ABSTRACT
La2CoMnO6 and Nd2CoMnO6 powders were successfully synthesized by applying the polyvinyl alcohol (PVA) sol-gel method. The synthesized nanoparticles were characterized by DSC, TG, FT-IR, XRD, Raman spectroscopy, and SEM. XRD analysis revealed that single phase La2CoMnO6 powders was directly synthesized through the charring procedure and no intermediate phase appeared. However, the well-crystallized monophasic Nd2CoMnO6 was synthesized at a calcination temperature of 600°C for 2 h. La2CoMnO6 and Nd2CoMnO6 powders showed paramagnetic behaviors at room temperature.
1. Introduction
The double perovskite oxides, which was usually expressed in the form A2BB’O6 (where A represents lanthanide ion and B and B’ represents transition metals ions) have attracted much attention in recent decades due to their magnetoresistance effect, magnetodielectric properties, magnetocaloric effect, multiferroic properties, and catalytic properties [Citation1–5]. Among them, La2CoMnO6 has received special attention recently. La2CoMnO6 is a ferromagnetic insulator with a Curie temperature of about 225 K [Citation6]. The substitution of the La ion by another rare earth element with a smaller ionic radius significantly changes their magnetic structure and properties [Citation7]. As the ionic radius of the rare earth element decreases, the ferromagnetic Curie temperature decreases gradually, along with the structural distortion [Citation8–11]. In addition, different synthesis methods resulted in complicated crystal structures [Citation6,Citation12]. As we can see, the conventional solid-state reaction method is commonly employed in the preparation of R2CoMnO6 (R = La, Nd) at high temperature [Citation8,Citation11,Citation13,Citation14]. In the meantime, the wet chemical synthesis of R2CoMnO6 was also reported. Various wet chemical methods, such as citric acid sol-gel method [Citation7,Citation12,Citation15–19], ethylene glycol sol-gel method [Citation4,Citation20,Citation21], polymeric precursor method [Citation6,Citation22], glycine-nitrate combustion method [Citation23–26], urea-nitrate combustion method [Citation27], nitrate decomposition method [Citation9,Citation10,Citation28–30], etc. have been employed to prepare R2CoMnO6. For example, Dass and Goodenough [Citation6] synthesized La2CoMnO6 using the polymeric precursor method, and the formation conditions of single-phase La2CoMnO6 was 600°C for 12 h. Liu et al. [Citation21] reported the synthesis of phase pure La2CoMnO6 via ethylene glycol sol-gel method at 600°C. Silva et al. [Citation22] synthesized monophasic La2CoMnO6 by polymeric precursor method at 900°C for 16 h. Joy et al. [Citation23] have prepared LaMn0.5Co0.5O3 using the glycine-nitrate method and single-phase LaMn0.5Co0.5O3 was obtained at 500°C for 12 h. They [Citation24] also reported the synthesis of NdMn0.5Co0.5O3 using the glycine combustion method. Even calcined at 700°C for 12 h, a peak from hexagonal Nd2O3 can still be found in the XRD patterns, although the authors attributed the peak to superlattice reflection [Citation24]. Sayed et al. [Citation26] synthesized La2CoMnO6 by glycine combustion method. The obtained powders were amorphous and monophasic La2CoMnO6 can be formed at 650°C for 2 h [Citation26]. La2CoMnO6 was also reported to be synthesized by the molten-salt method and single-phase La2CoMnO6 was obtained at 700°C for 6 hours [Citation31,Citation32]. Obviously, La2CoMnO6 can be prepared by various methods, but the preparation temperatures are relatively higher. On the other hand, the report on the wet chemical synthesis of Nd2CoMnO6 is very few. Low-temperature synthesis of La2CoMnO6 and Nd2CoMnO6 can save energy. In addition, La2CoMnO6 and Nd2CoMnO6 powders synthesized at low temperatures have smaller particle sizes and may show properties different from those of the bulk, for example, certain ferromagnetic nanoparticles show superparamagnetism. Therefore, it is necessary to synthesize La2CoMnO6 and Nd2CoMnO6 at low temperature. The aim of this work is to synthesize La2CoMnO6 and Nd2CoMnO6 at low temperatures by a different method.
