ABSTRACT
Vibrio vulnificus is a halophilic estuarine bacterium causing severe opportunistic infections. To successfully establish an infection, V. vulnificus must adapt to redox fluctuations in vivo. In the present study, we show that deletion of V. vulnificus fexA gene caused hypersensitivity to acid and reactive oxygen species. The ΔfexA mutant exhibited severe in vivo survival defects. For deeper understanding the role of fexA gene on the successful V. vulnificus infection, we analyzed differentially expressed genes in ΔfexA mutant in comparison with wild type under aerobic, anaerobic or in vivo culture conditions by genome-scale DNA microarray analyses. Twenty-two genes were downregulated in the ΔfexA mutant under all three culture conditions. Among them, cydAB appeared to dominantly contribute to the defective phenotypes of the ΔfexA mutant. The fexA deletion induced compensatory point mutations in the cydAB promoter region over subcultures, suggesting essentiality. Those point mutations (PcydSMs) restored bacterial growth, motility, cytotoxicity ATP production and mouse lethality in the ΔfexA mutant. These results indicate that the cydAB operon, being regulated by FexA, plays a crucial role in V. vulnificus survival under redox-fluctuating in vivo conditions. The FexA-CydAB axis should serve an Achilles heel in the development of therapeutic regimens against V. vulnificus infection.
Introduction
Vibrio vulnificus is a halophilic estuarine bacterium that causes opportunistic infection with high mortality [Citation1]. To establish successful infections in vivo, V. vulnificus should adapt to environmental changes [Citation2]. In the human body, different tissues are supplied with varying concentrations of O2, depending on their specific metabolic demands. The intestinal tissue faces daily fluctuations in perfusion and the gastrointestinal tract is characterized by a steep oxygen gradient across the epithelial layer [Citation3]. Oxygen serves an important signal molecule for the global regulation of gene expression in bacterial pathogens [Citation4]. V. vulnificus is a facultative aerobe that grows best in oxygen but can also multiply in the absence of oxygen. The intestinal mucosa is the first host barrier encountered by V. vulnificus upon oral intake. Considering the oxygen level fluctuations in tissues, it is crucial for V. vulnificus to develop a redox controlling system that can monitor oxygen concentration changes and regulate global gene expression to establish successful infections.
Bacterial pathogens have evolved with complex and organized systems for sensing and maintaining O2 homeostasis. Bacterial adaptation to O2 changes occurs either via direct transcriptional control by altering the DNA binding affinity of the sensory protein or two-component signaling cascades. FNR protein, the most representative direct O2-sensing transcription regulator, is activated at oxygen concentrations from 0 to 5 mbar (0–0.5%), the role of which has been attributed to anaerobic adaptation [Citation5,Citation6]. The Arc (anoxic redox control) system is a typical two-component regulator of global gene expression in response to changing oxygen concentrations. The Arc system comprises the cytoplasmic response regulator ArcA and the transmembrane sensor kinase ArcB [Citation7]. It has been well reported that the ArcBA two-component system globally regulates the expression of genes involved in aerobic, anaerobic and microaerobic catabolic pathways in E. coli [Citation5,Citation6,Citation8]. As a global regulator of metabolism and respiration, dysfunction of the ArcBA system is likely to attenuate the infective nature of a pathogen.
Our group has been working on V. vulnificus genes responsible for in vivo virulence expression and survival in an attempt to identify new therapeutic targets [Citation2,Citation9–11]. In the present investigation, we targeted the redox adaptation system and show that deletion of V. vulnificus fexA, a homologue of arcA. The V.vulnificus fexA is 84% identical in amino acid sequence to E. coli arcA [Citation12]. The ΔfexA mutant caused hypersensitivity to acid and reactive oxygen species while fnr deletion had no significant effect. To understand underlying mechanism of fexA on the V. vulnificus pathogenicity, we analyzed differentially expressed genes in ΔfexA mutant compared to wild type (WT) under aerobic, anaerobic or in vivo culture conditions by a microarray transcriptomic analysis. Twenty-two genes were downregulated in ΔfexA under all three culture conditions. We constructed deletion or site-directed mutants and tested virulence traits. Through molecular genetic characterization of those genes, we discovered the cydAB operon encoding cytochrome d oxidase complex as an Achilles heel of V. vulnificus that may be targeted for the development of new antimicrobials. The FexA-CydAB axis appeared to play a pivotal role in the adaptation to oxygen changes and energy production of V. vulnificus for in vivo survival and successful infection.
Materials and methods
Bacterial strains, plasmids, and media
Bacterial strains and plasmids used in the present study are enlisted in Supplementary Table S1. Detailed experimental procedures are in the “Supplementary information.”
Mutant construction
The chromosomal in-frame deletion and site-directed mutants were constructed by allelic exchange [Citation9,Citation10,Citation13–16]. Primers used for PCR are listed in Supplementary Table S2. Detailed experimental procedures are in the “Supplementary Information.”
Complementation and reversion
For complementation of the mutants, DNA fragments containing WT genes and their native promoters were generated by PCR using pfu polymerase (Stratagene, La Jolla, CA) with CMCP6 genomic DNA as the template using primers in Supplementary Table S2. Amplified product was purified, digested and cloned into the broad host range vector pLAFR3II [Citation16]. The resulting plasmids were transferred into the mutant strains by tri-parental mating using a conjugative helper plasmid, pRK2013 [Citation17]. Detailed experimental procedures are in the “Supplementary Information.”
