ABSTRACT
Transition metals are nutrients essential for life. However, an excess of metals can be toxic to cells, and host-imposed metal toxicity is an important mechanism for controlling bacterial infection. Accordingly, bacteria have evolved metal efflux systems to maintain metal homeostasis. Here, we established that PmtA functions as a ferrous iron [Fe(II)] and cobalt [Co(II)] efflux pump in Streptococcus suis, an emerging zoonotic pathogen responsible for severe infections in both humans and pigs. pmtA expression is induced by Fe(II), Co(II), and nickel [Ni(II)], whereas PmtA protects S. suis against Fe(II) and ferric iron [Fe(III)]-induced bactericidal effect, as well as Co(II) and zinc [Zn(II)]-induced bacteriostatic effect. In the presence of elevated concentrations of Fe(II) and Co(II), ΔpmtA accumulates high levels of intracellular iron and cobalt, respectively. ΔpmtA is also more sensitive to streptonigrin, a Fe(II)-activated antibiotic. Furthermore, growth defects of ΔpmtA under Fe(II) or Co(II) excess conditions can be alleviated by manganese [Mn(II)] supplementation. Finally, PmtA plays a role in tolerance to H2O2-induced oxidative stress, yet is not involved in the virulence of S. suis in mice. Together, these data demonstrate that S. suis PmtA acts as a Fe(II) and Co(II) efflux pump, and contributes to oxidative stress resistance.
Introduction
Transition metals such as iron, manganese, zinc, and copper are nutrients essential for life, and a variety of enzymes require a metal cofactor for their catalytic activity [Citation1,Citation2]. Decreasing the availability of these metals plays an important role in the strategy used by the host immune system to limit bacterial growth [Citation3]. As a countermeasure, bacteria employ various mechanisms to acquire metals from diverse sources [Citation1]. While acquiring metals is crucial for bacterial survival, an excessive accumulation of these metals can be toxic to cells [Citation4]. Emerging evidence indicates that imposed metal toxicity is another host defense strategy against bacterial pathogens [Citation1]. For example, neutrophils can mobilize zinc in response to Streptococcus pyogenes infection [Citation5]. Consequently, bacteria have evolved complex systems to maintain metal homeostasis, such as efflux or sequestration of metals [Citation6]. Moreover, there is mounting evidence that metal homeostasis, maintained via metal efflux pumps, plays a critical role in bacterial physiology and pathogenesis [Citation5–11].
Streptococcus suis is an important swine pathogen, causing meningitis, septicemia, pneumonia, endocarditis, and arthritis [Citation12]. It is also an emerging zoonotic agent, which has been associated with meningitis, septicemia, and other infections in humans [Citation12–14]. Currently, 29 serotypes have been proposed for S. suis [Citation15]. Among them, S. suis serotype 2 (S. suis 2) is the most frequently isolated worldwide and the most commonly involved in disease in both pigs and humans [Citation12]. By 2013, S. suis had resulted in more than 1600 human cases of infection worldwide, with the majority reported in Asia [Citation14]. Of note, a total of 240 human cases with 53 deaths were recorded in China during two large outbreaks of S. suis 2 infection, which occurred in 1998 and 2005 [Citation16,Citation17]. In recent years, sporadic human cases of S. suis infection have been frequently reported worldwide [Citation18–22], indicating that S. suis is a persistent threat to public health.
To date, the metal efflux systems of S. suis have received little attention. Indeed, a single cation efflux family protein, MntE, has been identified as a manganese [Mn(II)] export system and is involved in S. suis virulence [Citation23]. Recently, a PerR-regulated P1B-4-type ATPase (PmtA) has been reported to act as a ferrous iron [Fe(II)] efflux pump and to contribute to oxidative stress resistance and virulence in S. pyogenes [Citation10,Citation11]. Moreover, PfeT, the PmtA homolog of Bacillus subtilis, is known to protect bacteria from iron intoxication [Citation7], while FrvA, the PmtA homolog of Listeria monocytogenes, is critical for its virulence [Citation8]. These findings indicate that in certain bacteria, Fe(II) efflux and homeostasis are important for bacterial survival and pathogenesis. In S. suis, iron sequestration by Dpr plays a central role in intracellular iron homeostasis [Citation24,Citation25]. However, whether an Fe(II) efflux mechanism exists in S. suis remains unclear.
In this study, we focused on the PmtA homolog of S. suis and confirmed its role in Fe(II) and cobalt [Co(II)] efflux. We also investigated the role of S. suis PmtA in oxidative stress resistance and virulence. Our findings revealed that PmtA plays a role in oxidative stress resistance, yet has no effect on S. suis virulence.
Materials and methods
Bacterial strains, plasmids, primers, and culture conditions
Bacterial strains and plasmids used in this study are listed in Table S1. Primers are listed in Table S2. S. suis 2 strain SC19 [Citation26] and its isogenic derivatives were routinely cultured at 37°C in Tryptic Soy Broth (TSB) or on Tryptic Soy Agar (TSA; Becton, Dickinson and Company) supplemented with 10% (vol/vol) newborn bovine serum. Escherichia coli strain DH5α was grown in Luria–Bertani (LB) broth or on LB agar. When required, spectinomycin was added at 50 and 100 μg/ml for E. coli and S. suis, respectively.