As we know, the PVA sol-gel method has been applied in the preparation of various metal oxide powders [Citation33–35]. In this work, La2CoMnO6 and Nd2CoMnO6 were synthesized with the PVA route. Nanocrystalline La2CoMnO6 with average crystallite size ~20 nm was directly obtained during the charring procedure, while single-phase, nanosized Nd2CoMnO6 powders was synthesized at 600°C.
2. Experimental
La2O3, Nd2O3, Co(NO3)2⋅6H2O, MnCO3 and PVA (MW = 79000) were used as starting materials. The sol-gel preparation procedure of La2CoMnO6 and Nd2CoMnO6 is as follows: First, 5 wt% PVA solution and nitrate solution were prepared, respectively. The 5 wt% PVA aqueous solution was made by dissolving PVA powder into deionized water at 80°C. On the other hand, La2O3 or Nd2O3 as well as MnCO3 powders were dissolved in nitric acid, along with the addition of stoichiometric Co(NO3)2⋅6H2O, to form nitrate solution. Afterward, the above two solutions were mixed with stirring at 80°C until the transparent gel was formed. For 1 mol La2CoMnO6 or Nd2CoMnO6, a 6 mol PVA monomer was used [Citation35]. The samples were charred in an oven at 250°C for 2 h and the obtained powders were referred to as precursor. Finally, the precursors were calcined at 500°C to 700°C for 2 h.
The thermal decomposition behavior of the precursor was studied by applying differential scanning calorimetry (DSC) and thermogravimetric (TG) analysis on STA 449 F3 Jupiter (Netzsch, Germany) with a heating rate of 10°C/min. The Fourier transform infrared spectra of La2CoMnO6 and Nd2CoMnO6 precursor and powders were collected by using Vertex 70 FT-IR spectrometer (Bruker Optik GmbH, Ettlingen, Germany). The phase purity of the obtained powders was characterized using X’Pert PRO X-ray diffractometer (PANalytical B.V., Almelo, Netherland) with Cu Kα radiation. The Raman spectra were collected at room temperature with 532 nm Nd-YAG laser excitation using a LabRAM HR800 single-stage spectrometer (Horiba JobinYvon Ltd., France). The morphology and size analysis of La2CoMnO6 and Nd2CoMnO6 powders was carried out by field emission scanning electron microscope (FESEM) (GeminiSEM 300, Carl Zeiss, Germany). A physical property measurement system (PPMS) from Quantum Design (San Diego, CA) was used to study the magnetic properties of La2CoMnO6 and Nd2CoMnO6 powders with magnetic fields up to 2 T.
3. Results and discussions
displays the simultaneous DSC and TG curves of La2CoMnO6 precursor. The DSC curve shows no obvious exothermic or endothermic peak, indicating that the reaction of forming La2CoMnO6 is complete. The later XRD patterns show that phase pure La2CoMnO6 has formed. A slight weight loss of 7.7% can be observed in the TG curve until the temperature reaches 1000°C, for the reason that the adsorbed moisture is gradually evaporated and residual organics is volatilized during the heating process.
The results of simultaneous DSC and TG for Nd2CoMnO6 precursor are presented in . A small exothermic peak around 292°C in the DSC curve can be observed, which is associate with the oxidative decomposition of the majority of the organic material. On the other hand, the TG curve shows two-weight losses. The first weight loss of 5.4% below 100°C may associate with the vaporization of adsorbed moisture and the second weight loss of 4.6% between 100°C and 650°C can be attributed to the combustion of organics and the release of gases. After the temperature increased to more than 650°C, the sample weight gradually stabilized and remains almost unchanged.
displays the XRD patterns of La2CoMnO6 precursors and powders calcined at 500–700°C for 2 h. It can be seen that the single-phase La2MnCoO6 is formed directly in the precursor, and no peak from the unexpected intermediate phase is observed. All the diffraction peaks can be assigned to the monoclinic crystal structure [Citation6], as indexed in the figure. The XRD patterns of La2CoMnO6 precursor calcined at temperatures ranging from 500°C to 700°C for 2 h showed no significant change, except a slight-increased intensity, indicating an increase in crystallinity. The crystallite size of La2CoMnO6 powder was estimated using the Scherrer formula: the crystallite size of La2CoMnO6 precursor and powders calcined at 500°C, 600°C, and 700°C for 2 h is about 20.8, 21.2, 22.3, and 23.5 nm, respectively. summarizes the formation condition of single-phase La2MnCoO6 via various synthesis methods. We can see that the PVA sol-gel method in the present work has a lower temperature and shorter time for the synthesis of La2CoMnO6. To the best of our knowledge, this is the reported lowest synthesis temperature for La2CoMnO6.
