ABSTRACT
Gram-negative marine bacterium Vibrio parahaemolyticus is an important aquatic pathogen and has been demonstrated to be the causative agent of acute hepatopancreatic necrotic disease (AHPND) in shrimp aquaculture. The AHPND-causing V. parahaemolyticus strains contain a pVA1 plasmid encoding the binary PirAVP and PirBVP toxins, are the primary virulence factor that mediates AHPND and mortality in shrimp. Since PirABVP toxins are secreted extracellularly, one can hypothesize that PirABVP toxins would aggravate vibriosis in the aquatic environment. To address this, in vivo and in vitro experiments were conducted. Germ-free Artemia franciscana were co-challenged with PirABVP toxins and 10 Vibrio spp. The in vivo results showed that PirABVP toxin interact synergistically with MM30 (a quorum sensing AI-2 deficient mutant) and V. alginolyticus AQ13-91, aggravating vibriosis. However, co-challenge by PirABVP toxins and V. campbellii LMG21363, V. parahaemolyticus CAIM170, V. proteolyticus LMG10942, and V. anguillarum NB10 worked antagonistically, increasing the survival of Artemia larvae. The in vitro results showed that the addition of PirABVP toxins significantly modulated the production of the virulence factors of studied Vibrio spp. Yet these in vitro results did not help to explain the in vivo results. Hence it appears that PirABVP toxins can aggravate vibriosis. However, the dynamics of interaction is strain dependent.
Introduction
Shrimp is a highly traded seafood product [Citation1–3]. Intensification of shrimp production has increased the incidence of disease. In the shrimp aquaculture industry, economic losses from disease outbreak have been estimated by the FAO to be over US$9 billion per year, which is approximately 15% of the value of world farmed shellfish production [Citation4,Citation5]. The bacterial disease problem, often vibriosis, has escalated since late 2010, when the industry collapsed in South-Asian countries [Citation6,Citation7]. The Vibrio harveyi, V. alginolyticus, V. anguillarum, V. splendidus, V. salmonicida, V. vulnificus, and V. parahaemolyticus strains have been found as the main vibriosis-causative microorganisms [Citation6–9].
Apart from “classical” vibriosis, some Vibrio spp. are also responsible for causing acute hepatopancreatic necrosis disease (AHPND), originally known as early mortality syndrome (EMS), having a devastating impact on the shrimp aquaculture industry [Citation10–14]. The shrimp production in AHPND-affected regions has at times dropped considerably (up to 60%) and disease has caused an estimated loss of US $43 billion across Asia (China, Malaysia, Thailand, Vietnam) and in Mexico in last 10 years [Citation15–19]. A Vibrio spp. becomes a virulent AHPND-causing strain by acquiring a pVA1 plasmid (63-70 kb) encoding the binary toxin PirAVP/PirBVP, which consists of two subunits PirAVP and PirBVP, homologous to the Photorhabdus luminescens insect-related (Pir) toxins PirA/PirB [Citation11]. The secreted binary PirAVP and PirBVP toxins are the primary virulence factor of AHPND-causing bacteria mediating AHPND and mortality in shrimp [Citation19–22]. Notably, some recent studies have reported the presence of PirABVP carrying pVA1 plasmids in some non-Vibrio parahaemolyticus such as V. campbellii, V. owenseii, and V. harveyi [Citation23–26]. That sparks the concern about the influence of AHPND-causing PirABVP toxin on the virulence of non-AHPND Vibrio species, when these toxic proteins are released into the aquatic environment or in the host.
However, the critical problem in studying in vivo host-pathogen interaction is the difficulty to either eliminate or extricate the effect of the microbial communities that occur naturally in the system [Citation27]. Additionally, in germ-associated conditions, the microbial communities might influences the physiology of host-associated microbes, thereby making it difficult to understand the host response towards pathogens [Citation28,Citation29]. Consequently, the selection of an appropriate animal model system that permits better delineation of cause and effects in host-pathogen interactions is paramount. The brine shrimp (Artemia franciscana), an aquatic invertebrate that can be reared under gnotobiotic conditions (allowing full control over the host-associated microbial communities) are characteristically small, highly osmotolerant, branchiopod crustacean reported from a variety of harsh environment worldwide [Citation27,Citation30–33]. Apart from its unusual life history, relatively low space and cost requirement for culture, rapid generation cycle (cyst to adult in 20–30 days), well-characterized developmental stages and ability to form cyst which can be stored, makes A. franciscana an exceptional experimental system to study the host and pathogen relationship [Citation34–37]. In addition, the brine shrimp genome sequence shares high homology with the genomes of shrimps and other crustaceans. Hence, the outcome of Artemia-based studies might provide a basis for a better understanding of host-pathogen interactions in commercially important shrimp species [Citation38,Citation39].
In this study, using the highly controlled gnotobiotic brine shrimp model system, it was aimed to investigate whether PirABVP toxin proteins would aggravate the virulence of 10 Vibrio spp., i.e. V. harveyi (wild type and 4 quorum sensing deficient mutants, each of them with a certain degree of virulence attenuation relative to the wild type), V. proteolyticus, V. alginolyticus, V. campbellii, V. anguillarum, and non-AHPND V. parahaemolyticus. Additionally, in vitro experiments were also carried out to determine the bacterial cell viability, biofilm formation, swimming motility, haemolytic activity, caseinase activity, and lipase activity in response to PirABVP toxin. Overall, the results contribute to the understanding of the potential of AHPND toxins to aggravate vibriosis.