Quantitative gene expression analysis
The SC19 strain was grown to mid-exponential phase (OD600 =0.6) and divided into eight aliquots, seven of which were treated for 15 min with 1 mM FeSO4, 1 mM Fe(NO3)3, 0.25 mM CoSO4, 1 mM MnSO4, 1 mM NiSO4, 0.5 mM CuSO4, or 0.1 mM ZnSO4, respectively. The remaining aliquot was supplemented with deionized water (H2O) and served as the control. For each sample, total RNA was extracted using the Eastep Super total RNA isolation kit (Promega). RNA (500 ng) was converted to cDNA using the PrimeScript RT reagent Kit (TaKaRa). The MIQE guidelines [Citation27] were followed for quantitative PCR (qPCR) analysis. qPCR was performed on a StepOnePlus Real-Time PCR System (Applied Biosystems) using TB Green Premix Ex Taq II (TaKaRa). Melting curve analysis was performed to detect the specificity of the products. The reaction efficiency was evaluated by using serially diluted cDNA as the template. The relative gene expression level was assessed using the 2-ΔΔCT method [Citation28] with 16S rRNA as the reference gene.
Construction of a pmtA deletion mutant and functional complementation
Allelic exchange using a pSET4s suicide vector [Citation29] was performed to generate a pmtA deletion mutant (ΔpmtA) in the SC19 background. The complementation strain was constructed using the pSET2 vector, as described previously [Citation30].
Growth curve analyses
Metal toxicity assay. The wild-type (WT), ΔpmtA, and CΔpmtA strains grown to exponential phase were diluted in fresh medium supplemented with various concentrations of individual metals. The cells were grown in flat-bottom 96-well plates (200 μl/well) at 37°C, and the optical density at 595 nm (OD595) was measured hourly using a CMax Plus plate reader (Molecular Devices). The metal tested here included Fe(II) (FeSO4), ferric iron [Fe(III)] [Fe(NO3)3], Co(II) (CoSO4), Mn(II) (MnSO4), nickel [Ni(II)] (NiSO4), copper [Cu(II)] (CuSO4), and zinc [Zn(II)] (ZnSO4). Since Fe(II) rapidly oxidizes to Fe(III), a fresh FeSO4 solution was prepared before each use, and 1 g/l of trisodium citrate dihydrate (TCD) was added to the medium containing FeSO4 to reduce iron precipitation [Citation7].
Oxidative stress resistance assay. Overnight cultures of the WT, ΔpmtA, and CΔpmtA strains were grown to exponential phase in the presence of 2 mM FeSO4. The cells were harvested and resuspended in an equal volume of fresh medium. The suspension was then diluted in fresh medium supplemented with oxidative agents (H2O2, diamide, or paraquat). The cells were grown in 96-well plates at 37°C, and the OD595 was measured hourly. In a second experiment, the WT, ΔpmtA, and CΔpmtA strains grown to exponential phase were diluted in fresh medium supplemented with oxidative agents and Co(II). The cells were grown in 96-well plates at 37°C, and the OD595 was measured hourly.
Rescue of the ΔpmtA mutant by Mn(II) supplementation. The WT, ΔpmtA, and CΔpmtA strains grown to exponential phase were diluted in fresh medium supplemented with 4 mM FeSO4 or 0.25 mM CoSO4, and increasing concentrations of MnSO4. The cells were grown in 96-well plates at 37°C, and the OD595 was measured hourly.
Streptonigrin sensitivity assay. The WT, ΔpmtA, and CΔpmtA strains grown to exponential phase were diluted in fresh medium supplemented with 2 mM FeSO4 and increasing concentrations of streptonigrin. The cells were grown in 96-well plates at 37°C, and the OD595 was measured hourly.
Spot dilution assays
The WT, ΔpmtA, and CΔpmtA strains were grown to exponential phase and diluted in fresh medium supplemented with 4 mM Fe(NO3)3, 0.125 mM ZnSO4, or H2O. The cultures were further incubated at 37°C. At 3 and 6 h, aliquots were serially diluted 10-fold up to 10−5 dilution, and 5 μl of each dilution was spotted onto ager plates. The plates were photographically documented after approximately 18 h of incubation at 37°C. This assay was also performed for H2O2 sensitivity. The WT, ΔpmtA, and CΔpmtA strains were grown to exponential phase in the presence of 2 mM FeSO4. The cells were harvested and resuspended in an equal volume of fresh medium. The suspension was diluted in fresh medium supplemented with either 0.5 mM H2O2 or H2O, and then incubated at 37°C. At 4 and 6 h, aliquots were serially diluted 10-fold up to 10−5 dilution, and 5 μl of each dilution was spotted onto ager plates. The plates were photographically documented following incubation.
In another assay, the WT, ΔpmtA, and CΔpmtA strains were grown to mid-exponential phase. Each culture was then divided into six equal parts, which were treated with 4 mM FeSO4, 1 g/l TCD, 0.25 mM CoSO4, 4 mM Fe(NO3)3, 0.125 mM ZnSO4, or H2O, respectively. At 2 and 3 h, aliquots were serially diluted 10-fold up to 10−5 dilution, and 5 μl of each dilution was spotted onto ager plates. The plates were then photographically documented following incubation.
Intracellular metal content analysis by ICP-OES
The WT, ΔpmtA, and CΔpmtA strains were grown to early exponential phase (OD600 = 0.3). Each culture was then divided into four aliquots, which were treated for 2 h with 2 mM FeSO4, 1 g/l TCD, 0.125 mM CoSO4, or H2O, respectively. The cells were harvested, washed three times with PBS supplemented with 250 mM EDTA, and three times with PBS. A sample was taken, and the total protein concentration was determined using a Bradford Protein Assay Kit (Sangon Biotech). The remaining samples were centrifuged, resuspended in 66% nitric acid, digested at 70°C for 48 h, diluted to 2% nitric acid with H2O, and analyzed by inductively coupled plasma-optical emission spectroscopy (ICP-OES). The metal content was expressed as μg of metal per g of protein.