Bacterial growth
BD GasPakTM EZ Anaerobe Gas Generating Pouch System with Indicator and BD GasPakTM EZ Campy Gas Generating Pouch System were used for checking the anaerobic and microaerobic growth, respectively. In vivo growth was assayed in the rat peritoneal cavity dialysis tube implantation model as previously described [Citation10]. Detailed experimental procedures are in the “Supplementary Information.”
Ethics Statement
All animal experimental procedures were approved by the Institutional Animal Care and Use Committee in Chonnam National University under protocol CNU IACUC-H-2015-44 and animals were maintained following IACUC guidelines.
Survival assays of bacteria exposed to oxidative challenge and acidic pH
V. vulnificus cells were inoculated into fresh 2.5 HI broth containing 1 mM H2O2 to the final cell concentration of 1 × 107 CFU/ml and incubated at 37°C for 1 h with shaking at 200 rpm. Viable bacterial cells were determined in time course. Acid tolerance was examined as described previously with minor modifications using 10 mM sodium citrate buffer (pH 5.0) supplemented with 2% NaCl [Citation18].
Determination of bacterial count in the ligated ileal loop and the blood circulation
The bacterial growth in the intestine was determined by viable bacterial counting. Detailed experimental procedures are in the “Supplementary Information.”
Microarray analysis. Microarray analysis was performed under aerobic, anaerobic and in vivo growth conditions. Detailed experimental procedures are in the “Supplementary Information.”
Intracellular ATP level determination
Intracellular ATP level of bacteria was measured by using the ATP Determination kit (Invitrogen, Eugene, OR) in accordance with the manufacturer's protocol. Detailed experimental procedures are in the “Supplementary Information.”
LacZ reporter construction and β-Galactosidase assay
The promoter-lacZ fusions were constructed by cloning the PCR fragments containing the promoter sequences into pTL61T plasmid [Citation19,Citation20]. Detailed experimental procedures are in the “Supplementary Information.”
Identification of DNA binding proteins
Proteins that bound the cydAB promoter region were identified as previously described [Citation21]. Detailed experimental procedures are in the “Supplementary Information.”
Motility and cytotoxicity assay
For the motility test, 1 µl of 109 CFU/ml logarithmic growth phase bacterial suspension was inoculated onto the 2.5% NaCl HI plates solidified with 0.3% agar (Bacto agar, Difco). The plates were incubated at 37°C and zones of migration were observed after 7 h [Citation10,Citation14–16]. Detailed experimental procedures are in the “Supplementary Information.”
LD50 determination
The 50% lethal doses (LD50) of the WT and mutant V. vulnificus were determined with normal, iron-overloaded and sucking mice as described previously [Citation15]. Detailed experimental procedures are in the “Supplementary Information.”
Statistical analysis
Statistical significance was evaluated using Student's t-test. Statistical values were calculated using Graph Pad Prism 6 or Microsoft Excel, as appropriate. All experiments were repeated three times, and results from representative experiments are shown.
Results
The ΔfexA mutant demonstrated growth defect under aerobic, microaerobic and anaerobic conditions.
The ΔfexA manifested significantly retarded growth compared with the WT strain under aerobic, microaerobic and anaerobic culture conditions. On the other hand, the growth of the Δfnr was significantly retarded only under anaerobic conditions, while no growth defects were noted under aerobic and microaerobic culture conditions. When the ΔfexA strain was complemented with plasmid-encoded fexA, the growth deficiency under aerobic conditions was fully restored ((A)). These results indicate that FexA plays an important role in V. vulnificus growth under wide spectrum redox status.
Figure 1. The ΔfexA mutant exhibit growth defect and severe deficiencies in acid tolerance, reactive oxygen resistance and in vivo survival. (A) Bacterial growth under aerobic, microaerobic and anaerobic conditions was determined. (B) Acid tolerance under 10 mM sodium citrate (pH 5.0) was determined as percent survival. (C) Bacterial survival in 2.5 HI broth containing 1 mM H2O2 was determined. (D) Intra-intestinal survival, growth and subsequent invasion into blood stream of V. vulnificus strains were determined. (E) Survival of mice intraperitoneally infected with the V. vulnificus strains were determined (n = 17 ∼18). The error bars represent standard errors. *P < 0.05; **P < 0.01, ***p < 0.001.
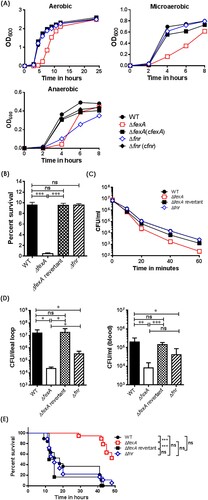
The ΔfexA mutant was defective in acid tolerance and reactive oxygen resistance
To compare the effect of the FexA or FNR on V. vulnificus virulence, we tested resistance to H2O2 and acidic pH. For this set of studies, we used a ΔfexA revertant instead of plasmid-based complemented strains. The complementing plasmid seemed to be unstable and easily lost under stressful conditions, such as H2O2 or acid challenge. The ΔfexA mutant displayed significantly decreased acid tolerance and increased susceptibility to H2O2 ((B,C)), whereas the Δfnr mutant showed WT level survival. The acid tolerance deficiency of the ΔfexA was fully restored in the WT revertant. The revertant strain of ΔfexA showed a similar survival rate to that of the WT strain ((B,C)).