Material and methods
Bacterial species and growth conditions
Eleven bacterial species including 10 Vibrio spp. (V. harveyi BB120, BB152, JMH603, MM30, JMH634, V. alginolyticus AQ13-91, V. campbellii LMG21363, V. parahaemolyticus CAIM170, V. proteolyticus LMG10942, and V. anguillarum NB10) and Aeromonas hydrophila LVS3 bacteria were used in the study (). It is important to mention that the strain V. harveyi ATCC strain BAA-1116 (BB120) has been reclassified as Vibrio campbellii based on microarray comparative genome hybridization and multilocus sequence analysis [Citation40,Citation41]. The Vibrio spp. were used for challenge assay, while A. hydrophila LVS3 (autoclaved) served as feed for Artemia larvae. The stock culture of bacteria was streaked onto Marine agar (MA) plates (Carl Roth, Belgium) to obtain pure colonies. Afterwards, a single colony was inoculated in a 50 mL Erlenmeyer flask containing 20 mL marine broth (MB) (Carl Roth, Belgium) and incubated overnight at 28°C under constant agitation (120 rpm). The stock culture of each bacterial species was prepared using 30% glycerol and stored at −80°C until further use.
Table 1. Bacteria species used in this study.
Preparation of recombinant V. parahaemolyticus PirAVP and PirBVP
The PirA (pET21b PirAVP) and PirB plasmid (pET21b PirBVP) obtained from Taiwan [Citation10] were transformed into E. coli Rosetta (DE3) competent cells and the expression and purification of recombinant PirAVP and PirBVP toxins were done as described by Kumar et al. [Citation21]. Briefly, the expression of C-terminal His6-tagged PirAVP and PirBVP proteins was induced by the addition of 0.25 μM of isopropyl thiogalactoside (IPTG). After expression had proceeded at 16°C for 16 h, the recombinant proteins were purified with the magneHis™ protein purification system (Promega Corporation, USA). The purified recombinant PirAVP and PirBVP toxins were dialyzed in phosphate buffer solution (PBS) (Honeywell, Belgium) and concentrated with Amicon® ultra-15 centrifugal filters (Merck Millipore, USA). The acquired PirAVP and PirBVP toxins were collected and immediately preserved at −80°C for further analysis.
Detection of V. parahaemolyticus PirAVP and PirBVP toxins through SDS-PAGE
After purification, the quality and quantity of the recombinant PirAVP and PirBVP proteins was checked by SDS-PAGE and Bradford method, respectively, as described by Kumar et al. (2019). The proteins were electrophoresed in stain-free 4-20% polyacrylamide gel, with each lane receiving an equivalent volume (10 μL) of toxin. The gels were then stained with Coomassie Biosafe (BioRad Laboratories) and visualized by a ChemiDoc MP imaging system (BioRad, Belgium) for qualitative analysis of recombinant PirAVP and PirBVP toxins. The concentration of protein was determined using the Bradford method [Citation42].
Gnotobiotic brine shrimp rearing system
The gnotobiotic brine shrimp larvae were produced by hatching brine shrimp cysts axenically (germ-free) following decapsulation and hatching procedures as described by Kumar et al. [Citation43] with slight modifications. Briefly, 30 mg Artemia franciscana cysts (INVE Aquaculture, Dendermonde, Belgium) was hydrated in 10 mL distilled water with 2 µm filtered aeration for 1 h. Then, 330 µL NaOH (32%) and 5 mL NaOCl (50%) were added to decapsulate the cysts. After 4 min, the decapsulation was stopped by adding 5 mL autoclaved Na2S2O3 (10 g/L). The decapsulated cysts were washed with filtered autoclaved artificial seawater (FASW) containing 35 g/L instant ocean synthetic sea salt (Aquarium Systems, Sarrebourg, France) to get rid of the decapsulation reagents. The cysts were then suspended in 50 mL falcon tubes containing 30 ml FASW followed by incubating at 28°C on the rotor with constant illumination of about 27 µE/m2/sec for 28 h to reach the developmental stage II. After hatching, the axenic condition was checked by spreading 100 µL of hatching water on MA plate and then incubated for 5 days at 28°C [Citation28]. Experiments that started with non-axenic larvae were discarded.