Mouse infections
Animal studies were approved by the Animal Welfare and Ethics Committees of Yangzhou University. Forty female BALB/c mice (4–6 weeks old) were randomly divided into four groups. The mice in three of the groups were intraperitoneally infected with 300 μl of PBS containing 3×108 CFU of the WT, ΔpmtA, and CΔpmtA strains, respectively. The mice in the remaining group were intraperitoneally mock-infected with 300 μl of PBS and served as a control. The mice were monitored twice daily over seven days for clinical symptoms and survival rates.
Protein sequence analysis and statistical analysis
Protein sequence alignments were performed using Clustal Omega (https://www.ebi.ac.uk/Tools/msa/clustalo/) and processed using ESPript 3.0 (http://espript.ibcp.fr/ESPript/ESPript/). The structure of PmtA was predicted using SWISS-MODEL (https://www.swissmodel.expasy.org/). The promoter of pmtA was predicted using BPROM (http://linux1.softberry.com/berry.phtml).
Statistical analyses were performed using GraphPad Prism 5. The differences in gene expression were analyzed by 1-way analysis of variance with Bonferroni’s post-test, while the differences in intracellular metal concentrations were analyzed by the two-tailed paired t-test. The mice survival was analyzed using the Log-rank test.
Results
S. suis PmtA is a homolog of Fe(II) efflux pumps found in several gram-positive bacteria
In the S. suis SC19 genome, the product of the B9H01_RS01605 locus is annotated as a heavy metal translocating P-type ATPase [Citation26]. This protein exhibits 60%, 48%, and 46% amino acid sequence identity with Fe(II) efflux pumps from S. pyogenes (PmtA) [Citation10,Citation11], L. monocytogenes (FrvA) [Citation8], and B. subtilis (PfeT) [Citation7], respectively. Hence, this protein was designated as PmtA, and we hypothesized that S. suis PmtA was implicated in Fe(II) efflux. Multiple sequence alignment revealed that PmtA possesses two metal-binding motifs, a Ser-Pro-Cys (SPC) motif and an His-Glu-Gly-Ser-Thr (HEGST) motif located in transmembrane region 4 and 6, respectively (Figure S1). These two motifs are characteristic of the P1B4-type ATPases [Citation31,Citation32]. Located 162 bp upstream of pmtA is the gene encoding the PerR regulator [Citation33], which was also referred to as Zur [Citation34] or Fur [Citation35]; while 134 bp downstream of pmtA is a gene encoding a hypothetical protein (Figure S2). Furthermore, BLASTN analyses revealed that pmtA is present in all complete S. suis genomes, with 91% to 100% sequence identity at the nucleotide level (Table S3).
PmtA expression is upregulated in response to Fe(II), Co(II), and Ni(II)
To assess the involvement of PmtA in metal efflux, we first examined pmtA relative gene expression in response to various metals, including Fe(II), Fe(III), Co(II), Mn(II), Ni(II), Cu(II), and Zn(II). Compared to H2O treatment, the transcript levels of pmtA were 80-fold higher when the SC19 strain was treated with 1 mM Fe(II) (). Furthermore, the expression level of pmtA increased approximately 130- and 136-fold after treatment with Co(II) and Ni(II), respectively (). In contrast, no significant difference in pmtA expression was observed when the SC19 strain was treated with Fe(III), Mn(II), Cu(II), and Zn(II) (). Altogether, these results suggest that S. suis pmtA expression is upregulated in response to an excess of Fe(II), Co(II), or Ni(II), which led us to speculate that PmtA may be involved in the efflux of Fe(II), Co(II), and Ni(II).
PmtA is involved in S. suis growth under iron, cobalt or zinc excess conditions
To explore the role of PmtA in S. suis physiology, we constructed an in-frame deletion mutant of pmtA (ΔpmtA) and the corresponding complementation strain (CΔpmtA). The two strains were confirmed by PCR (Figure S3A), reverse transcription PCR (RT–PCR) (Figure S3B), and DNA sequencing (data not shown). Under normal growth conditions, pmtA expression in CΔpmtA was approximately 160-fold higher than in the WT strain (Figure S3C).
In the absence of Fe(II), all three strains exhibited similar growth rates (A). However, when exposed to various concentrations of Fe(II), ΔpmtA exhibited growth inhibition, and the level of inhibition correlated with the increase in Fe(II) concentration (B–D). The growth defect of ΔpmtA was even more prominent with the increase of Co(II) (E–H), while slightly impaired growth was observed when ΔpmtA was exposed to Fe(III) or Zn(II) (Figure S4A–C). In line with the growth curve results, spot dilution assays showed that ΔpmtA formed less colonies than did the WT strain when grown in the presence of Fe(III) or Zn(II) (Figure S5). The expression of PmtA in CΔpmtA rescued the growth defect of ΔpmtA under iron, cobalt, or zinc excess conditions (, S4A–C, and S5), confirming that this phenotype is due to the deletion of pmtA. Growth curve analyses performed in the presence of Ni(II), Mn(II), and Cu(II) showed no major difference between the WT, ΔpmtA, and CΔpmtA strains (Figure S4D–F).
Figure 2. PmtA is involved in S. suis resistance to iron or cobalt excess. (A–D) The WT, ΔpmtA, and CΔpmtA strains were grown in the absence (A) and presence of 2 mM (B), 3 mM (C), or 4 mM Fe(II) (D). (E-H) Growth curves in the absence (E) and presence of 0.125 mM (F), 0.25 mM (G), or 0.50 mM Co(II) (H). Graphs data are mean values ± SD from three wells.