The ΔfexA mutant was defective in intra-intestinal survival, growth and subsequent invasion/growth in blood stream
To test the role of FexA and FNR in the adaptation mechanism of V. vulnificus to oxygen fluctuation during intestinal infection, we carried out an intestinal infection experiment employing mouse ileal loop as the portal of entry. Briefly, we inoculated WT, ΔfexA, ΔfexA revertant or Δfnr strain into ligated ileal loop and counted viable bacteria in the ligated ileal loop (on TCBS) and blood circulation (on 2.5 HI) to evaluate intestinal survival, growth and subsequent invasion/growth into blood stream. Viable bacteria in bloodstream at the time of counting should reflect V. vulnficus cells successfully invaded and grew in the bloodstream. The ΔfexA mutant showed defective survival in the ileal loop (p < 0.05) and decreased invasion/growth into the blood circulation (p < 0.01) compared with WT. To evaluate the role of FNR in comparison with FexA, we also tested Δfnr in the same experimental setting. The Δfnr mutant also showed defective survival in the ileal loop (p < 0.05) and decreased invasion/growth in blood circulation (p < 0.05). The defects of ΔfexA were significantly more profound than Δfnr ((D)). To further investigate the role of FexA and FNR in the gastrointestinal adaptation, we determined intragastric LD50 (ig LD50) of the WT, ΔfexA and Δfnr mutants using suckling mice. The ΔfexA and Δfnr mutants showed 75 and 2.3 fold increase of ig LD50, respectively (Supplementary Table S3). The ΔfexA-infected mice showed significantly delayed survival (p < 0.001 by Log-rank Mantel–Cox test) while the Δfnr did not show significant difference (p > 0.05) compared with WT ((E)). These results clearly showed that fexA plays more dominant roles in adapting oxygen fluctuation in vivo and subsequent successful intestinal infection. Consequently, we focused on the role of FexA in the V. vulnificus gene expression to address the defects.
Differentially expressed genes in the ΔfexA strain under aerobic, anaerobic and in vivo conditions were identified by a DNA microarray analysis
In an effort to understand the underlying mechanism of the virulence deficiency of the ΔfexA strain, we carried out genome-scale transcriptome analysis using a DNA microarray in comparison with the WT strain under aerobic, anaerobic and in vivo growth conditions. By focusing on the ORFs that changed by 2-fold or more, we found that the expression of 800 (18.1% of the genome) genes was significantly affected by the fexA mutation under aerobic, anaerobic or in vivo growth conditions. The differentially expressed genes in the ΔfexA strain were classified into clusters of orthologous groups (COGs) for functional analyses (Supplementary Figure S1). Change in the gene expression pattern was most distinct in in vivo cultures from other culture conditions, while in vitro aerobic and anaerobic cultures showed less variation. Genes associated with transcription, cellular process and signaling and metabolism were most differentially regulated in the ΔfexA background (Supplementary Figure S1). Among the differentially regulated ORFs, 68 and 22 genes were consistently up- and downregulated, respectively, in the ΔfexA under all growth conditions (). These genes would be defined to belong to core FexA regulon. In the present study, we primarily focused on 22 genes that were commonly downregulated in the absence of FexA (). These 22 genes were located in 9 loci, 5 in chromosome 1 and 4 in chromosome 2. These genes should play axial roles in the FexA regulon.
Figure 2. Differentially expressed genes of the ΔfexA strain under aerobic, anaerobic and in vivo conditions were identified by DNA microarray analysis. Venn diagram showing the extent of overlapping genes that are differentially upregulated or downregulated in the ΔfexA mutant among aerobic, anaerobic and in vivo growth conditions. Downregulated genes of the ΔfexA strain under aerobic, anaerobic and in vivo conditions.
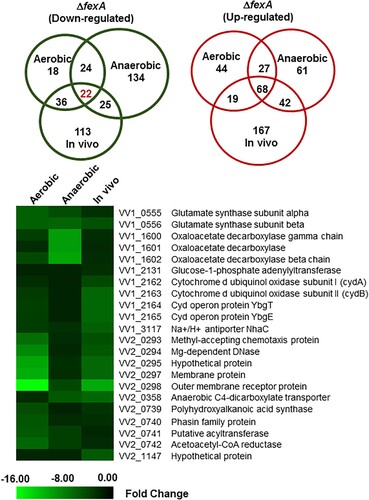
Table 1. Genes down-regulated in ΔfexA strain under aerobic, anaerobic and in vivo conditions (cutoff: fold change 2.0).
FexA-CydAB axis is essential for V. vulnificus survival
To investigate the pathogenic role of the 22 downregulated genes, we constructed in frame deletion or site-directed mutants and tested virulence changes. Notably, no significant virulence change was observed in the deletion mutants of 8 loci except for the cytochrome d ubiquinol oxidase operon (). Because the deletion of cyd operon proved to be lethal, we constructed site-directed cydB mutants by changing amino acids in the highly conserved positions and determined bacterial growth under aerobic condition. As shown in Supplementary Figure S2, the cydB R100H and cydB G144A mutants showed severe growth retardation even compared with the ΔfexA mutant. These results demonstrate that the cytochrome d oxidase complex under FexA regulation is essential for survival.