Brine shrimp challenge assay
To investigate whether it was possible to conduct the challenge test in smaller volume of FASW, a total of three separate in vivo assays were performed. At first, the challenge assay was performed in two different containers: glass tubes (10 mL FASW) and Eppendorf tubes (1 mL FASW). The results showed that there was significant difference in the survival of Artemia nauplii in the non-challenged (control) group in both glass tubes and Eppendorf tubes. Statistically, the survival rates of Artemia nauplii in the control were significantly different from those challenged with V. harveyi BB120 (immersion, 107 cells/ml) in two containers after 48 h (Figure S1 A, B). Next, the survival assay was conducted in 96-well plates with 100 µL/well FASW to investigate the survival performance of Artemia nauplii in even smaller volume (Figure S1 C). The experiment was performed with three Artemia nauplii density, i.e. 1 nauplius/well, 5 and 10 nauplii/well. Average survival rate of Artemia nauplii in the control group with a stocking density of 1 nauplius/well was approximately 75% and 25%, in 5 nauplii/well 87% and 35% and 89% and 47% in 10 nauplii/well group after 48 and 60 h post experiment (Figure S1 C). Based on the above results, the 96-well plates containing 10 Artemia nauplii in 100 µL FASW were found suitable and used further for challenge experiments in this study. The McFarland standard (BioMerieux, Marcy L’Etoile, France) was used to determine the bacterial density from optical density (OD) assuming 1.2 × 109 cells/ml at OD of 1 [Citation44].
Next, to determine the feeding regime for Artemia larvae in 96-well plates during 60 h of the experiment, additional survival assay was performed. Along with previously reported feeding regime, i.e. 107 cells/ml used in 10 and 1 ml FASW challenge assay [Citation21,Citation45], an additional feeding 108 cells/ml was used and fed either once or twice to the Artemia larvae. The results indicated that the survival of Artemia larvae fed twice was significantly higher than those fed once after 60 h in both feeding doses (Figure S2 A). However, there was no significant difference in Artemia survival between two feeding levels, i.e. 107 cells/ml or 108 cells/ml. Afterwards, the Artemia larvae were challenged with V. harveyi BB120 strain and the survival was recorded after 48 and 60 h post infection. The results showed that feeding doses (107 or 108 cells/ml) did not result in any significant difference in the survival of Artemia larvae challenged with V. harveyi BB120 strain (Figure S2 B). However, higher survival (%) were recorded in Artemia nauplii fed twice at 108 cells/ml as compared with 107 cells/ml feeding regimes, in the challenge assay with V. harveyi strains (Figure S3). Therefore, the feeding dose 108 cells/ml, fed twice, was used for further experiment.
After standardization, 96-well plates containing 100 µL FASW, 10 A. franciscana larvae per well and feeding twice at 108 LVS3 cells/ml was applied in further challenge assays. In the first experiment, a group of 10 larvae was transferred to 96-well plates and challenged separately with either V. harveyi BB120 (wild type), BB152 (HAI-1 deficient mutant), JMH603 (CAI-1 deficient mutant), MM30 (AI-2 deficient mutant), JMH634 (triple QS mutant), V. alginolyticus AQ13-91, V. campbellii LMG21363, V. parahaemolyticus CAIM170, V. proteolyticus LMG10942 or V. anguillarum NB10 strain (immersion, 107 cells/ml) followed by incubation at 28°C for 60 h. The non-challenge Artemia larvae served as control. The survival rate of Artemia was recorded every 12 h post challenge. The experiment was carried out in 12 replicates equivalent to 12 wells of the plate. Next, the survival assay was carried out using four experimental groups, i.e. only Artemia larvae, Artemia larvae + bacterial strain, Artemia larvae + PirABVP toxin (immersion, 5.2 µg/100 µL) and Artemia larvae + bacterial strain + PirABVP toxin (immersion, 5.2 µg/100 µL). In our previous publications, we found that Pir toxins at 5.2 µg/100 µl induce AHPND, damages gastrointestinal tract, causing mortality in brine shrimp larvae [Citation21,Citation46]. Hence, 5.2 µg/100 µL dose was used to further investigate its role on the virulence of non-AHPND Vibrio species. The plates were maintained at 28°C for 60 h and every 12 h, the survival rate of Artemia was recorded by placing the plates under the microscope. The experiment was carried out in 12 replicates equivalent to 12 wells of the plate.
Cell viability investigation by flow cytometry
At first, cell viability assay was standardized using different dye and bacterial concentration. It was found that Thiazole Orange and Propium Iodide were detected in FITC (fluorescein isothiocyanate) channel and PC5.5 channel, respectively, in the CytoFLEX flow cytometer system (Beckman Coulter’s Life sciences, France). Hence, the plots of PC5.5-A as the vertical axis and FITC-A as a horizontal axis were used to present the results. The results showed that Thiazole Orange was able to stain both live and dead bacterial cells, while Propium Iodide only stained dead cells (Table S1). Notably, the mixture of both dyes was able to distinguish both live and dead cells in the sample better than those only stained with only one dye (Table S1). Therefore, the mixture of Thiazole Orange and Propium Iodide was used for further cell viability test in this study. Moreover, another experiment was carried out to determine how dead cells of tested bacterial strains locate on the PC5.5 × FITC plot. Subsequently, the logarithm of signal emission from dead cells of the examined strains was at least from 103 to 104 on PC5.5 axis. Particularly, the signal areas of dead cells belonging to V. harveyi strains (except for JMH634) and V. anguillarum NB10 were gated from 5 × 103 above while to V. harveyi JMH634 was at 104 above (Figure S4 A, B). The dead cells of other strains emitted the signal at 103 above in terms of area logarithm (Figure S4 C).