Figure 3. XRD patterns of La2CoMnO6 precursors and powders calcined at various temperatures for 2 h via PVA sol-gel route
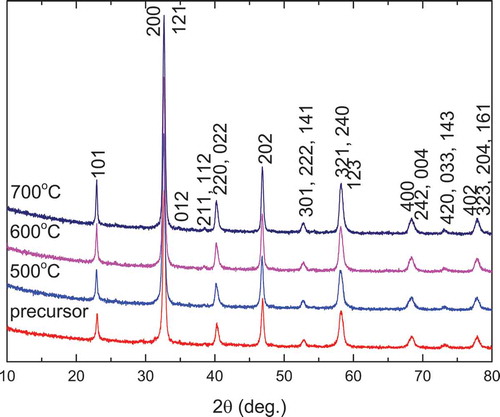
Table 1. The preparation method and formation conditions of single-phase La2CoMnO6.
The XRD patterns of Nd2CoMnO6 precursors and powders calcined at 500–700°C for 2 h are depicted in . The XRD pattern of the Nd2CoMnO6 precursor shows that it is amorphous, which is different from that of La2CoMnO6. La2CoMnO6 phase formed directly in the precursor, indicating the formation temperature of single-phase Nd2CoMnO6 is higher than that of La2CoMnO6, which can only be attributed to the smaller radius of Nd than that of La. The Nd2CoMnO6 powder calcined at 500°C is still amorphous although some very weak peaks from Nd2CoMnO6 appeared. Calcining the precursor at 600°C for 2 h, monophasic Nd2CoMnO6 with monoclinic structure was formed. Moreover, when the calcination temperature reaches to 700°C, the XRD patterns still remain almost unchanged. The XRD patterns are consistent with those reported in Ref. 24 and Citation36. Therefore, it is convinced that phase pure Nd2CoMnO6 can be prepared at 600°C for 2 h by PVA sol-gel method. The crystallite size of Nd2CoMnO6 powder calcined at 600°C and 700°C for 2 h calculated from Scherrer formula is about 20.1 and 23.9 nm, respectively. There are very few reports on the wet chemical synthesis of Nd2CoMnO6. Joly et al. prepared NdMn0.5Co0.5O3 using the glycine-nitrate combustion method. For the sample calcined at 700°C for 12 h, there may also be a small amount of hexagonal Nd2O3 impurity [Citation24]. We can conclude that the PVA sol-gel method can synthesize single-phase Nd2CoMnO6 at a lower temperature than the glycine-nitrate combustion method.
Figure 4. XRD patterns of Nd2CoMnO6 precursors and powders calcined at various temperatures for 2 h via PVA sol-gel route
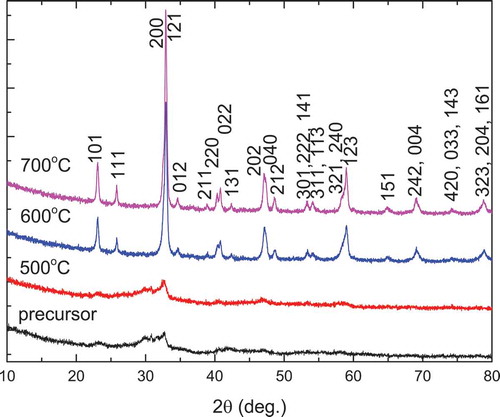
presents the FT-IR spectra of La2CoMnO6 precursor and calcined powders. For the La2CoMnO6 precursor, the presence of hydroxyl groups (O-H) is believed to give rise to a broad absorption band around 3450 cm−1. Moreover, two obvious absorption bands have been detected at 1384 cm−1 and 601 cm−1, which corresponds to nitrate ions [Citation35,Citation36] and the formation of La2CoMnO6 [Citation37], respectively. This is in accordance with the previous XRD result: La2CoMnO6 phase has formed in the precursor (see ). After heating the La2CoMnO6 precursor at 500–700°C, the intensities of absorption bands at 601 cm−1 increase due to increased crystallinity, and the band at 1384 cm−1 disappears because of the decomposition of residual nitrate.