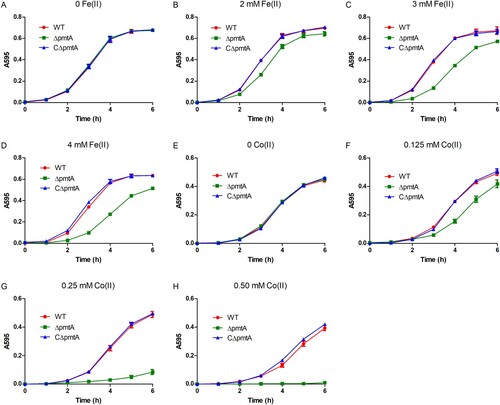
To further asses the role of PmtA in resistance to Fe(II), Co(II), Fe(III), and Zn(II), spot dilution assays were performed. Following treatment for 2 h with Fe(II) (A) or Fe(III) (Figure S6A), ΔpmtA formed less colonies than did the WT and CΔpmtA strains. The effect was even more prominent following 3 h of treatment (B and S6B). However, the three strains displayed similar abilities to form colonies after treatment with Co(II) () or Zn(II) (Figure S6).
Figure 3. Spot dilution assays of the S. suis strains treated with Fe(II) or Co(II). The WT, ΔpmtA, and CΔpmtA strains were grown to mid-exponential phase. Each culture was next treated with 4 mM Fe(II), 1 g/l TCD, 0.25 mM Co(II), or H2O, respectively. At 2 h (A) and 3 h (B), aliquots were serially diluted 10-fold up to 10–5 dilution, and 5 μl of each dilution was spotted onto ager plates. The graphs are representative of three independent experiments.
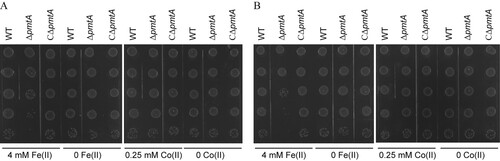
Collectively, our results indicate that PmtA protects S. suis against Fe(II) and Fe(III)-induced bactericidal effect, as well as Co(II) and Zn(II)-induced bacteriostatic effect.
PmtA deletion leads to increased levels of intracellular iron and cobalt
To understand the basis of growth defect of ΔpmtA under Fe(II) or Co(II) excess conditions, we used ICP-OES to analyze the intracellular metal levels in the WT, ΔpmtA, and CΔpmtA strains grown in the absence or presence of Fe(II) or Co(II). Both in the absence and presence of Fe(II), ΔpmtA accumulated significantly increased levels of intracellular iron (A–B). In the absence of Co(II), the intracellular cobalt contents were similar in the three strains (C). Nevertheless, following the addition of Co(II) to the growth medium, the level of intracellular cobalt accumulated was approximately 2-fold higher in ΔpmtA than in the WT and CΔpmtA strains (D). These results indicate that PmtA plays a role in the efflux of both iron and cobalt.
Figure 4. Levels of intracellular iron and cobalt in the WT, ΔpmtA, and CΔpmtA strains. (A–B) Intracellular iron content in the absence (A) and presence (B) of Fe(II). (C–D) Intracellular cobalt content in the absence (C) and presence (D) of Co(II). Graphs data are mean values ± SD from three biological replicates.
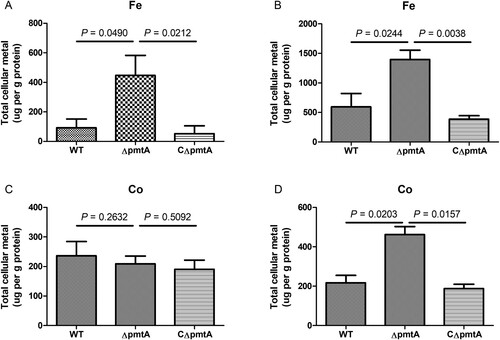
The ΔpmtA mutant is more sensitive to the Fe(II)-activated antibiotic streptonigrin
Streptonigrin is an antibacterial agent that requires iron for its bactericidal activity [Citation36]. Since ΔpmtA accumulates increased levels of intracellular iron, we hypothesized that this mutant would be more sensitive to streptonigrin than the WT or CΔpmtA strains. When grown in medium supplemented with 2 mM Fe(II), ΔpmtA showed only a slight growth defect (A). In stark contrast, ΔpmtA exhibited a remarkable growth defect when grown in medium supplemented with 2 mM Fe(II) and 200 nM streptonigrin (B). Moreover, the growth defect of ΔpmtA was even more severe when the concentration of streptonigrin was increased to 300 nM (C), while growth was almost completely inhibited when the concentration was increased to 500 nM (D). Interestingly, CΔpmtA grew much better than the WT strain following the addition of streptonigrin to the medium (B–D). These results suggest that pmtA deletion can sensitize S. suis to streptonigrin, while overexpression protects against the drug.
Figure 5. ΔpmtA exhibits increased sensitivity to streptonigrin. The S. suis strains were grown in the presence of 2 mM Fe(II) and increasing concentrations of streptonigrin. (A) No streptonigrin, (B) 200 nM streptonigrin, (C) 300 nM streptonigrin, and (D) 500 nM streptonigrin. Graphs data are mean values ± SD from three wells.
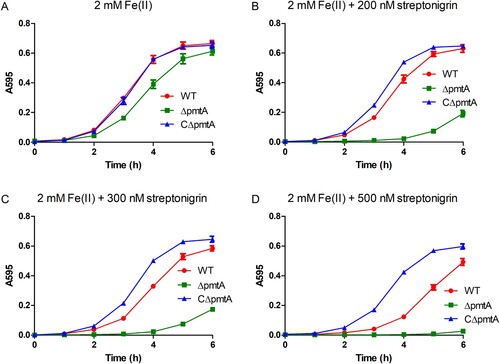
Growth defect of ΔpmtA under Fe(II) or Co(II) excess conditions can be alleviated by Mn(II) supplementation
Previous studies have shown that in B. subtilis and S. pyogenes, inactivation of the Fe(II) efflux pump results in growth defects, which could be rescued by Mn(II) supplementation [Citation7,Citation11]. Based on these observations, we sought to determine whether the Fe(II) and Co(II) toxicity observed in ΔpmtA could be alleviated by Mn(II) supplementation. While the growth of ΔpmtA was inhibited by 4 mM Fe(II) (A), the addition of Mn(II) to the medium rescued the growth of ΔpmtA under Fe(II) excess conditions (B–D). Similarly, growth defect of ΔpmtA under Co(II) excess conditions was alleviated following Mn(II) supplementation (E–H).
