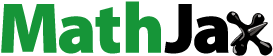
ABSTRACT
Cobalt blue pigments, which mainly comprise CoAl2O4, are widely synthesized as nano-scale particles using the coprecipitation method. Such ultrafine powders are necessary for the ceramics industry because of their significant effect on the color of glaze and bulk tiles. In this paper, cobalt blue pigments have been prepared using the coprecipitation method and then subjected to supercritical fluid drying. The differences in their physicochemical and optical properties are characterized using a series of analysis including X-ray diffraction (XRD), field emission scanning electron microscopy (FESEM), energy-dispersive spectrometer (EDS), and CIE-L*a*b*colorimetry analysis. The results indicate that the CoAl2O4 pigments subjected to supercritical fluid drying exhibit a fine-scale structure, a crystallite size of approximately 52 nm at 1400°C, and an excellent stability at high temperatures. They also present a vivid blue color, with improved brightness and hue than those of other cobalt blue pigments. Furthermore, this study elucidates the promising potential of supercritical fluid drying for fabricating nano-sized CoAl2O4 pigments with excellent optical and physicochemical properties.
1. Introduction
Inorganic ceramic pigments with nano-sized particles are known to have great market potential as they can not only protect and decorate ceramic products but also improve the stability of traditional pigments. To achieve a tiny granularity, nano-sized pigment particles are used to attain a high surface area, which leads to high surface coverage and a number of reflectance points [Citation1]. In recent years, ink-jet technology has become increasingly popular; however, nozzle clogging is a severe problem [Citation2,Citation3]. Therefore, the size of particles and their agglomeration must be controlled for ink-jet printing. Consequently, smaller inorganic particles that exhibit less agglomeration have been prepared by numerous researchers [Citation4]. Moreover, pigments with thinner particles also contribute to improving the optical property of ceramics.
It is well established that the type of cobalt ion determines the blue color of the ceramic [Citation5]. In the recent past, blue CoAl2O4 has usually been synthesized using a co-precipitation method [Citation5–7], solution combustion [Citation8,Citation9], grinding and calcining, molten salt decomposition, sol-gel method [Citation10], and hydrothermal method [Citation11]. The products prepared using the coprecipitation method exhibit the advantages of excellent uniformity, high purity, minimal side reactions, an easily controlled reaction process, and a potential for industrialization. Meanwhile, solution combustion is the traditional drying method. Furthermore, CoAl2O4 is a cubic spinel structure wherein aluminum and cobalt are located in octahedral and tetrahedral sites, respectively [Citation12]. CoAl2O4 pigments with high-temperature stability are widely used for underglaze ceramic decoration, since the treatment temperature is often required to be 1360°C or above.
The supercritical drying approach, which increases the pressure and temperature of a substance beyond its critical values, has proven to be useful for reducing the agglomeration of particles [Citation13]. Under such conditions, the substance (often gas) becomes similar to an ordinary liquid; that is, it exhibits comparable density, fluidity, and some other characteristics. Furthermore, surface tension and capillary forces are eliminated; therefore, if the solvent liquid is pulled away in this state, superfine particles are obtained. This property has been effectively utilized in the fluid drying process.
In this article, coprecipitation method has been used with supercritical ethanol to prepare a nanoscale underglaze ceramic pigment that is blue. Simultaneously, another control group is prepared by employing traditional heat drying before calcination. The impacts of supercritical state on the particle size, structure, and color properties of the ceramic pigments were tested and analyzed. Compared with the control group subjected to traditional heat drying, the advantages of the prepared nanoscale samples have been elucidated in terms of coloration, structure, and size.
2. Experimental procedure
Cobalt acetate (C4H6CoO4 · 4H2O, Aladdin Corp.) and aluminum nitrate (Al(NO3)3 · 9H2O, Aladdin Corp.) are dissolved separately in water with a molar ratio of 1:2; subsequently, they are blended together. Under magnetic stirring at 500 rpm, NH3·H2O was added dropwise to reach a pH value of approximately 9; here, the NH3·H2O quantity can marginally exceed the required value. Stirring was performed for 2.5 h to ensure full precipitation, and the precipitate was separated with a centrifugal machine; subsequently, the precipitate was washed with absolute ethanol to neutrality for obtaining an alcohol mixture comprising cobalt and aluminum hydroxide. Next, the stainless-steel cylinder containing the alcohol mixture was placed in the supercritical device; subsequently, anhydrous ethanol was also added to this device, after which it was sealed and heated. The fluid pressure was set at 8.5 MPa and the temperature reached up to 250°C. These pressure and temperature values were maintained for 120 min; the fluid was slowly discharged after being cooled to room temperature, thereby forming a loose CoAl2O4 powder. Consequently, some amount of the as-prepared powders were separately heated at 500°C, 800°C, 1200°C, and 1400°C for 1 h. Another group of samples was prepared using the same protocol, expect for the fact that they were subjected to heat drying.