The FT-IR spectra of Nd2CoMnO6 precursor and calcined powders are presented in . For the Nd2CoMnO6 precursor, the absorption bands around 3436 cm−1 and 1631 cm−1 are related to H2O molecules on the surface of the precursor; the absorption band around 1510 and 1385 cm−1 can be assigned to carbonate ions [Citation38,Citation39]. In the spectrum of Nd2CoMnO6 calcined at 500°C for 2 h, no obvious change was observed except decreased intensities of carbonate and adsorbed water. Heating the precursor at 600°C for 2 h, two new strong absorption bands at 589 cm−1 and 450 cm−1 appears, suggesting the formation of Nd2CoMnO6 [Citation37]. Similarly, this is also in accordance with the XRD results in . Compared with La2CoMnO6, the substitution of La by Nd resulted in a shift of the corresponding absorption band to a lower wave-number. The FT-IR spectrum for the powder calcined at 700°C is almost the same as that of the powder calcined at 600°C.
displays the Raman spectra of La2CoMnO6 precursor and powders calcined at 500°C and 700°C for 2 h. As can be seen from the figure, two Raman active modes around 515 cm−1 and 642 cm−1 are observed. The strongest Raman peak around 642 cm−1 can be attributed to the symmetric stretching of the basal oxygen ions of the octahedra (B1g) [Citation40]. The second Raman peak around 515 cm−1 can be attributed to the antisymmetric stretching (Ag) associated with the Jahn–Teller distortion [Citation41–43]. These Raman spectra are consistent with that reported in Refs. 10, and Citation44–46. In addition, the obtained Raman spectra shows an increase in the intensity of the peaks as the calcination temperature grows higher, which implies that the crystallinity of La2CoMnO6 increases.
Figure 7. Raman spectra of La2CoMnO6 precursors and powders calcined at 500°C and 700°C for 2 h via PVA sol-gel route
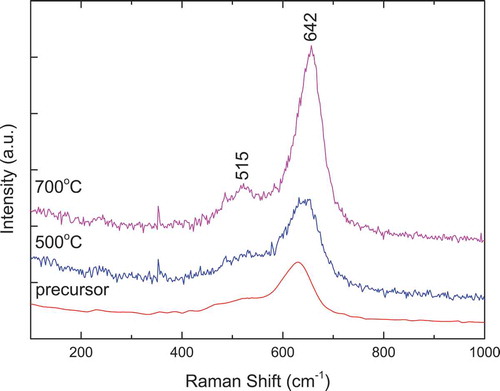
The Raman spectra of Nd2CoMnO6 precursors calcined at 500°C and 700°C are shown in . The Raman spectra are similar to that of La2CoMnO6 in , but the two Raman peaks move to lower wavenumber, which is consistent with that reported in Refs. Citation10 and Citation36. The peaks around 627 and 475 cm−1 belong to symmetric stretching and antisymmetric stretching/bending modes of the Co(Mn)O6 octahedron [Citation40–42]. Well-crystallized Nd2CoMnO6 formed at 700°C, its Raman spectrum is strong. Very weak crystallization peak appears in the XRD pattern of Nd2CoMnO6 precursors calcined at 500°C (), its Raman spectrum is obviously weaker than that of Nd2CoMnO6 precursors calcined at 700°C, but the two main Raman peaks have appeared.
Figure 8. Raman spectra of Nd2CoMnO6 precursors calcined at 500°C and 700°C for 2 h via PVA sol-gel route
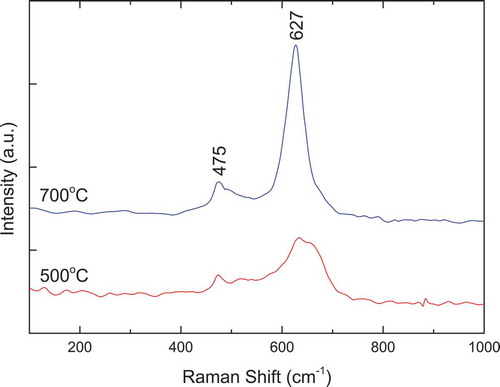
The FESEM images of La2CoMnO6 precursor, La2CoMnO6, and Nd2CoMnO6 powders calcined at 600°C for 2 h with different magnifications are presented in . La2CoMnO6 and Nd2CoMnO6 powders show three-dimensional network structures. This is because, during the formation of the gel, the long-chain structure of PVA is interlaced to form a complex network structure in the space. The gel network is filled with a solvent. When the gel is dried and calcined to form La2CoMnO6 or Nd2CoMnO6 powders, the powders also present a spatial three-dimensional network structure. The enlarged images ( (b,d,e)) revealed that the obtained powders are composed of smaller primary particles having a size on the order of nanometers.