The ΔfexA mutant induced spontaneous compensatory point mutations in the cydAB promoter region
The ΔfexA formed smaller colonies on 2.5 HI plates. Interestingly, when the mutant was revived from frozen stocks, large colony variants appeared on agar (Supplementary Figure S3, arrow). When cydAB gene sequences were determined in the large colony ΔfexA revertants, single nucleotide mutations in the cydAB promoter region were noted. We found four types of point mutations: A to G at −105 bp (PcydSM4), C to A or T at −109 bp (PcydSM2, PcydSM3), and A to C at −117 bp (PcydSM1), as shown in Supplementary Figure S3. The mutation sites are located inside or 5 bp upstream of the second putative FexA binding site.
Compensatory mutations in cydAB promoter region (PcydSMs) significantly enhanced cydAB expression
To confirm whether the single nucleotide mutations in the cydAB promoter region (PcydSM1, PcydSM2, PcydSM3 and PcydSM4) affected cydAB transcription in the ΔfexA mutant background, we constructed five types of cydAB-lacZ reporter plasmids by using the pTL61T vector (pPcydWT, pPcydSM1, pPcydSM2, pPcydSM3 and pPcydSM4) and the resulting reporter plasmids were transformed into ΔfexAΔlacZ and WTΔlacZ strains (Supplementary Table S1). For this experiment, microaerobic culture was used to mimic the intestinal environment where V. vulnificus starts infection [Citation22]. As shown in , in the ΔfexA mutant background, the promoter activities of PcydSM1, PcydSM2, PcydSM3 and PcydSM4 were significantly enhanced compared to those WT cydAB promoter (pPcydWT) under in vitro aerobic, microaerobic and anaerobic conditions (p < 0.001; ). Among the single nucleotide mutant promoter reporter, PcydSM1 showed the highest expression under tested conditions in the ΔfexA background. The activation level of these mutated cydAB promoters in the WT background were similarly enhanced in all four types of mutations, especially under aerobic and microaerobic conditions (Supplementary Figure S4).
Figure 3. Compensatory mutations in the cydAB promoter region significantly enhanced cydAB promoter activity. The cydAB promoter activities were determined by measuring β-galactosidase activity of the cydAB-lacZ reporter plasmids under aerobic, microaerobic and anaerobic growth conditions. The error bars represent standard errors. ***p < 0.001.
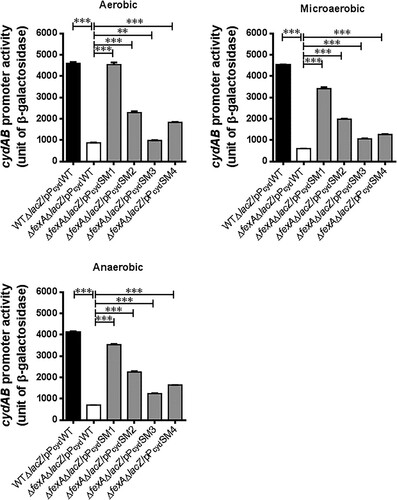
CydAB play a critical role in fexA-mediated regulation of bacterial growth and virulence expression
To address whether the growth retardation and virulence defect of the ΔfexA mutant was due to decreased expression of cydAB, we constructed ΔfexA/PcydSMs strains by swapping the WT cydAB promoter with compensatory mutated ones (PcydSMs) and tested bacterial growth, cytotoxicity and motility of the strains. The bacterial growth retardation ((A)), cytotoxicity deficiency ((B)) and motility defect ((C)) of the ΔfexA mutant was restored in ΔfexA/PcydSMs strains. These results corroborate that compensatory mutations in the cydAB promoter region restored the growth retardation, cytotoxicity and motility of the ΔfexA mutant.
Figure 4. Compensatory mutations in the cydAB promoter region reversed phenotypic changes of the ΔfexA mutant. (A) Bacterial growth was monitored by measuring the OD600 value of cultures at different time points. Cytotoxicity (B) and motility (C) of the WT strain and respective mutants were determined. The error bars represent standard errors **p < 0.01.
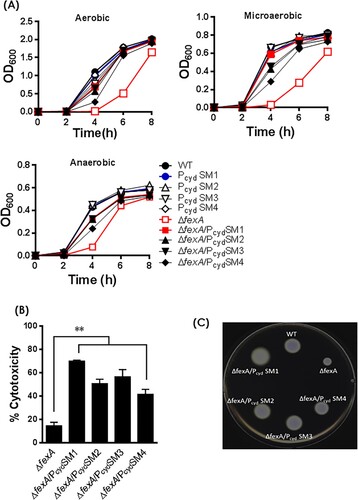
The FexA-CydAB axis modulates energy production of V. vulnificus
Given that cytochrome d oxidase is the sole terminal oxidase in the electron transport chain of V. vulnificus and cydAB expression is downregulated in the ΔfexA mutant, we hypothesized that ΔfexA can produce less amount of ATP and PcydSM1 replacement would restore the ATP production. As expected, the intracellular ATP level of the ΔfexA mutant grown under aerobic, microaerobic and anaerobic conditions was significantly lower than that of the WT strain, which was restored by introduction of the four types of mutations in the promoter region ((A)). These results explain that the low levels of intracellular ATP in the ΔfexA mutant resulting from decreased expression of cydAB contribute to the growth and virulence deficiencies under, at least, aerobic and microaerobic conditions.