Next, the overnight bacteria cultures adjusted to 107 cells/mL were used for flow cytometry analysis. Bacteria suspension supplemented with 5.2 µg/100 µL PirABVP toxin protein was used as treatment while the pure suspension served as control. Afterwards, the bacteria suspension was incubated at 28°C for 24 h under constant agitation (6 rpm) followed by centrifugation (2000× g at 25°C) for 15 min. Subsequently, the pellets were resuspended in FASW and up to 10−3 serial dilution was prepared. Afterwards, 100 µL of acquired suspension was placed into each well of the 96-well plates together with 5 µL Thiazole Orange (17 mM) and 5 µL Propium Iodide (0.15 mM). The mixture of 100 µL of FASW and dyes was used as a negative control. The experiment was conducted in triplicate equivalent to 3 wells of the plate. The plate was then covered by the aluminium foil for 10 min to allow staining reaction to occur. The amount of total count and dead bacterial cells were then determined by CytoFLEX flow cytometer system.
In vitro assay for the biofilm formation, swimming motility, caseinase activity, haemolytic activity, and lipase activity of Vibrio spp.
Before performing the in vitro assay, the bacterial suspension was diluted to 107 cells/mL using sterile MB and supplemented with PirABVP toxin at 5.2 µg/100 µL. The bacteria suspension without PirABVP toxin served as a positive control. Subsequently, a final volume of 500 µL from each group was transferred to Eppendorf tubes and incubated at 28°C for 24 h under constant agitation (6 rpm).
Biofilm formation was quantified as described by Stepanovic et al. [Citation47]. Briefly, the bacterial suspension from two groups described above was adjusted to OD550 of 0.1 and 200 µL aliquots was transferred, in triplicate, into the wells of a sterile 96-well plate. Wells receiving only MB served as a negative control. The plate was incubated at 28°C for 24 h under static conditions to allow the biofilm formation. Afterwards, each well was gently washed three times with 300 µL physiological solution (0.9% NaCl) to remove all the non-adherent bacteria. The wells were supplemented with 200 µL of 99% methanol and incubated for 2 h. The methanol was removed from each well and the plate was air-dried overnight. Subsequently, the wells were stained with 150 µL of 0.1% crystal violet for 20 min, rinsed under a gentle flow of tap water and allowed to air dry. Then, wells were supplemented with 150 µL of 95% ethanol to resolubilize the bounded dye from the adherent bacterial cells and absorbance was measured at 570 nm with an infinite 200 Tecan plate reader (Tecan, Switzerland).
Swimming motility was measured as described by Yang and Defoirdt [Citation48]. The bacterial suspension from two groups described above was adjusted to OD550 of 0.5 and 2 µL of the sample was spotted at the centre of fresh soft MA (0.2% agar) plates. The pure MB served as negative control. The plates were incubated at 28°C for 24 h. Thereafter, the diameters of the motility halos were measured. Each treatment was conducted in triplicate.
Caseinase activity was measured as described by Natrah et al. [Citation49]. The required volume of medium was divided into two parts, one of which consisted of MB (80 g/L) and agar (30 g/L), and the other half was supplemented with 8% (w/v) skim milk powder. The former was autoclaved at 121°C for 20 min while the latter only needed 5 min of autoclaving. After that, the homogenous mixture of both suspensions was poured into plastic Petri dishes and allowed to air dry. The bacterial suspension from two groups described above was adjusted to OD550 of 0.5 and 2 µL of the sample was spotted at the centre of the agar plates. The plates were then incubated at 28°C for 3–4 days. Afterwards, the diameters of the clearing zone and colonies were measured. Each treatment was conducted in triplicate.
Hemolytic activity and lipase activity were measured as described by Natrah et al. [Citation49] on 5% defibrinated sheep blood supplemented with 1% Tween 80 on MA plates. 2 µL of aliquots (OD550 of 0.5) were spotted at the centre of the agar plates followed by the incubation at 28°C for 3–4 days. Pure MB was used as negative control. Afterwards, the diameters of activity zones and colonies were observed. Each treatment was conducted in triplicate.
Statistical analysis
Survival data were arcsine transformed to satisfy normality and homoscedasticity requirements as necessary and then analysed by SPSS software version 24.0. The Independent samples T-test and one-way ANOVA (analysis of variances) followed by Duncan’s multiple range test was used to compare means of survival rate between unchallenged and bacterial challenged shrimp. The interactive effect of PirABVP toxin and tested bacteria were determined by two-way ANOVA (which allows to subsequently determine if the effect is additive, synergistic, or antagonistic) at 5 different time points. Survival in all treatments had to be higher than 10%, at a certain time point, for an interactive effect to be considered and the interpretation of the results to be reliable. Moreover, the data obtained from in vitro tests were analysed by independent samples T-test. The Significance level was set at p < 0.05.