The as-prepared powders were characterized using phase analysis (XRD, D/MAX2500VL/PC, Rigaku, Japan), field emission scanning electronic microscopy (FESEM, MIRA3 LMU, TESCAN.), energy-dispersive spectrometer (EDS), colorimetry analysis with a spectrophotometer (FD-5, SHIMADZU), and infrared spectrometer (Thermo, Madison, USA). In the XRD analysis, the full width with a half maximum (FWHM) values were defined by referring to standard silicon powder. Scherrer equation was used with peak widths to evaluate the crystallite sizes, according to Equationequation (1)(1)
(1) [Citation14]:
Where D is the average thickness of the crystal grain that is perpendicular to the crystal plane, K is the Scherrer constant, γ is the X-ray wavelength (generally 1.54056 Å), B is the half-height width or integral width of the measured sample diffraction peak, and θ is the Bragg angle in degrees.
3. Results and discussion
3.1. X-ray diffraction
The X-ray diffraction patterns of precursors dried at different supercritical temperatures are shown in , and XRD patterns for powders with conventional heat drying are presented in Figure S1. Synthesized CoAl2O4 pigment powders are observed to be made up of spinel phases. According to the JCPDS-ICDD cards [Citation15], the main crystal phase of powders treated at 500°C is Co3O4, while main crystal phases of powders heated at 800°C are Co3O4 and CoAl2O4. At 1200°C, CoAl2O4 is mixed with Co2AlO4 and occupies the main phase; it transforms into CoAl2O4 as the temperature rises to 1400°C. Diffraction peaks of (220), (331), and (440) corresponding to CoAl2O4 become more evident as the temperature increases. When comparing the set of four patterns, it is not difficult to determine that higher temperatures are used to treat the powders; consequently, the purer spinel phase can be obtained. In addition, the products treated at different temperatures exhibit only a few differences, which indicates that no new phases are obtained. Based on these results, it can be established that phase stability is not influenced by heat treatment.
The structure parameters of powders subjected to supercritical fluid drying and traditional heat drying at different temperatures are shown in . The intensity ratios of powders (I(311)/I(220)) subjected to supercritical fluid drying are higher than those treated using traditional heat drying, regardless of the heating temperature. It has been reported previously that the low I(311)/I(220) value can be attributed to a low level of cation mixing [Citation16,Citation17]. A (331) peak is observed at 1200°C and 1400°C, which is associated with the CoAl2O4 phase according to JCPDS-ICDD cards (No. 10–458) [Citation15]. Further, an increase in the (331) peak intensity indicates the exaltation of CoAl2O4. The FWHM of (311) diffraction peaks decreases with heating, which also indicates an increase in particle size and an improvement in crystallinity.
Table 1. Structural parameters of as-prepared powders heated at different temperatures
The crystallite sizes are calculated by using the Scherrer equation [Citation14]. The crystallites become larger as the temperature rises, regardless of whether the precursors are treated using the supercritical method or traditional heating. Further, the size of the powders subjected to supercritical drying is smaller than that of the samples treated with heat drying at the corresponding temperatures. Besides, when the temperature increases from 1200°C to 1400°C, the samples become larger; the increase in size is the maximum at this temperature range. This result implies that the formation of CoAl2O4 could enlarge the crystallite. When compared with the pigments subjected to heat drying, pigment particles dried using the supercritical fluid show a size of 51.71 nm at 1400°C and 36.27 nm at 1200°C; these diameters are significantly smaller than those of the particles subjected to traditional heating drying (72.59 nm and 50.07 nm, respectively). Therefore, supercritical drying can increase the crystallinity, decrease the crystallite size, and alleviate the agglomeration of the particles, thereby exhibiting potential for application in the field of nano-scale materials.