Figure 5. Compensatory mutations in the cydAB promoter region restore ATP production and the deficiencies of H2O2 resistance and in vivo survival of ΔfexA mutant. (A) Intracellular ATP production was measured under aerobic, microaerobic and anaerobic growth conditions. (B) Bacterial survival in 2.5 HI broth containing 1 mM H2O2 was determined. (C) In vivo growth of the ΔfexA mutant in the rat peritoneal cavity (n = 4) was determined by using a dialysis tube implantation model. (D) Bacterial growth in minimal essential medium (MEM) supplemented with 0.2% glucose, 0.2% glycerol or 0.2% succinate under aerobic culture condition was determined. The error bars represent standard errors. *p < 0.05; **p < 0.01; ns, not significant.
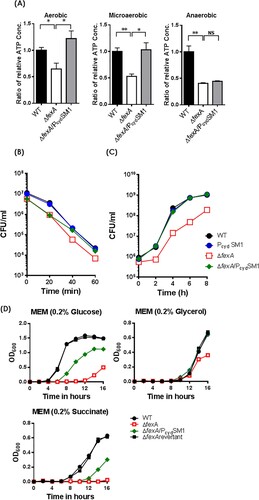
The FexA-CydAB axis is essential for the adaptation of oxygen fluctuations and in vivo survival of V. vulnificus
Introduction of the PcydSM1 mutation in the cydAB promoter region of the ΔfexA strain also fully restored its resistance to H2O2 ((B)) and in vivo growth ((C)), as well as the LD50s of the ΔfexA/PcydSM1 strain to the level of the WT strain in normal i.p., iron-overloaded i.p., and suckling mouse i.g. infection (). These results indicate that the FexA-CydAB axis dominantly modulates energy production in infecting V. vulnficus in response to variable in vivo oxygen tension for their survival and virulence expression. To further understand the regulation of FexA on the cydAB in the context of metabolism, we further determined bacterial growth in a minimal essential medium (MEM) supplemented with different carbon sources such as glucose, glycerol, or succinate (a non-fermentable carbon source). In contrast to the wild type, the ΔfexA mutant manifested delayed growth and was completely defective growing in 0.2% succinate MEM media (non-fermentable carbon source). In addition, the growth defects of ΔfexA mutant were fully recovered in the fexA revertant in all culture conditions and a compensatory point mutation in the cydAB promoter region (ΔfexA/PcydSM1) restored bacterial growth in 0.2% succinate MEM media ((D)). These results corroborate that FexA directly regulates cydAB, critical components of complex III in the electron transfer system of V. vulnificus using succinate for proton generation and that the FexA plays a pivotal role in in vivo proliferation of V. vulnificus via regulation of expression of the cydAB.
Table 2. Effect of mutations on the lethality of V. vulnificus in mice.
The PcydSM1 compensatory mutation enhanced the cydAB promoter and fexA binding
To further address the role of FexA on the cydAB promoter and determine why compensatory point mutations in the cydAB promoter region enhanced cydAB transcription, we analyzed the components of the Pcyd-protein complex by employing a biotin bead-based precipitation assay as described in Material and Method section. As shown in , the WT cydAB promoter (PcydWT) was bound by five proteins under aerobic culture conditions; FexA, deoxycytidylate deaminase, PepA, LeuO, and SeqA. Notably, PcydSM1 compensatory point mutation significantly increased the binding of FexA protein to the cydAB promoter region. When the ΔfexA mutant lysate was incubated with the PcydWT DNA fragment, PepA, LeuO, SeqA and transcriptional regulator HexR were identified ((A,B)). Notably, PcydSM1 bound to H-NS, while PcydWT did not. In the Pcyd promoter region, multiple T-N11-A sequences were observed. Among them, two sites (−231 ∼ −277 bp and −46 ∼ −84 bp) were predicted to be putative LeuO binding sites and overlapped the putative FexA (−265 ∼ −274 bp), FNR (−236 ∼ −249 bp), SeqA (−238 ∼ −241 bp) and HexR (−48 ∼ −67 bp) binding sites. These results suggest that the FexA protein binds to the Pcyd promoter and counteracts probable transcriptional repressors to promote expression. In the absence of FexA, compensatory single nucleotide mutations, such as PcydSM1 confering a lowered binding affinity to transcription repressors, should lead to the expression of crucial survival genes.
Figure 6. Analysis of the cydAB promoter-binding protein complex. (A) Determination of the cydAB promoter binding proteins. A biotin-labeled DNA fragment of the cydAB promoter region was affixed to streptavidin-conjugated Dynabeads, and then incubated with V. vulnificus cytoplasmic extract. Non-adhering and low-specificity DNA-binding proteins were removed by repeated washing and DNA-binding proteins were eluted. Single protein bands were cut from the SDS-PAGE gel for MALDI-TOF Mass Spectrometry assay. (B) The promoter region of the cydAB operon. Bioinformatic analysis suggests two putative FNR binding (blue colour), two putative FexA binding (red colour), two LeuO binding (highlighted), one HexR (green colour) and one SeqA (violet colour) sites.