Results
Virulence of Vibrio spp. towards A. franciscana larvae
In the first experiment, the virulence of Vibrio isolates to the host Artemia larvae was investigated by monitoring survival at every 12 h, until 60 h post challenge (). The results illustrated that the virulence of wild type V. harveyi and its derivatives and other studied Vibrio isolates were notably different from each other 48 h post challenge. The Artemia larvae challenged with V. proteolyticus LMG10942, V. campbellii LMG21363, V. parahaemolyticus CAIM170, V. harveyi BB120 (wild type) and BB152 strain (HAI-1 deficient mutant) exhibited significantly high mortality as compared to others Vibrio isolates and control 48 h post challenge. Although, the survival of Artemia larvae in V. alginolyticus AQ13-91, V. harveyi MM30 (AI-2 deficient mutant) and JMH603 strain (CAI-1 deficient mutant) group was significantly lower as compared to the control, the Vibrio-induced mortality was lower than for other Vibrio isolates after 48 h post challenge (). Interestingly, at 60 h post challenge, a similar trend in the survival of Artemia larvae was observed in V. proteolyticus LMG10942, V. campbellii LMG21363, V. anguillarum NB10, V. parahaemolyticus CAIM170, V. harveyi BB120 (wild type) and BB152 strain (HAI-1 deficient mutant) challenged groups. Moreover, V. harveyi JMH634 strain (triple QS mutant) was found to be non-pathogenic and the survival of Artemia larvae was similar to the control group. These results indicate that Vibrio isolates exhibited a different degree of virulence to Artemia larvae. As established before, the virulence of V. harveyi and its derivatives were found to be quorum-sensing (QS) dependent [Citation50,Citation51].
Table 2. Survival (%) of Artemia franciscana at different time points after being challenged with V. harveyi wild type and its quorum sensing mutants (mean ± SD, n = 12).
Effect of PirABVP toxin on virulence factor production and in vivo virulence of V. harveyi wild type and its isogenic quorum-sensing mutants.
An in vitro experiment was conducted aiming to determine the effect of PirABVP toxin on cellular viability of V. harveyi wild type BB120 and mutant strains. The overnight culture of bacteria and PirABVP toxin mixture was tested for live/dead cells using Thiazole Orange and Propium Iodide staining using flow cytometry. The result showed that addition of PirABVP toxins significantly increased the total cell count and percentage of dead cells in the suspension of MM30 strain (AI-2 deficient mutant) (, S2). In contrast, a significant decreased total cell count was recorded in the suspensions of BB152, JMH603, and JMH634 strain supplemented with the toxin. Meanwhile, no significant difference in dead/live cell proportion (dead cells %) was observed in the other V. harveyi strains tested (). The addition of PirABVP toxins had no effect on BB120 strain cellular viability.
Table 3. Average (±SD) cell counts and dead cells percentage of V. harveyi wild type BB120 and its derivatives with or without PirABVP (PirAB+ or PirAB-), obtained from flow cytometer (n = 3).
The supplementation of PirABVP toxin significantly enhances the expression of virulence factors, including swimming motility, caseinase activity, and lipase activity of V. harveyi BB120 strain (wild type), while the haemolytic activity of bacterium was suppressed in the presence of PirABVP (, S3). However, in BB152 (HAI-1 deficient mutant) and MM30 (AI-2 deficient mutant) strains, the addition of PirABVP toxin significantly enhances the swimming motility and caseinase activity (only in BB152 strain), while biofilm formation, haemolytic activity, and lipase activity was not significantly altered (, S4, S5). In addition, PirABVP toxin supplementation increased the biofilm formation and swimming motility of JMH603 (CAI-1 deficient mutant), while increased lipase activity was observed with the JMH634 (triple QS mutant) strain (, S6, S7). These results indicate that addition of PirABVP toxin potentially increases the virulence of the studied V. harveyi BB120 (wild type), MM30 (AI-2 deficient mutant), BB152 (HAI-1 deficient mutant), JMH603 (CAI-1 deficient mutant) and JMH634 (triple QS mutant) strains, while increased total cell count was only reported from V. harveyi MM30 strain.
Table 4. The effect of PirABVP on virulence factors of V. harveyi wild type BB120 and its derivatives (n = 3).
Under the in vivo experiment, at first, the quality of the purified PirAVP and PirBVP toxins was analysed through SDS-PAGE. The purified recombinant PirAVP and PirBVP toxins were found at approximately 13 and 50 kDA, respectively, in SDS-PAGE gels (Figure S5). Next, the effect of PirABVP toxin on the virulence of V. harveyi wild type and its derivatives towards Artemia was investigated (). The results illustrated that PirABVP toxin challenge in combination with either V. harveyi BB120 (wild type), BB152 (HAI-1 deficient mutant), or JMH603 (CAI-1 deficient mutant) display additive effect (no interaction) at 36 and 48 h post challenge and high mortality in Artemia larvae was recorded. At 60 h post challenge, the BB120, BB152, or JMH603 strain displayed a statistically significant synergistic interaction with PirABVP toxin ((A–C)). This synergistic effect, towards the end of the challenge period with survival in some treatments becoming very low, might be an inherent feature of survival data with questionable biological meaning. Moreover, significant synergistic interaction of PirABVP toxin with MM30 (AI-2 deficient mutant) at 36 h (with survival higher than 15% in all treatments) to 60 h post challenge was observed ((D)). In contrast, no considerable interaction between PirABVP toxin and JMH634 (triple QS mutant) strain was detected ((E)). These results suggest that PirABVP toxin can interact with some Vibrio strains (more specifically the AI2 mutant, as such mildly virulent towards Artemia), increasing its virulence towards Artemia larvae while there is no increase in virulence by Pir toxins, when the strain is avirulent (such as the triple QS mutant JMH634).