Figure 6. Mn(II) supplementation rescues growth defect of ΔpmtA. (A–D) The WT, ΔpmtA, and CΔpmtA strains were grown in the presence of 4 mM Fe(II) alone (A) or 4 mM Fe(II) with 0.25 mM (B), 0.50 mM (C), or 1 mM Mn(II) (D). (E-H) Growth curves in the presence of 0.25 mM Co(II) alone (E) or 0.25 mM Co(II) with 0.25 mM (F), 0.50 mM (G), or 1 mM Mn(II) (H). Graphs data are mean values ± SD from three wells.
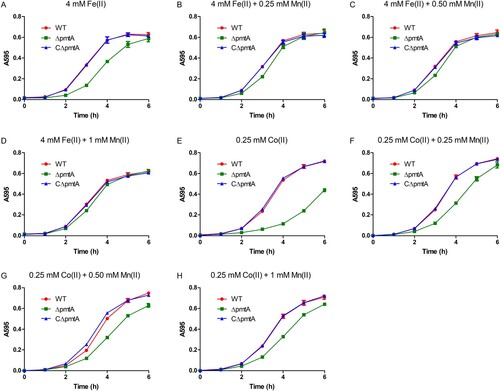
PmtA plays a role in oxidative stress resistance in S. suis
Previous studies have revealed that the Fe(II) efflux mediated by PmtA is important for oxidative stress resistance in S. pyogenes [Citation10,Citation11]. We, therefore, examined the role of S. suis PmtA in oxidative stress tolerance using growth curve analyses. The WT, ΔpmtA, and CΔpmtA strains were pretreated with 2 mM Fe(II) and then diluted in fresh medium supplemented with individual oxidative agents. The growth curves of all three strains were almost identical when grown in normal medium (A). In medium supplemented with H2O2, ΔpmtA exhibited a moderate growth defect, while CΔpmtA grew better than the WT strain (B–C). ΔpmtA grown in the presence of 0.5 mM H2O2 also formed less colonies (D–E). In contrast, the three strains exhibited similar growth in medium supplemented with diamide (Figure S7A) or paraquat (Figure S7B). We also examined the sensitivity of the WT, ΔpmtA, and CΔpmtA strains towards these oxidative stress agents in the presence of 0.125 mM Co(II). We did not observe major differences when we compared the growth of these strains in medium supplemented with Co(II) alone and Co(II) with an oxidative agent (Figure S7C–F). Together, these results suggest that S. suis PmtA plays a role in tolerance to H2O2-induced oxidative stress.
Figure 7. PmtA is involved in S. suis resistance to H2O2-induced oxidative stress. The S. suis strains were grown to exponential phase in the presence of 2 mM FeSO4. The cells were collected, diluted in fresh medium, and grown in the absence (A) and presence of 0.5 mM (B) or 0.75 mM H2O2 (C). Graphs data are mean values ± SD from three wells. (D–E) Spot dilution assays of the S. suis strains grown in the presence of 0.5 mM H2O2. At 4 h (D) and 6 h (E), aliquots were removed from the cultures, serially diluted 10-fold up to 10–5 dilution, and 5 μl of each dilution was spotted onto the plates from 10–1 (top) to 10–5 (bottom). The graphs are representative of three independent experiments.
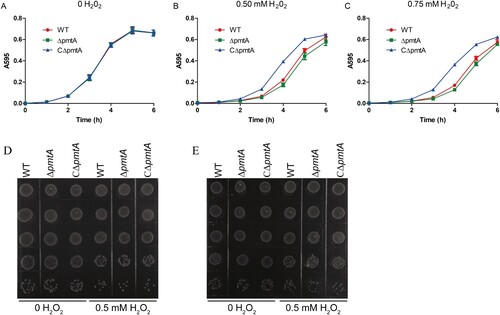
PmtA deletion does not affect S. suis virulence in mice
To evaluate the role of PmtA in S. suis virulence, we conducted an experimental infection study of BALB/c mice. Four groups of ten mice were inoculated intraperitoneally with PBS as a control or 3×108 CFU of the WT, ΔpmtA, or CΔpmtA strains. Following injection, the mice in the PBS group exhibited no sign of infection and survived through the experiment. However, the mice challenged with S. suis developed typical clinical symptoms, such as lethargy and prostration, within 12 h post-infection. Most of the infected mice died during the following days. The final survival rates for the WT, ΔpmtA, and CΔpmtA groups were 10%, 20%, and 20%, respectively (). We did not find significant differences between the survival rates of the ΔpmtA and WT groups (P = 0.9088), and those of the ΔpmtA and CΔpmtA groups (P = 0.8690). Therefore, our data strongly suggest that PmtA does not contribute to the virulence of S. suis in mice.
Discussion
The acquisition of transition metals is critical for both bacterial survival and pathogenesis. In S. suis, previous studies have demonstrated that several metal acquisition systems are required for full virulence [Citation35,Citation37,Citation38]. In contrast to metal acquisition, metal efflux has attracted much less attention. Only recently, we showed that MntE acts as a Mn(II) export system that contributes to S. suis virulence [Citation23]. To better understand the physiology and pathogenesis of S. suis, other metal efflux systems should be investigated.