Figure 9. FESEM micrographs of La2CoMnO6 precursor (a)(b), La2CoMnO6 powders (c)(d) and Nd2CoMnO6 powders (e)(f) calcined at 600°C for 2 h
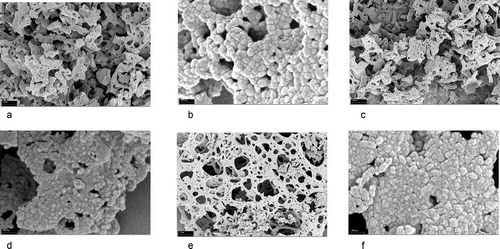
The magnetization vs. applied magnetic field curves at room temperature and 100 K for La2CoMnO6 precursor, La2CoMnO6 powder calcined at 500°C, and Nd2CoMnO6 powders calcined at 600°C are shown in , respectively. A linear relationship between magnetization vs. applied magnetic field at room temperature is observed for La2CoMnO6 precursor, La2CoMnO6, and Nd2CoMnO6 powders, indicating that the powders are paramagnetic at room temperature. However, La2CoMnO6 precursor, La2CoMnO6 and Nd2CoMnO6 powders show typical hysteresis loop at 100 K. This is consistent with the results reported in Refs. Citation7, Citation15, Citation27, and Citation36. Compared with La2CoMnO6 precursor, La2CoMnO6 powder calcined at 500°C shows larger remnant magnetization (MR) and coercive field (Hc). The magnetization curves of Nd2CoMnO6 powders do not show any saturation at 100 K even at the highest magnetic field of 20 kOe. In addition to that, Nd2CoMnO6 also exhibits a larger Hc of about 5 kOe at 100 K. Similar results are also reported in Nd2CoMnO6 prepared by solid-state reaction method and Sm2CoMnO6 [Citation7].
4. Conclusions
Pure La2CoMnO6 and Nd2CoMnO6 powders had been obtained through PVA sol-gel method. XRD analysis revealed that single phase La2CoMnO6 powders with an average crystallite size of ~20 nm was synthesized directly through the charring step, while monophasic and well-crystallized Nd2CoMnO6 powders were obtained at 600°C for 2 h. The PVA sol-gel method used in the procedure has the advantages of lower synthesis temperature and shorter preparation time for the synthesis of La2CoMnO6 and Nd2CoMnO6. Magnetic measurements showed that both La2CoMnO6 and Nd2CoMnO6 powders are paramagnetic at room temperature.
Acknowledgments
The authors wish to acknowledge the Analytical and Testing Center in Huazhong University of Science and Technology for XRD, FT-IR, and Raman, analysis.
Disclosure statement
No, potential conflict of interest was reported by the authors.
Additional information
Funding
References
- Kakarla DC, Jyothinagaram KM, Das AK, et al. Dielectric and magnetodielectric properties of R2NiMnO6 (R = Nd, Eu, Gd, Dy, and Y). J Am Ceram Soc. 2014;97:2858–2866.
- Singh MP, Truong KD, Fournier P, et al. A radical approach to promote multiferroic coupling in double perovskites. J Magn Magn Mater. 2009;321:1743–1747.
- Balli M, Fournier P, Jandl S, et al. A study of the phase transition and magnetocaloric effect in multiferroic La2MnNiO6 single crystals. J Appl Phys. 2014;115:173904.
- Murthy JK, Chandrasekhar KD, Murugavel S, et al. Investigation of the intrinsic magnetodielectric effect in La2CoMnO6: role of magnetic disorder. J Mater Chem C. 2015;3:836–843.
- Wang QQ, Ma LP, Wang LC, et al. Mechanisms for enhanced catalytic performance for NO oxidation over La2CoMnO6 double perovskite by A-site or B-site doping: effects of the B-site ionic magnetic moments. Chem Eng J. 2019;372:728–741.
- Dass RI, Goodenough JB. Multiple magnetic phases of La2CoMnO6-δ (0≤δ≤0.05). Phys Rev B. 2003;67:014401.