3.2. Field emission scanning electronic microscopy
FESEM analysis of the CoAl2O4 powders synthesized using the coprecipitation method and subjected to supercritical drying and traditional heat drying is shown in . It is observed that both powders are uniformly sized. demonstrate that the pigments subjected to traditional heat drying show a uniform square morphology with narrow size distribution, and that the particle size is smaller than 500 nm. The morphology of powders can be clearly observed, implying that no agglomeration occurred. show a smaller spinel size than that observed in , which is in accordance with the particle size distribution shown in . This is probably because the supercritical method removes or reduces the surface tension effect caused by the agglomeration of colloid particles; therefore, the powder becomes dispersible. The process of supercritical drying is shown in . Under high pressure, the supercritical fluid (ethanol) penetrates the boundaries of the particles, mixes with the particles, dopes the holes on the particles, and helps Co(OH)2 and Al(OH)3 spread evenly, which is helpful for the fabrication of CoAl2O4. When the pressure is relieved and the supercritical fluid is released, the ethanol is liberated from the intervals of the particles; if the residual solvent is removed, the agglomeration will break. As compared to traditional heat drying methods performed at atmospheric pressure, the framework of the precipitate can easily collapse with a weak cohesion during the drying process; therefore, the precipitates are not easily agglomerated and finer particles can be obtained.
Figure 2. CoAl2O4 powders subjected to traditional heat drying ((a) and (b)) and supercritical fluid drying ((c) and (d)) at 1400°C.
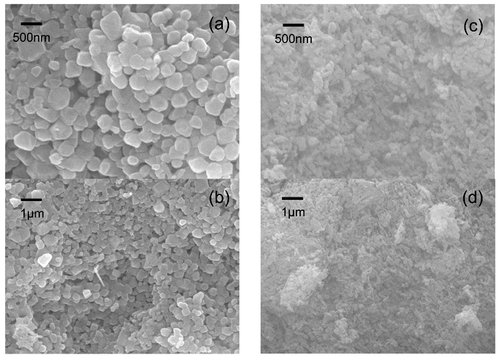
Figure 3. Particle size distribution of CoAl2O4 powders subjected to traditional heat drying (b) and supercritical fluid drying (d) at 1400°C.
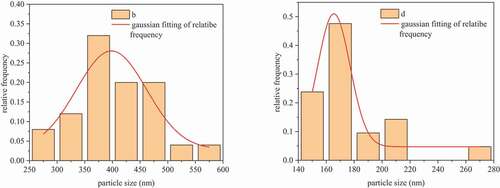
Figure 4. Stages of the supercritical fluid drying process: (a) applying pressure and (b) releasing pressure.
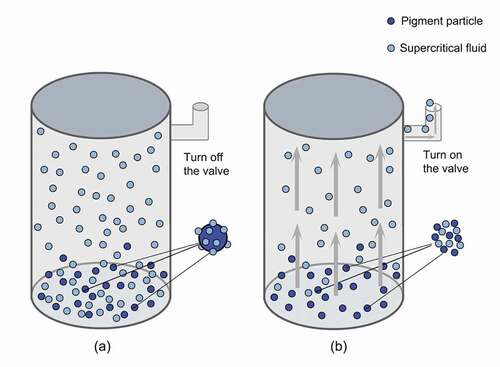
An energy-dispersive spectrometer (EDS) has been utilized to confirm the composition of the powders after heating. Based on the quantitative analysis of the spectrum (), the Co/Al weight ratio is 1.24 and the corresponding atomic ratio is 0.57, which probably proves that CoAl2O4 is the main phase.
Table 2. Composition parameters of the CoAl2O4 sample heated at 1400°C via the supercritical fluid drying method
3.3. Infrared spectroscopy
The IR spectra of the CoAl2O4 sample dried with the supercritical method at 500°C, 1200°C, and 1400°C are recorded in the 4000–400 cm−1 range (). Three bands near ~500 cm−1, ~540 cm−1, and ~660 cm−1 are observed to exhibit a relationship with the formation of blue CoAl2O4 [Citation18]. The bands at 680.59 and 564.04 cm−1 are related to the inherent stretching vibrations associated with metal ion doping in tetrahedral sites, which correspond to Co-O in CoAl2O4. Likewise, the band at 503.30 cm−1 is attributed to the octahedral metal stretching frequency of Al-O [Citation19,Citation20]. Positions of the main frequency bands depend on the configurations of ions in tetrahedral and octahedral sites. The shifts from 500°C to 1200°C and 1400°C show the phase transformation to CoAl2O4 [Citation21].