Figure 1. Survival (%) of Artemia franciscana at different time points after being challenged with PirABVP toxin @ 5.2 µg/100 µl and Vibrio harveyi strains (A) PirABVP toxin and V. harveyi wild type BB120 strain, (B) PirABVP toxin and HAI-1 deficient mutant BB152 strain, (C) PirABVP toxin and CAI-1 deficient mutant JMH603 strain, (D) PirABVP toxin and AI-2 deficient mutant MM30 strain and (E) PirABVP toxin and QS triple mutant JMH634 strain. The error bars represent the standard deviation values of 12 replicates. Asterisks on the top of error bars indicate the significant in vivo interaction, either synergistic or antagonistic interaction between toxin and bacteria, and based on output interaction plot obtained from two-way ANOVA analysis *(p < 0.05), **(p < 0.01), ***(p < 0.001). The effect of the toxin or the Vibrio is not statistically analysed and hence not presented here, only the interactive compound of the 2-way ANOVA is presented. For an interactive statistical effect to be considered of biological relevance, only data at timepoints for which the survival in all treatments was above 10% are considered.
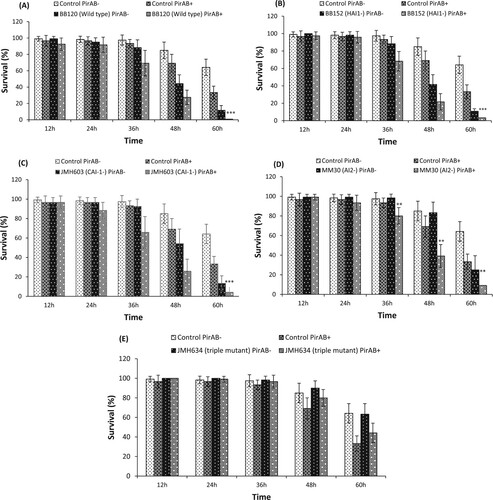
Effect of PirABVP toxin on virulence factor production and in vivo virulence of V. alginolyticus AQ1391
Next, the effect of PirABVP toxin supplementation on in vivo virulence of V. alginolyticus AQ1391 strain was investigated in Artemia larvae. The results showed that in presence of the PirABVP toxin, V. alginolyticus AQ1391 induce significant mortality in Artemia larvae ((A)). In addition, a significant synergistic interaction between PirABVP toxin and V. alginolyticus AQ1391 was observed at 48 and 60 h post challenge.
Figure 2. Survival (%) of A. franciscana at different time points after being challenged with PirABVP toxin @ 5.2 µg/100 µl and (A) V. alginolyticus AQ13-91, (B) V. campbellii LMG21363 (C) V. parahaemolyticus CAIM170, (D) V. proteolyticus LMG10942 and (E) V. anguillarum NB10. The error bars represent the standard deviation values of 12 replicates. Asterisks on the top of error bars indicate the significant in vivo interaction, either synergistic or antagonistic interaction between toxin and bacteria, and based on output interaction plot obtained from two-way ANOVA analysis *(p < 0.05), **(p < 0.01), ***(p < 0.001). The effect of the toxin or the Vibrio is not statistically analysed and hence not presented here, only the interactive compound of the 2-way ANOVA is presented. For an interactive statistical effect to be considered of biological relevance, only data at timepoints for which the survival in all treatments was above 10% are considered.
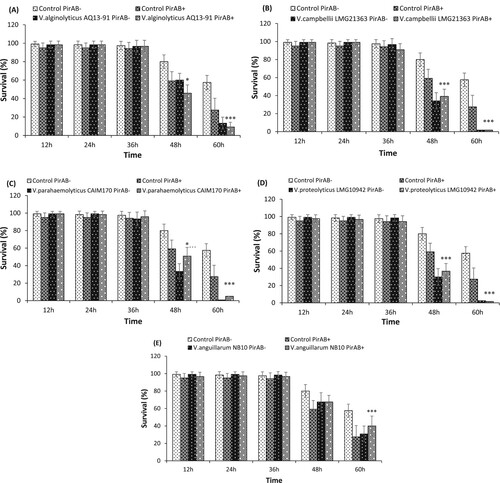
The in vitro results illustrated that PirABVP toxin had no significant effect on the proportion of dead cells of V. alginolyticus AQ1391 strain as compared to the control (p = 0.082490). However, the total cell count in the PirABVP toxin supplemented group was notably higher than the control (, S8). The, V. alginolyticus AQ1391 did not display biofilm formation, haemolytic, caseinase and lipase activity (, S9). Taken together, the results showed that the addition of PirABVP toxin significantly increases the in vivo virulence and total cell count of V. alginolyticus AQ1391 strain. In contrast, PirABVP toxin had no effect on dead/live cell proportion and in vitro virulence of V. alginolyticus.
Table 5. Average (±SD) cell counts and dead cells percentage of V. alginolyticus AQ1391, V. campbellii LMG21363, V. proteolyticus LMG10942, V. parahaemolyticus CAIM170 and V. anguillarum NB10 strains with or without PirABVP (PirAB+ or PirAB-), obtained from flow cytometer (n = 3).