- Sahoo RC, Das S, Nath TK. Effect of rare earth site substitution on magnetic and transport properties of Ln2CoMnO6 (Ln = La, Sm and Gd) double perovskites. J Magn Magn Mater. 2018;460:409–417.
- Troyanchuk IO, Samsonenko NV, Shapovalova EF, et al. A synthesis and characterization of Ln(B0.5Mn0.5)O3 (Ln-lanthanoid; B = Ni, Co) perovskites. Mater Res Bull. 1997;32:67–74.
- Asai K, Fujiyoshi K, Nishimori N, et al. Magnetic properties of REMe0.5Mn0.5O3 (RE = Rare Earth Element, Me = Ni, Co). J Phys Soc Jpn. 1998;67:4218–4228.
- Bull CL, McMillan PF. Raman scattering study and electrical properties characterization of elpasolite perovskites Ln2(BB′)O6 (Ln=La, Sm … Gd and B, B’=Ni, Co, Mn). J Solid State Chem. 2004;177:2323–2328.
- Sazonov AP, Troyanchuk IO, Kozlenko DP, et al. Magnetic ordering in the Nd2CoMnO6+δ perovskite system. J Magn Magn Mater. 2006;302:443–447.
- Madhogaria RP, Das R, Clements EM, et al. Effect of antiphase boundaries on the magnetic properties of La2CoMnO6. AIP Adv. 2019;9:035142.
- Goodenough JB, Wold A, Arnott RJ, et al. Relationship between crystal symmetry and magnetic properties of ionic compounds containing Mn3+. Phys Rev. 1961;124:373–384.
- Sazonov AP, Troyanchuk IO, Sikolenko VV, et al. Effect of the oxygen nonstoichiometry on the structure and magnetic properties of Nd2CoMnO6+δ double perovskites. Phys Stat Sol b. 2007;244:3367–3376.
- Mahato RN, Sethupathi K, Sankaranarayanan V. Colossal magnetoresistance in the double perovskite oxide La2CoMnO6. J Appl Phys. 2010;107:09D714.
- Jia YS, Li Y, Zhao LZ, et al. Magnetism and critical behavior of double perovskite RE2CoMnO6 (RE = La and Eu) compounds. J Magn Magn Mater. 2019;481:156–161.
- Chang H, Gao Y, Liu F, et al. Effect of synthesis on structure, oxygen voids, valance bands, forbidden band gap and magnetic domain configuration of La2CoMnO6. J Alloy Compd. 2017;690:8–14.
- Hosseini SA, Salari D, Niaei A, et al. Physical–chemical property and activity evaluation of LaB0.5Co0.5O3 (B = Cr, Mn, Cu) and LaMnxCo1-xO3 (x = 01, 025, 05) nano perovskites in VOC combustion. J Ind Eng Chem. 2013;19:1903–1909.
- Jiang M, Li J, Zhao Y, et al. Double perovskites as model bifunctional catalysts toward rational design: the correlation between electrocatalytic activity and complex spin configuration. ACS Appl Mater Interfaces. 2018;10:19746–19754.
- Murthy JK, Venimadhav A. Magnetodielectric behavior in La2CoMnO6 nanoparticles. J Appl Phys. 2012;111:024102.
- Liu F, Gao Y, Chang H, et al. Control of magnetic properties and band gap by Co/Mn ordering and oxygen distributions of La2CoMnO6. J Magn Magn Mater. 2017;435:217–222.
- Silva RX, De MAS, Almeida RM, et al. Structural order, magnetic and intrinsic dielectric properties of magnetoelectric La2CoMnO6. J Alloy Compd. 2016;661:541–552.
- Joy PA, Khollam YB, Patole SN, et al. Low-temperature synthesis of single phase LaMn0.5Co0.5O3. Mater Lett. 2000;46:261–264.
- Joly VLJ, Joy PA, Date SK. Synthesis of two different ferromagnetic phases of RMn0.5Co0.5O3 (R = Pr, Nd, Sm) by a low-temperature method. Mater Lett. 2001;51:172–175.
- Viswanathan M, Kumar PSA, Bhadram VS, et al. Influence of lattice distortion on the Curie temperature and spin–phonon coupling in LaMn0.5Co0.5O3. J Phys Condens Matter. 2010;22:346006.
- Sayed FN, Achary SN, Deshpande SK, et al. Role of annealing atmosphere on structure, dielectric and magnetic properties of La2CoMnO6 and La2MgMnO6. Z Anorg Allg Chem. 2014;640:1907–1921.