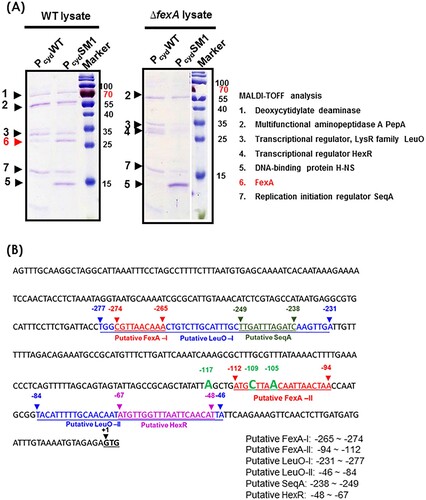
Discussion
For the successful infection, opportunistic pathogens must adapt to redox fluctuations in vivo. The ArcA and FNR systems have been reported to be involved in the fine-tuning of metabolism in response to oxygen fluctuations in various bacteria [Citation8,Citation23–30]. In V. vulnificus, deletion of fexA, an arcA ortholog, attenuated a plethora of V. vulnificus functions, including in vitro growth and in vivo survival, resistance to reactive oxygen species (ROS) and acidic pH, motility, cytotoxicity and mouse lethality, all of which contribute to pathogenicity in vivo. A whole transcriptome analysis of the WT and ΔfexA under aerobic, anaerobic and in vivo conditions provided a glimpse of the V. vulnificus FexA regulon. In the FexA regulon, terminal cytochrome d oxidases (cydAB) play critical roles for survival and virulence expression of V. vulnificus in response to oxygen availability changes in the host environment. The cydAB expression decreased in the ΔfexA strain under all of the tested culture conditions and deletion of cydAB gene resulted in lethal mutation. The ΔfexA formed smaller colonies on 2.5 HI plates compared to WT. Notably, some ΔfexA colonies reverted to its original size by spontaneous transversion point mutations in the cydAB promoter region. The compensatory point mutations in the cydAB promoter region significantly enhanced its expression level in WT and the ΔfexA mutant. Furthermore, a compensatory point mutation, PcydSM1, reversed all attenuated phenotypes of the ΔfexA. The FexA protein was detected in the cydAB promoter-bound protein complex and the PcydSM1 compensatory mutation enhanced the cydAB promoter-FexA binding. Conclusively, we presume that the terminal cytochrome d oxidases are Achilles heel of V. vulnficus and may serve as potential therapeutic targets against V. vulnificus.
E. coli has two cytochrome oxidase complexes, cytochrome d oxidase and cytochrome o oxidase (cyoABCDE), to generate the proton motive force for ATP biosynthesis. However, V. vulnificus CMCP6 does not harbour any homologues of E. coli cyoABCDE. Accumulating reports have shown that inactivation of redox regulators or the terminal oxidase induce negative effect on bacterial stress resistance and survival under in vivo conditions [Citation31–34]. Given that CydAB is the sole cytochrome oxidase complex and deletion of cydAB was lethal to V. vulnificus (), the cydAB is indispensable for the energy production under the control of FexA during V. vulnificus infection.
In the present study, based upon transcriptome data and KEGG database, we present how the energy would be generated by the FexA-CydAB axis in vivo (). It is predicted that at least three enzymatic complexes in the cytoplasmic membrane would generate proton gradient contributing to ATP production. The KEGG database predicts that NADH dehydrogenase, CydAB, and cytochrome c oxidase will generate protons (www.genome.jp/kegg-bin/show_pathway?vvu00190+VV1_2074). Notably, only the CydAB operon in the electron transfer chain was under the control of FexA and a deletion mutation of the NADH dehydrogenase (VV1_2074) in the first complex could be constructed (data not shown), suggesting a differential role in the electron transfer chain. Redox state maintenance is a key cellular function and is crucial for bacterial adaptability. Compromised redox homeostasis should be detrimental to the survival of bacteria. Many reports addressed that the redox imbalance caused by inactivation of redox regulators or the terminal oxidases has a profound negative effect on bacterial stress resistance and survival in vivo [Citation31–34]. Considering the non-existence of the cytochrome o oxidase operon in the V. vulnificus genome, the cytochrome d oxidase CydAB should never be dispensable for the survival of V. vulnificus, not like other bacteria that harbour both of them, in which cydAB orthologs could be deleted. In addition to cydAB, we also found that multiple genes potentially associated with redox regulation under FexA regulation (). We have constructed deletion mutants for those genes and analyzed their roles in V. vulnificus pathogenicity. Among them, CydAB was the most dominant.
Figure 7. The FexAB two component system regulates the adaptive responses to oxygen availability. Upon stimulation, FexB undergoes autophosphorylation, and a phosphoryl group is transferred to FexA by a His-Asp-His-Asp phosphorelay, which consequently activates the expression of cydAB. CydAB encodes the terminal oxidase of the electron transport pathway, which is essential for the survival of V. vulnificus. The most important role played by FexA in V. vulnificus is the activation of cydAB expression.