Table 6. The effect of PirABVP on virulence factors of the studied Vibrio spp (n = 3).
Effect of PirABVP toxin on virulence factor production and in vivo virulence of V. campbellii LMG21363
Interestingly, the addition of PirABVP toxin significantly decreases the in vivo virulence of V. campbellii LMG21363 toward Artemia larvae. The results showed that after 48 h post PirABVP toxin and V. campbellii LMG21363 exposure, the survival of brine shrimp larvae was significantly higher by approximately 5% as compared to larvae only challenged with bacteria ((B)).
The cell viability of V. campbellii LMG21363 was affected by the presence of PirABVP toxin. Results showed that dead cells (%) in LMG21363 strain supplemented with PirABVP toxin was lower than the non-supplemented suspension (PirAB-). In addition, the total cell count of LMG21363 strain with PirABVP toxin was significantly higher than the control (, S8). Though, V. campbellii LMG21363 did not exhibit biofilm formation and lipase activity, the presence of PirABVP toxin significantly enhanced the haemolytic activity of LMG21363 strain. Moreover, the addition of PirABVP toxin had no significant effect on swimming motility and caseinase activity of LMG21363 strain (, S10). These results indicate that, PirABVP toxin antagonistically interacts with V. campbellii LMG21363 strain and significantly decreases its in vivo virulence. In addition, the PirABVP toxin significantly increase the in vitro total cell count and haemolytic activity V. campbellii LMG21363, while no effect was observed in swimming motility and caseinase activity.
Effect of PirABVP toxin on virulence factor production and in vivo virulence of V. parahaemolyticus CAIM170
The AHPND-causing PirABVP toxin significantly decreases the in vivo virulence of V. parahaemolyticus CAIM170 strain (antagonistic interaction of PirABVP toxin and CAIM170 strain). The results showed that Artemia larvae challenged together with PirABVP toxin and CAIM170 strain had significantly higher survival at 48 h post exposure as compared with larvae challenged with bacteria only ((C)).
The PirABVP toxin had no significant effect on the cell viability of V. parahaemolyticus CAIM170 strain. The total cell count and live/dead cells proportion were found similar in CAIM170 strain with or without PirABVP toxin addition (, S8). Though, V. parahaemolyticus CAIM170 did not exhibit biofilm formation and lipase activity, the addition of PirABVP toxin was found to increase the swimming motility of bacterium. However, PirABVP toxin supplementation significantly decreases the haemolytic activity of CAIM170 strain (, S11). These results indicate that PirABVP toxin interacts antagonistically with V. parahaemolyticus CAIM170 in vivo and decreases its virulence. Moreover, PirABVP toxin enhances the swimming motility of bacterium, while decreases the haemolytic activity of CAIM170 strain.
Effect of PirABVP toxin on virulence factor production and in vivo virulence of V. proteolyticus LMG10942
Similar to V. campbellii LMG21363, the PirABVP toxin supplementation had antagonistic interaction on in vivo virulence of V. proteolyticus LMG10942 strain towards Artemia larvae. The results showed that, after 48 h exposure with PirABVP toxin and LMG10942 strain, the survival of Artemia larvae significantly increased as compared with larvae challenged with bacteria alone ((D)).
Next, the effect of PirABVP toxin on cell viability of V. proteolyticus LMG10942 was investigated. The data obtained from flow cytometry analysis illustrated that the addition of PirABVP toxin significantly increased the total cell count of LMG10942 strain (, S8). Moreover, the dead cells (%) in LMG10942 were lower in the presence of toxin as compared to control. Among the studied virulence factors, V. proteolyticus LMG10942 did not exhibit haemolytic, caseinase, and lipase activies, while the addition of PirABVP toxin did not affect the swimming motility of V. proteolyticus LMG10942 (, S12). Together, these results show that PirABVP toxin interacts antagonistically with V. proteolyticus LMG10942 in vivo and decreases its virulence. Moreover, PirABVP toxin had no effect on the in vitro virulence of V. proteolyticus LMG10942.
Effect of PirABVP toxin on virulence factor production and in vivo virulence of V. anguillarum NB10
The results showed that the addition of PirABVP toxin had no effect on the virulence of V. anguillarum NB10 strain in Artemia larvae up to 48 h post challenge. The Artemia larvae challenged with V. anguillarum NB10 with or without PirABVP toxin was approximately 68 ± 11% and 68 ± 8%, respectively, after 48 h exposure. However, at 60 h post challenge, the supplementation of PirABVP toxin significantly decreases the virulence of NB10 strain and the average survival (%) of Artemia challenged with the mixture of bacteria and toxin was higher than those challenged with only bacteria ((E)) (antagonistic interaction).