Here, we report that PmtA functions as an Fe(II) and Co(II) efflux pump in S. suis. We provided evidence that: (i) S. suis PmtA exhibits a high level of identity with Fe(II) efflux systems from S. pyogenes, L. monocytogenes, and B. subtilis, which can also efflux Co(II) [Citation7,Citation8,Citation10,Citation11]; (ii) pmtA expression is significantly upregulated in response to Fe(II) or Co(II); (iii) ΔpmtA is highly sensitive to Fe(II) or Co(II) excess; (iv) ΔpmtA accumulates increased level of intracellular iron and cobalt; (v) pmtA deletion increases the sensitivity to streptonigrin, a Fe(II)-activated antibiotic. We also showed that growth defects of ΔpmtA under Fe(II) or Co(II) excess conditions could be alleviated by Mn(II) supplementation. Further more, we demonstrated that PmtA is implicated in tolerance to H2O2-induced oxidative stress. Although pmtA expression was not induced by Fe(III), both growth curves and spot dilution assays showed that ΔpmtA exhibited growth inhibition under Fe(III) excess conditions. A possible explanation is that the upper regulator of PmtA could not sense Fe(III). Interestingly, similar results have been reported for PfeT, a homolog of PmtA [Citation7]. In B. subtilis, a pfeT null mutant displayed increased sensitivity to Fe(III) excess, whereas the ATPase activity of PfeT could not be activated by Fe(III) [Citation7]. Under Zn(II) excess conditions, ΔpmtA showed a small growth defect, while CΔpmtA grew better than the WT strain. The involvement of PmtA in Zn(II) transport has also been described in the ΔperR mutant of S. pyogenes [Citation9]. Fur is involved in iron uptake and storage in many bacteria [Citation39]. The expression of Fur regulon might be different in ΔpmtA, which is defective in iron efflux. In S. suis, Fur is required for Zn(II) resistence [Citation34]. Thus, we speculate that growth defect of ΔpmtA under Zn(II) excess conditions might be due to the difference in expression of Fur regulon. Conversely, treatment of S. suis with Ni(II) resulted in a remarkable upregulation of pmtA expression, yet ΔpmtA exhibited no growth defect under Ni(II) excess conditions. Unlike the PmtA homologs in certain bacteria that are regulated by PerR or Fur [Citation7–11], PmtA is not under PerR (Fur) control in S. suis [Citation33,Citation34]. The DNA-binding sequence of S. suis AdcR corresponds to the TTAACNRGTTAA motif [Citation40]. In S. suis, a TTAACTTAGTTAA sequence is 33 bp in front of pmtA; thus, pmtA might belong to AdcR regulon. In S. pneumoniae, the AdcR regulon was highly induced by Ni(II) [Citation41]. Therefore, we speculated that the induction of pmtA under Ni(II) excess conditions might be due to AdcR, or that the involvement of PmtA in tolerance to Ni(II) excess might be masked by the presence of other Ni(II) efflux mechanisms in S. suis.
The maintenance of the Fe/Mn ratio is important for bacterial physiology. In S. pyogenes, the deletion of either the Mn(II) or Fe(II) efflux pump resulted in growth inhibition under metal excess conditions, which could be restored by the addition of another metal [Citation6,Citation11]. Moreover, the Fe(II) sensitivity of a B. subtilis pfeT null mutant can be suppressed by Mn(II) supplementation [Citation7]. In line with these observations, our results showed that growth defect of ΔpmtA under Fe(II) excess conditions was partly rescued following Mn(II) supplementation. Similarly, the addition of Mn(II) to the growth medium was able to relieve Co(II) toxicity. A possible mechanism for Fe(II) and Co(II) toxicity is a mismetallation affecting the activity of enzymes that require other metal cofactors, such as Mn(II) [Citation7,Citation42]. This hypothesis is in line with our observation that the addition of Mn(II) to the growth medium was able to alleviate the toxic effects of Fe(II) and Co(II) excess.
Fe(II) toxicity is also closely related to the oxidative stress resulting from the Fenton reaction [Citation43]. The reaction between Fe(II) and H2O2 generates highly reactive hydroxyl radicals, which can cause DNA damage and membrane stress [Citation1]. It is, therefore, reasonable to speculate that PmtA contributes to S. suis resistance to oxidative stress by mediating the efflux of Fe(II). In support of this hypothesis, our results showed that following pretreatment with Fe(II), ΔpmtA grew less efficiently in the presence of H2O2. Similar observations have been made for the Fe(II) efflux pumps of Salmonella enterica [Citation44] and S. pyogenes [Citation10,Citation11]. Also, it is not surprising that CΔpmtA, in which pmtA expression is significantly increased, grew better than the WT strain under H2O2 stress conditions.
It is well established that certain metal efflux systems contribute to bacterial virulence [Citation5,Citation6,Citation8,Citation23]. However, the role of PmtA in streptococcal pathogenesis remains controversial [Citation10,Citation11]. While VanderWal et al. demonstrated that PmtA is critical for S. pyogenes virulence in two mouse models of invasive infection [Citation10], Turner et al. reported that ΔpmtA exhibits no virulence defect in a mouse model of invasive disease [Citation11]. Here, we examined the involvement of PmtA in S. suis virulence using a mouse model of infection. Our results clearly showed that PmtA does not affect the virulence of S. suis in mice. Consistent with this result, Arenas et al. recently demonstrated that pmtA (SSU0288) is downregulated at all sites of infection and plays no major role in S. suis survival within the host using the piglet infection model [Citation45]. Based on these observations, it is conceivable that the host may not employ iron toxicity to control bacterial infections.
In conclusion, the results presented here clearly demonstrate that PmtA is a Fe(II) and Co(II) efflux pump in S. suis. We also show that Fe(II) efflux mediated by PmtA is involved in the resistance to oxidative stress, while PmtA has no effect on S. suis virulence in mice.