3.4. Colorimetry analysis
As reported in previous research, CoAl2O4 is expected to be a spinel-structural crystal, in which the Co2+ ions occupy the tetrahedral sites and Al3+ ions occupy the octahedral sites. Co2+ ions in tetrahedral sites render the crystallite blue; however, if Co2+ ions dope the octahedral sites, the pigments will turn green [Citation18,Citation22,Citation23].
The colorimetry analysis of the samples treated with supercritical drying and traditional heat drying has been presented in by using L*, a*, and b* values. To illustrate the differences intuitively and conveniently, the related colors are transferred to RGB values and filled in the small blocks. The L* value (0 to 100) mainly corresponds to the brightness and intensity of the pigments (a higher L* value indicates a brighter color, while a lower L* indicates a darker color or lower intensity). The parameter a* lies between −127 to 128, which indicates the color range from green to red. Meanwhile, the blue hue is primarily controlled by the parameter b*, which lies between −128 to 127 and indicates the color range from blue to yellow [Citation24]. The L*a*b* parameters were transformed to RGB color space for clearly showing the color descriptions ().
Table 3. CIEL*a*b* colorimetry of CoAl2O4 pigment powders subjected to varying drying treatments at different temperatures and time
According to the results observed in , a higher blue hue and brighter color are exhibited by the cobalt blue pigments dried using the supercritical method, as indicated by the relatively high L* value and low b* value; this result is in contrast to that obtained for the samples subjected to traditional heat drying at 1200°C and 1400°C. However, both sets of samples show small a* values, thereby giving rise to the green color. Based on the results and discussion of the XRD analysis, Co2AlO4 mixes with the CoAl2O4 phase in the samples heated at 1200°C, which leads to the green color being observed in the colorimetry analysis. For samples treated at 1400°C, both set of samples present higher levels of blue hues. A decrease in the green hue and an increase in the blue hue imply that the partial crystal phases transform from Co2AlO4 to CoAl2O4; this result is the same as that observed in the XRD analysis above.
For all the samples that have been subjected to increasing temperatures, the precursors are mainly converted to Co3O4 at 500°C and 800°C and then transformed into CoAl2O4 at higher temperatures. Co2AlO4 can be synthesized using the side reaction that occurs at the temperature range from 800°C to 1200°C. The colorimetry analysis is consistent with the results of XRD analysis. Overall, the pigments treated with supercritical drying demonstrate brighter colors with bluer hues, as compared to those of the control group.
4. Conclusions
Herein, cobalt blue underglaze ceramic pigments (CoAl2O4) are synthesized using the coprecipitation method and subjected to either supercritical fluid drying or traditional heat drying. The differences in the characteristic of both sample groups are investigated, including their physicochemical and optical properties.
Pigments dried with the supercritical fluid are indicated to possess higher crystallinity, finer crystallite size, and superior stability at different temperatures. As the heating temperature increases from 1200°C to 1400°C, the pigment particles agglomerate and the crystallite size increases, which could be attributed to the phase transformation to CoAl2O4. At 1400°C, the relatively stable pigment particles obtained via the supercritical method present a smaller size (51.71 nm) than that of the samples treated with traditional heat drying (72.59 nm). The SEM results also confirm the potential of the supercritical method for preparing nano-scale pigment particles. Furthermore, the supercritical method can facilitate pigment coloration. In general, the cobalt blue pigments dried using the supercritical fluid show high L* values and low b* values, which correspond to high brightness and blue hues, respectively.
Acknowledgments
The authors gratefully acknowledge the supported from the Key Projects of Hunan Provincial Department of Education (19A132, 18C0492), the Natural Science Foundation of Hunan Province (2020JJ6070), and the National College Student Innovation Training Program (S201911535010, S20201153505S), The science and technology innovation Programof Hunan Province (2021RC4065).
Disclosure statement
No potential conflict of interest was reported by the author(s).
Additional information
Funding
References
- Baldi G, Baldi G, Baldi G, et al., P.M.T. Cavalcante. Colour performance of ceramic nano-pigments. Dyes Pigm. 2009;80(2):226–232.
- Chen ZZ, Shi EW, Li WJ, et al. hydrothermal synthesis and optical property of nano-sized CoAl2O4 pigment. Mater Lett. 2002;55(5):281–284.
- Chang QB, Wang X, Wang YQ, et al. Particle size and chroma control of CoAl2O4 pigment. Key Eng Mater. 2015;655:220–223.