- Filho PLC, Barrozo P, Landinez-Tellez DA, et al. Structural and magnetic properties of Ln2CoMnO6 (Ln=Dy and La) produced by combustion synthesis. J Supercond Nov Magn. 2013;26:2521–2524.
- Bull CL, Gleeson D, Knight KS. Determination of B-site ordering and structural transformations in the mixed transition metal perovskites La2CoMnO6 and La2NiMnO6. J Phys Condens Matter. 2003;15:4927–4936.
- Nishimori N, Asai K, Mizoguchi M. NMR Study on the super transferred hyperfine magnetic field at 55Mn in ferromagnetic perovskites La(Co1-xMgx)0.5Mn0.5O3. J Phys Soc Jpn. 1995;64:1326–1333.
- Mahendiran R, Bréard Y, Hervieu M, et al. Giant frequency dependence of dynamic freezing in nanocrystalline ferromagnetic LaCo0.5Mn0.5O3. Phys Rev B. 2003;68:104402.
- Mao Y. Facile molten-salt synthesis of double perovskite La2BMnO6 nanoparticles. RSC Adv. 2012;2:12675–12678.
- Mao Y, Parsons J, McCloy JS. Magnetic properties of double perovskite La2BMnO6 (B = Ni or Co) nanoparticles. Nanoscale. 2013;5:4720–4728.
- Saha SK, Pathak S, Pramanik P. Low-temperature preparation of fine particles of mixed oxide systems. J Mater Sci Lett. 1995;14:35–37.
- Gulgun MA, Nguyen MH, Kriven WM. Polymerized organic-inorganic synthesis of mixed oxides. J Am Ceram Soc. 1999;82:556–560.
- Liu T, Xu YB, Zhao JY. Low-temperature synthesis of BiFeO3 via PVA sol-gel route. J Am Ceram Soc. 2010;93:3637–3641.
- Ghosh S, Dasgupta S, Sen A, et al. Low-temperature synthesis of nanosized bismuth ferrite by soft chemical route. J Am Ceram Soc. 2005;88:1349–1352.
- Rao GVS, Rao CNR, Ferraro JR. Infrared and electronic spectra of rare earth perovskites: ortho-chromites, -manganites and –ferrites. Appl Spectrosc. 1970;24:436–445.
- Busca G, Lorenzelli V. Infrared spectroscopic identification of species arising from reactive adsorption of carbon oxides on metal oxide surfaces. Mater Chem. 1982;7:89–126.
- Feng JS, Liu T, Xu YB, et al. Effects of PVA content on the synthesis of LaFeO3 via sol–gel route. Ceram Int. 2011;37:1203–1207.
- Martín-Carrón L, de Andrés A, MJ M-L, et al. Raman phonons as a probe of disorder, fluctuations, and local structure in doped and undoped orthorhombic and rhombohedral manganites. Phys Rev B. 2002;66:174303.
- Martín-Carrón L, de Andrés A, MT C, et al. Raman phonons and light scattering in RMnO3 (R=La, Pr, Nd, Ho, Er Tb and Y) orthorhombic and hexagonal manganites. J Alloy Compd. 2001;323–324: 494–497.
- Martín-Carrón L, de Andrés A. Melting of the cooperative Jahn-Teller distortion in LaMnO3 single crystal studied by Raman spectroscopy. Eur Phys J B. 2001;22:11–16.
- Truong KD, Laverdiére J, Singh MP, et al. Impact of Co/Mn cation ordering on phonon anomalies in La2CoMnO6 double perovskites: raman spectroscopy. Phys Rev B. 2007;76:132413.
- Iliev MN, Abrashev MV, Litvinchuk AP, et al. Raman spectroscopy of ordered double perovskite La2CoMnO6 thin films. Phys Rev B. 2007;75:104118.
- Yadav R, Para TA, Reshi HA, et al. Easy synthesis and electric, magneto-transport and magnetic properties of double perovskite La2CoMnO6 compound. J Mater Sci: Mater Electron. 2017;28:2970–2975.
- Das RR, Lekshmi PN, Das SC, et al. Competing short-range magnetic correlations, metamagnetic behavior and spin-phonon coupling in Nd2CoMnO6 double perovskite. J Alloy Compd. 2019;773:770–777.