Supplemental Material
Download Zip (13 MB)Acknowledgements
We thank Dr Sekizaki (National Institute of Animal Health, Japan) for supplying plasmid pSET4s and pSET2.
Disclosure statement
No potential conflict of interest was reported by the authors.
Additional information
Funding
References
- Turner AG, Ong CY, Walker MJ, et al. Transition metal homeostasis in Streptococcus pyogenes and Streptococcus pneumoniae. Adv Microb Physiol. 2017;70:123–191. doi: 10.1016/bs.ampbs.2017.01.002
- Andreini C, Bertini I, Cavallaro G, et al. Metal ions in biological catalysis: from enzyme databases to general principles. J Biol Inorg Chem. 2008;13(8):1205–1218. doi: 10.1007/s00775-008-0404-5
- Guilhen C, Taha MK, Veyrier FJ. Role of transition metal exporters in virulence: the example of Neisseria meningitidis. Front Cell Infect Microbiol. 2013;3:102. doi: 10.3389/fcimb.2013.00102
- Honsa ES, Johnson MD, Rosch JW. The roles of transition metals in the physiology and pathogenesis of Streptococcus pneumoniae. Front Cell Infect Microbiol. 2013;3:92. doi: 10.3389/fcimb.2013.00092
- Ong CL, Gillen CM, Barnett TC, et al. An antimicrobial role for zinc in innate immune defense against group A Streptococcus. J Infect Dis. 2014;209(10):1500–1508. doi: 10.1093/infdis/jiu053
- Turner AG, Ong CLY, Gillen CM, et al. Manganese homeostasis in group A Streptococcus is critical for resistance to oxidative stress and virulence. mBio. 2015;6(2):e00278–15. doi: 10.1128/mBio.00278-15
- Guan G, Pinochet-Barros A, Gaballa A, et al. Pfet, a P1B4-type ATPase, effluxes ferrous iron and protects Bacillus subtilis against iron intoxication. Mol Microbiol. 2015;98(4):787–803. doi: 10.1111/mmi.13158
- Pi H, Patel SJ, Arguello JM, et al. The Listeria monocytogenes Fur-regulated virulence protein FrvA is an Fe(II) efflux P1B4-type ATPase. Mol Microbiol. 2016;100(6):1066–1079. doi: 10.1111/mmi.13368
- Brenot A, Weston BF, Caparon MG. A PerR-regulated metal transporter (PmtA) is an interface between oxidative stress and metal homeostasis in Streptococcus pyogenes. Mol Microbiol. 2007;63(4):1185–1196. doi: 10.1111/j.1365-2958.2006.05577.x
- VanderWal AR, Makthal N, Pinochet-Barros A, et al. Iron efflux by PmtA is critical for oxidative stress resistance and contributes significantly to group A Streptococcus virulence. Infect Immun. 2017;85(6):e00091–17. doi: 10.1128/IAI.00091-17
- Turner AG, Ong CY, Djoko KY, et al. The PerR-regulated P1B-4-type ATPase (PmtA) acts as a ferrous iron efflux pump in Streptococcus pyogenes. Infect Immun. 2017;85(6):e00140–17. doi: 10.1128/IAI.00140-17
- Lun ZR, Wang QP, Chen XG, et al. Streptococcus suis: an emerging zoonotic pathogen. Lancet Infect Dis. 2007;7(3):201–209. doi: 10.1016/S1473-3099(07)70001-4
- Wertheim HFL, Nghia HDT, Taylor W, et al. Streptococcus suis: an emerging human pathogen. Clin Infect Dis. 2009;48(5):617–625. doi: 10.1086/596763
- Goyette-Desjardins G, Auger JP, Xu J, et al. Streptococcus suis, an important pig pathogen and emerging zoonotic agent-an update on the worldwide distribution based on serotyping and sequence typing. Emerg Microbes Infect. 2014;3(6):e45.
- Tohya M, Arai S, Tomida J, et al. Defining the taxonomic status of Streptococcus suis serotype 33: the proposal for Streptococcus ruminantium sp nov. Int J Syst Evol Micr. 2017;67(9):3660–3665. doi: 10.1099/ijsem.0.002204
- Tang JQ, Wang CJ, Feng YJ, et al. Streptococcal toxic shock syndrome caused by Streptococcus suis serotype 2. Plos Med. 2006;3(5):668–676. doi: 10.1371/journal.pmed.0030151
- Yu HJ, Jing HQ, Chen ZH, et al. Human Streptococcus suis outbreak, Sichuan, China. Emerg Infect Dis. 2006;12(6):914–920. doi: 10.3201/eid1206.051194
- Anita N, Marta K, Peter S. Special case of purulent meningitis caused by Streptococcus suis. case report. Orv Hetil. 2019;160(1):30–34. doi: 10.1556/650.2019.31243
- Yanase T, Morii D, Kamio S, et al. The first report of human meningitis and pyogenic ventriculitis caused by Streptococcus suis: a case report. J Infect Chemother. 2018;24(8):669–673. doi: 10.1016/j.jiac.2018.01.009
- Raberahona M, Rasoanandrasana S, Rahajamanana VL, et al. Novel Streptococcus suis sequence type 834 among humans, Madagascar. Emerg Infect Dis. 2018;24(2):391–392. doi: 10.3201/eid2402.171138
- Gomez-Torres J, Nimir A, Cluett J, et al. Human case of Streptococcus suis disease, Ontario, Canada. Emerg Infect Dis. 2017;23(12):2107–2109. doi: 10.3201/eid2312.171005
- Rajahram GS, Hameed AA, Menon J, et al. Case report: two human Streptococcus suis infections in Borneo, Sabah, Malaysia. BMC Infect Dis. 2017;17(1):188. doi: 10.1186/s12879-017-2294-z