- Kim JH, Son BR, Yoon DH, et al. Characterization of blue CoAl2O4 nano-pigment synthesized by ultrasonic hydrothermal method. Ceram Int. 2012;38(7):5707–5712.
- Ahmed IS, Shama SA, Moustafa MM, et al. Synthesis and spectral characterization of CoxMg1-xAl2O4 as new nano-coloring agent of ceramic pigment. Spectrochim Acta A. 2009;74(3):665–672.
- Song Y, Zheng Y, Tang Y, et al. Fabrication and coloration mechanism of CoAl2O4 ceramic pigment by co-precipitation method. J Synth Cryst. 2017;46:79–84. CNKI:SUN:RGJThttps://doi.org/https://doi.org/10.2017/01.014.
- Zhang A, Mu B, Luo Z, et al. Bright blue halloysite/CoAl2O4 hybrid pigments: preparation, characterization and application in water-based painting. Dyes Pigm. 2017;139:473–481.
- Liu X, Li J, Cheng K. preparation o f spinel type ZnxCo1-xAI2O4 ultrafine ceramic pigment by solution combustion method. J Synth Cryst. 2014;43:2086–2090. CNKI:SUN:RGJThttps://doi.org/https://doi.org/10.2014/08.053.
- Gilabert J, Palacios MD, Sanz V, et al. Characteristics reproducibility of (Fe, Co)(Cr, Al)2O4 pigments obtained by solution combustion synthesis. Ceram Int. 2016;42(11):12880–12887.
- Chemlal S, Larbot A, Persin M, et al. Cobalt spinel CoAl2O4 via sol-gel process: elaboration and surface properties. Mater Res Bull. 2000;35(14–15):2515–2523.
- Bai LX. Synthesis of cobalt–aluminate spinels via glycine chelated precursors. Mater Chem Phys. 2006. DOI:https://doi.org/10.1016/j.matchemphys.2005.07.066
- Agilandeswari K, Kumar AR. Synthesis, characterisation, optical and luminescence properties of CoAl2O4. AIP Publishing LLC. 2015;1667:120022.
- Roth TB, Anderson AM, Carroll MK. Analysis of a rapid supercritical extraction aerogel fabrication process: prediction of thermodynamic conditions during processing. J Non Cryst Solids. 2008;354(31):3685–3693.
- Holzwarth U, Gibson N. The Scherrer equation versus the ‘Debye-Scherrer equation’. Nat Nanotechnol. 2011;6(9):534.
- Wong-Ng W, Mcmurdie HF, Hubbard CR, et al. JCPDS-ICDD Research Associateship (cooperative program with NBS/NIST). J Res Natl Inst Stan. 2001;106(6):1013.
- Arjunan P, Kouthaman M, Kannan K, et al. Stable prismatic layer structured cathode material via Cation mixing for sodium ion battery. Ionics. 2020;26(9):4543–4551.
- Alade I-O, Zhang Y, Xu X. Modeling and prediction of lattice parameters of binary spinel compounds (AM2X4) using support vector regression with Bayesian optimization. New J Chem. 2021;45(34):15255–15266.
- Mindru I, Marinescu G, Gingasu D, et al. Blue CoAl2O4 spinel via complexation method. Mater Chem Phys. 2010;122(2–3):491–497.
- Waldron RD. Infrared spectra of ferrites. Phys Rev. 1955;99(6):1727–1735.
- Peng X, Cheng J, Yuana J, et al. Environmental blue CoAl2O4 pigment co-doped by Zn2+ and Mg2+: synthesis, structure and optical properties. Adv Appl Ceram. 2018;117(5):303–311.
- Ahmad SI, Ansari SA, Kumar DR. Structural, morphological, magnetic properties and cation distribution of Ce and Sm co-substituted nano crystalline cobalt ferrite. Mater Chem Phys. 2018;208:248–257.
- Chandramohan P, Srinivasan MP, Velmurugan S, et al. Cation distribution and particle size effect on Raman spectrum of CoFe2O4. J Solid State Chem. 2011;184(1):89–96.
- Gaudon M, Robertson LC, Lataste E, et al. A. demourgues, cobalt and nickel aluminate spinels: blue and cyan pigments. Ceram Int. 2014;40(4):5201–5207.
- Luo MR, Cui G, Rigg B. The development of the CIE 2000 colour-difference formula: CIEDE2000. Color Res Appl. 2001;26(5):340–350.