoul). 2018 May 1;26(3):274–281.
- Vorhees CV, Williams MT. Morris water maze: procedures for assessing spatial and related forms of learning and memory. Nat Protoc. 2006;1(2):848.
- Chuong MC, Kelley CJ, Muhammad Y, et al. Investigating effect of water of hydration on active pharmaceutical ingredients in a water-sensitive dosage form. J Anal Sci Technol. 2018;9(1):7.
- Brundavanam RK, Poinern GEJ, Fawcett D. Modelling the crystal structure of a 30 nm sized particle based hydroxyapatite powder synthesised under the influence of ultrasound irradiation from X-ray powder diffraction data. Am J Mater Sci. 2013;3(4):84–90.
- Arsad MS, Lee PM, Hung LK, editors. Synthesis and characterization of hydroxyapatite nanoparticles and β-TCP particles. 2nd International Conference on Biotechnology and Food Science IPCBEE; Singapore: IACSIT Press; 2011.
- Venkateswarlu K, Sreekanth D, Sandhyarani M, et al. X-ray peak profile analysis of nanostructured hydroxyapatite and fluorapatite. International Journal of Bioscience, Biochemistry and Bioinformatics. 2012:389–393. DOI:10.7763/IJBBB.2012.V2.139
- Zhang S, Li J, Lykotrafitis G, et al. Size-dependent endocytosis of nanoparticles. Adv Mater. 2009;21:419–424.
- Kou L, Sun J, Zhai Y, et al. The endocytosis and intracellular fate of nanomedicines: implication for rational design. Asian J Pharm Sci. 2013;8(1):1–10.
- Shyong YJ, Tsai CC, Lin RF, et al. Insulin-loaded hydroxyapatite combined with macrophage activity to deliver insulin for diabetes mellitus. J Mater Chem B. 2015 Mar 21;3(11):2331–2340.
- Shyong Y-J, Wang M-H, Kuo L-W, et al. Mesoporous hydroxyapatite as a carrier of olanzapine for long-acting antidepression treatment in rats with induced depression. J Control Release. 2017;255:62–72.
- Zhou H, Lee J. Nanoscale hydroxyapatite particles for bone tissue engineering. Acta Biomater. 2011 Jul;7(7):2769–2781.
- Swetha M, Sahithi K, Moorthi A, et al. Biocomposites containing natural polymers and hydroxyapatite for bone tissue engineering. Int J Biol Macromol. 2010 July 1;47(1):1–4.
- Oberbek P, Bolek T, Chlanda A, et al. Characterization and influence of hydroxyapatite nanopowders on living cells. Beilstein J Nanotechnol. 2018;9:3079–3094.
- Tao X, Li Y, Hu Q, et al. Preparation and drug release study of novel nanopharmaceuticals with polysorbate 80 surface adsorption. J Nanomater. 2018;2018.
- Uhr M, Grauer MT, Holsboer F. Differential enhancement of antidepressant penetration into the brain in mice with abcb1ab (mdr1ab) P-glycoprotein gene disruption. Biol Psychiatry. 2003;54(8):840–846.
- Cacabelos R. Donepezil in Alzheimer’s disease: from conventional trials to pharmacogenetics. Neuropsychiatr Dis Treat. 2007;3(3):303.