- Xu J, Zheng C, Cao M, et al. The manganese efflux system MntE contributes to the virulence of Streptococcus suis serotype 2. Microb Pathog. 2017;110:23–30. doi: 10.1016/j.micpath.2017.06.022
- Niven DF, Ekins A. Iron content of Streptococcus suis and evidence for a dpr homologue. Can J Microbiol. 2001;47(5):412–416. doi: 10.1139/w01-027
- Pulliainen AT, Kauko A, Haataja S, et al. Dps/Dpr ferritin-like protein: insights into the mechanism of iron incorporation and evidence for a central role in cellular iron homeostasis in Streptococcus suis. Mol Microbiol. 2005;57(4):1086–1100. doi: 10.1111/j.1365-2958.2005.04756.x
- Teng L, Dong X, Zhou Y, et al. Draft genome sequence of hypervirulent and vaccine candidate Streptococcus suis strain SC19. Genome Announc. 2017;5(3):e01484–16. doi: 10.1128/genomeA.01484-16
- Bustin SA, Benes V, Garson JA, et al. The MIQE guidelines: minimum information for publication of quantitative real-time PCR experiments. Clin Chem. 2009;55(4):611–622. doi: 10.1373/clinchem.2008.112797
- Livak KJ, Schmittgen TD. Analysis of relative gene expression data using real-time quantitative PCR and the 2(-Delta Delta C(T)) method. Methods. 2001;25(4):402–408. doi: 10.1006/meth.2001.1262
- Takamatsu D, Osaki M, Sekizaki T. Thermosensitive suicide vectors for gene replacement in Streptococcus suis. Plasmid. 2001;46(2):140–148. doi: 10.1006/plas.2001.1532
- Takamatsu D, Osaki M, Sekizaki T. Construction and characterization of Streptococcus suis-Escherichia coli shuttle cloning vectors. Plasmid. 2001;45(2):101–113. doi: 10.1006/plas.2000.1510
- Arguello JM. Identification of ion-selectivity determinants in heavy-metal transport P1B-type ATPases. J Membr Biol. 2003;195(2):93–108. doi: 10.1007/s00232-003-2048-2
- Arguello JM, Gonzalez-Guerrero M, Raimunda D. Bacterial transition metal P1B-ATPases: transport mechanism and roles in virulence. Biochem. 2011;50(46):9940–9949. doi: 10.1021/bi201418k
- Zhang T, Ding Y, Li T, et al. A Fur-like protein PerR regulates two oxidative stress response related operons dpr and metQIN in Streptococcus suis. BMC Microbiol. 2012;12:85. doi: 10.1186/1471-2180-12-85
- Feng YJ, Li M, Zhang HM, et al. Functional definition and global regulation of Zur, a zinc uptake regulator in a Streptococcus suis serotype 2 strain causing streptococcal toxic shock syndrome. J Bacteriol. 2008;190(22):7567–7578. doi: 10.1128/JB.01532-07
- Aranda J, Garrido ME, Fittipaldi N, et al. The cation-uptake regulators AdcR and Fur are necessary for full virulence of Streptococcus suis. Vet Microbiol. 2010;144(1–2):246–249. doi: 10.1016/j.vetmic.2009.12.037
- Yeowell HN, White JR. Iron requirement in the bactericidal mechanism of streptonigrin. Antimicrob Agents Ch. 1982;22(6):961–968. doi: 10.1128/AAC.22.6.961
- Aranda J, Cortes P, Garrido ME, et al. Contribution of the FeoB transporter to Streptococcus suis virulence. Int Microbiol. 2009;12(2):137–143.
- Schreur PJW, Rebel JMJ, Smits MA, et al. Troa of Streptococcus suis is required for manganese acquisition and full virulence. J Bacteriol. 2011;193(19):5073–5080. doi: 10.1128/JB.05305-11
- Aranda J, Garrido ME, Fittipaldi N, et al. Protective capacities of cell surface-associated proteins of Streptococcus suis mutants deficient in divalent cation-uptake regulators. Microbiol. 2009;155(Pt 5):1580–1587. doi: 10.1099/mic.0.026278-0
- Aranda J, Garrido ME, Cortes P, et al. Analysis of the protective capacity of three Streptococcus suis proteins induced under divalent-cation-limited conditions. Infect Immun. 2008;76(4):1590–1598. doi: 10.1128/IAI.00987-07
- Manzoor I, Shafeeq S, Afzal M, et al. The regulation of the AdcR regulon in Streptococcus pneumoniae depends both on Zn2+- and Ni2+-availability. Front Cell Infect Microbiol. 2015;5:91. doi: 10.3389/fcimb.2015.00091
- Barras F, Fontecave M. Cobalt stress in Escherichia coli and Salmonella enterica: molecular bases for toxicity and resistance. Metallomics. 2011;3(11):1130–1134. doi: 10.1039/c1mt00099c
- Pi H, Helmann JD. Ferrous iron efflux systems in bacteria. Metallomics. 2017;9(7):840–851. doi: 10.1039/C7MT00112F
- Frawley ER, Crouch ML, Bingham-Ramos LK, et al. Iron and citrate export by a major facilitator superfamily pump regulates metabolism and stress resistance in Salmonella Typhimurium. Proc Natl Acad Sci U S A. 2013;110(29):12054–12059. doi: 10.1073/pnas.1218274110
- Arenas J, Bossers-de Vries R, Harders-Westerveen J, et al. In vivo transcriptomes of Streptococcus suis reveal genes required for niche-specific adaptation and pathogenesis. Virulence. 2019;10(1):334–351. doi: 10.1080/21505594.2019.1599669