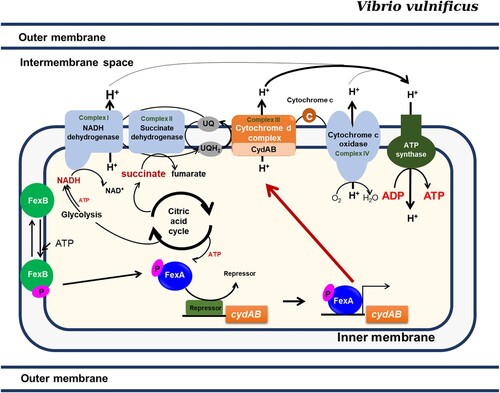
Bacterial motility is highly energy-consuming and the polar vibrio flagellar motor is driven by a Na+ motive force at the expense of ATP [Citation35]. Considering that the expression of flagellar biosynthesis genes was not affected by the FexA mutation and that the motility deficiency of the ΔfexA mutant was fully recovered by increasing cydAB expression, the decreased motility should have contributed to the adhesion defects of ΔfexA. The most potent cytotoxin RtxA1 is expressed upon the close encounter of V. vulnificus with host cells, which is driven by flagellar motility and subsequent adhesion to host cell surface [Citation9,Citation36]. Decreased adhesion should have resulted in the cytotoxicity defect.
Supplemental Material
Download Zip (443.4 KB)Disclosure statement
No potential conflict of interest was reported by the authors.
Correction Statement
This article has been republished with minor changes. These changes do not impact the academic content of the article.
Additional information
Funding
References
- Strom MS, Paranjpye RN. Epidemiology and pathogenesis of vibrio vulnificus. Microbes and Infection/Institut Pasteur. 2000;2(2):177–188. doi: 10.1016/S1286-4579(00)00270-7
- Kim YR, Lee SE, Kim CM, et al. Characterization and pathogenic significance of vibrio vulnificus antigens preferentially expressed in septicemic patients. Infect Immun. 2003;71(10):5461–5471. doi: 10.1128/IAI.71.10.5461-5471.2003
- Taylor CT, Colgan SP. Hypoxia and gastrointestinal disease. J Mol Med. 2007;85(12):1295–1300. doi: 10.1007/s00109-007-0277-z
- Mekalanos JJ. Environmental signals controlling expression of virulence determinants in bacteria. J Bacteriol. 1992;174(1):1–7. doi: 10.1128/jb.174.1.1-7.1992
- Salmon K, Hung SP, Mekjian K, et al. Global gene expression profiling in Escherichia coli K12. The effects of oxygen availability and FNR. J Biol Chem. 2003;278(32):29837–29855. doi: 10.1074/jbc.M213060200
- Sawers G. The aerobic/anaerobic interface. Curr Opin Microbiol. 1999;2(2):181–187. doi: 10.1016/S1369-5274(99)80032-0
- Lynch AS, Lin EC. Transcriptional control mediated by the ArcA two-component response regulator protein of Escherichia coli: characterization of DNA binding at target promoters. J Bacteriol. 1996;178(21):6238–6249. doi: 10.1128/jb.178.21.6238-6249.1996
- Gunsalus RP, Park SJ. Aerobic-anaerobic gene regulation in Escherichia coli: control by the ArcAB and Fnr regulons. Res Microbiol. 1994;145(5–6):437–450. doi: 10.1016/0923-2508(94)90092-2
- Kim YR, Lee SE, Kook H, et al. Vibrio vulnificus RTX toxin kills host cells only after contact of the bacteria with host cells. Cell Microbiol. 2008;10(4):848–862. doi: 10.1111/j.1462-5822.2007.01088.x
- Duong-Nu TM, Jeong K, Hong SH, et al. All three TonB systems are required for vibrio vulnificus CMCP6 tissue invasiveness by controlling flagellum expression. Infect Immun. 2016;84(1):254–265. doi: 10.1128/IAI.00821-15
- Kim HU, Kim SY, Jeong H, et al. Integrative genome-scale metabolic analysis of vibrio vulnificus for drug targeting and discovery. Mol Syst Biol. 2011;7:460. doi: 10.1038/msb.2010.115
- Ju HM, Hwang IG, Woo GJ, et al. Identification of the vibrio vulnificus fexA gene and evaluation of its influence on virulence. J Microbiol Biotechn. 2005;15(6):1337–1345.
- Miller VL, Mekalanos JJ. A novel suicide vector and its use in construction of insertion mutations: osmoregulation of outer membrane proteins and virulence determinants in vibrio cholerae requires toxR. J Bacteriol. 1988;170(6):2575–2583. doi: 10.1128/jb.170.6.2575-2583.1988
- Kim SY, Thanh XT, Jeong K, et al. Contribution of six flagellin genes to the flagellum biogenesis of vibrio vulnificus and in vivo invasion. Infect Immun. 2014;82(1):29–42. doi: 10.1128/IAI.00654-13
- Lee SE, Kim SY, Kim CM, et al. The pyrH gene of vibrio vulnificus is an essential in vivo survival factor. Infect Immun. 2007;75(6):2795–2801. doi: 10.1128/IAI.01499-06
- Kim SY, Lee SE, Kim YR, et al. Regulation of vibrio vulnificus virulence by the LuxS quorum-sensing system. Mol Microbiol. 2003;48(6):1647–1664. doi: 10.1046/j.1365-2958.2003.03536.x
- Ditta G, Stanfield S, Corbin D, et al. Broad host range DNA cloning system for gram-negative bacteria: construction of a gene bank of Rhizobium meliloti. Proc Natl Acad Sci U S A. 1980;77(12):7347–7351. doi: 10.1073/pnas.77.12.7347
- Rhee JE, Jeong HG, Lee JH, et al. Aphb influences acid tolerance of vibrio vulnificus by activating expression of the positive regulator CadC. J Bacteriol. 2006;188(18):6490–6497. doi: 10.1128/JB.00533-06
- Linn T, St Pierre R. Improved vector system for constructing transcriptional fusions that ensures independent translation of lacZ. J Bacteriol. 1990;172(2):1077–1084. doi: 10.1128/jb.172.2.1077-1084.1990
- Miyoshi S, Shinoda S. Activation mechanism of human Hageman factor-plasma kallikrein-kinin system by vibrio vulnificus metalloprotease. FEBS Lett. 1992;308(3):315–319. doi: 10.1016/0014-5793(92)81301-2
- Jutras BL, Verma A, Stevenson B. Identification of novel DNA-binding proteins using DNA-affinity chromatography/pull down. Curr Protoc Microbiol. 2012. Chapter 1:Unit1 F 1.
- He G, Shankar RA, Chzhan M, et al. Noninvasive measurement of anatomic structure and intraluminal oxygenation in the gastrointestinal tract of living mice with spatial and spectral EPR imaging. Proc Natl Acad Sci U S A. 1999;96(8):4586–4591. doi: 10.1073/pnas.96.8.4586
- Salmon KA, Hung SP, Steffen NR, et al. Global gene expression profiling in Escherichia coli K12: effects of oxygen availability and ArcA. J Biol Chem. 2005;280(15):15084–15096. doi: 10.1074/jbc.M414030200
- Chao G, Shen J, Tseng CP, et al. Aerobic regulation of isocitrate dehydrogenase gene (icd) expression in Escherichia coli by the arcA and fnr gene products. J Bacteriol. 1997;179(13):4299–4304. doi: 10.1128/jb.179.13.4299-4304.1997
- Park SJ, Chao G, Gunsalus RP. Aerobic regulation of the sucABCD genes of Escherichia coli, which encode alpha-ketoglutarate dehydrogenase and succinyl coenzyme A synthetase: roles of ArcA, Fnr, and the upstream sdhCDAB promoter. J Bacteriol. 1997;179(13):4138–4142. doi: 10.1128/jb.179.13.4138-4142.1997
- Nystrom T, Larsson C, Gustafsson L. Bacterial defense against aging: role of the Escherichia coli ArcA regulator in gene expression, readjusted energy flux and survival during stasis. EMBO J. 1996;15(13):3219–3228. doi: 10.1002/j.1460-2075.1996.tb00686.x
- Nunn WD. A molecular view of fatty acid catabolism in Escherichia coli. Microbiol Rev. 1986;50(2):179–192.
- Lin EC, Iuchi S. Regulation of gene expression in fermentative and respiratory systems in Escherichia coli and related bacteria. Annu Rev Genet. 1991;25:361–387. doi: 10.1146/annurev.ge.25.120191.002045
- Liu X, De Wulf P. Probing the ArcA-P modulon of Escherichia coli by whole genome transcriptional analysis and sequence recognition profiling. J Biol Chem. 2004;279(13):12588–12597. doi: 10.1074/jbc.M313454200
- Shalel-Levanon S, San KY, Bennett GN. Effect of ArcA and FNR on the expression of genes related to the oxygen regulation and the glycolysis pathway in Escherichia coli under microaerobic growth conditions. Biotechnol Bioeng. 2005;92(2):147–159. doi: 10.1002/bit.20583
- Endley S, McMurray D, Ficht TA. Interruption of the cydB locus in Brucella abortus attenuates intracellular survival and virulence in the mouse model of infection. J Bacteriol. 2001;183(8):2454–2462. doi: 10.1128/JB.183.8.2454-2462.2001
- Henard CA, Bourret TJ, Song M, et al. Control of redox balance by the stringent response regulatory protein promotes antioxidant defenses of Salmonella. J Biol Chem. 2010;285(47):36785–36793. doi: 10.1074/jbc.M110.160960
- Alexeeva S, Hellingwerf KJ, de Mattos MJT. Requirement of ArcA for redox regulation in Escherichia coli under microaerobic but not anaerobic or aerobic conditions. J Bacteriol. 2003;185(1):204–209. doi: 10.1128/JB.185.1.204-209.2003
- Tribelli PM, Nikel PI, Oppezzo OJ, et al. Anr, the anaerobic global regulator, modulates the redox state and oxidative stress resistance in Pseudomonas extremaustralis. Microbiology. 2013;159(Pt 2):259–268. doi: 10.1099/mic.0.061085-0
- Atsumi T, McCarter L, Imae Y. Polar and lateral flagellar motors of marine vibrio are driven by different ion-motive forces. Nature. 1992;355(6356):182–184. doi: 10.1038/355182a0
- Lee JH, Rho JB, Park KJ, et al. Role of flagellum and motility in pathogenesis of vibrio vulnificus. Infect Immun. 2004;72(8):4905–4910. doi: 10.1128/IAI.72.8.4905-4910.2004