The addition of PirABVP toxin had no effect on the cell viability of V. anguillarum NB10 strain. The results showed that NB10 strain supplemented with PirABVP toxin did not cause significant change in the percentage of the dead cells as compared to the control. Notably, the average total cells count without toxin supplementation was significantly higher than those with toxin (, S8). Moreover, the addition of PirABVP toxin on V. anguillarum NB10 had no effect on studied biofilm formation, lipase activity, haemolytic activity and caseinase activity. In contrast, the presence of PirABVP significantly reduced the swimming motility of V. anguillarum NB10 (, S13). Though, PirABVP toxin interacts antagonistically with V. anguillarum NB10 strain and decrease its virulence (in vivo), the toxin had no significant effect on the in vitro production of virulence factors (except swimming motility) of the NB10 strain.
Discussion
The Gram-negative Vibrio species, ubiquitous in estuarine and coastal waters, are opportunistic aquatic pathogen and responsible for causing AHPND and vibriosis in shrimps [Citation8,Citation20]. For instance, V. harveyi, V. alginolyticus, V. anguillarum, V. splendidus, V. salmonicida, V. vulnificus, and V. parahaemolyticus causing conventional vibriosis in shrimp farming [Citation6–9]. While, pVA1 plasmid associated V. parahaemolyticus, V. punensis, V. harveyi, V. owensii, and V. campbelli are reported to cause AHPND and massive mortality, even up to 100%, in shrimp aquaculture [Citation10–12,Citation21,Citation25,Citation26,Citation52,Citation53]. As AHPND-causing bacteria are releasing extracellular PirABVP toxins, their presence in the aquatic environment, apart from mediating AHPND and mortality in shrimp (@20 µg toxin/g shrimp) [Citation21,Citation54–56], may modulate the intra and inter-species interaction and influence virulence of non-AHPND Vibrio species. To this end, brine shrimp (Artemia franciscana), an aquatic invertebrate crustacean and a model for crustacean shrimp [Citation28,Citation29,Citation36,Citation39,Citation57] was used to investigate in vivo effect of PirABVP toxin on the virulence of non-AHPND vibrios during challenge studies. Subsequently, in vitro experiments were also carried out to determine the bacterial cell viability, biofilm formation, swimming motility, and the haemolytic, caseinase, and lipase activities in the non-AHPND vibrios in response to PirABVP toxin exposure.
Quorum sensing (QS) is a bacterial intercommunication system that controls the expression of numerous genes, thereby regulating the activities of large group of cells [Citation58–60]. QS system use small signal molecules called autoinducers (AIs) that controls the bacterial bioluminescence, virulence factor expression, biofilm formation, motility, entry into stationary phase, sporulation, and mating [Citation61,Citation62]. In vibrios, three parallel QS system have been discovered: AI-1, a typical acylated homoserine lactones (AHLs), CAI-1 (V. cholerae autoinducer 1), and the AI-2, have been designed for inter-species cell-cell communication [Citation63,Citation64]. Hence, we hypothesized that quorum sensing might regulate the PirABVP toxin-induced Vibrio species virulence and subsequent vibriosis in shrimp. To verify this, V. harveyi BB120 (wild type), BB152 (HAI-1 deficient mutant), JMH603 (CAI-1 deficient mutant), MM30 (AI-2 deficient mutant) and JMH634 (triple QS mutant) strains were used, each of them with a certain degree of virulence attenuation relative to the wild type. The results showed that addition of PirABVP toxin synergistically enhanced in vivo virulence of V. harveyi MM30 only, while no significant interaction was observed in triple QS mutant JMH634 strain ((A–D), (A)). Moreover, additive effect was noticed in BB120 and BB152 strains. These observations point in the direction of additive effects for strains that are virulent (wild type or strains in which quorum sensing gene deletions do not affect virulence), synergistic effects for strains with an attenuated (intermediate) virulence (MM30) and no affect for avirulent strains like the triple QS mutant. It is possible that the PirABVP toxins, known to destroy epithelial cells in the Artemia gut [Citation21,Citation22,Citation46,Citation65–69], facilitate the entry of MM30 strain, making epithelial cells a barrier to infection for MM30 cells. Additionally, the PirABVP toxin supplementation also synergistically increases the in vivo virulence of V. alginolyticus AQ13-91 strain (wild type), an intermediate virulent strain. In both cases more research will be needed to unravel the mechanistic nature of the observed synergistic effect.
However, supplementation of PirABVP toxin has significant antagonistic interaction with in vivo virulence of V. campbellii LMG21363, V. parahaemolyticus CAIM170, V. proteolyticus LMG10942, and V. anguillarum NB10 strain ((B–E)). The results showed that, after exposure with PirABVP toxin and Vibrio spp., the survival of Artemia larvae significantly increased as compared with larvae challenged with bacteria alone. The cellular and humoral components of the immune system present in the digestive tract plays an important role in preventing the potential binding and invasion of incoming pathogen [Citation70]. There are few reports which suggest that the administration of bacterial toxins could serve as cross-protective antigens and provide protection against microbial infection. For instance, Campa-Cordona et al. [Citation71] reported that recombinant PirA-like toxin, when administered through bath immersion, enhances the immune response of shrimp, providing protection against AHPND-causing V. parahaemolyticus [Citation71]. In another study, ToxAVP toxin, when administrated to Pacific red snapper Lutjanus peru was reported to positively affect the humoral immune response and upregulate the expression of immune genes that protects the red snapper from V. parahaemolyticus infection [Citation72,Citation73]. As, PirABVP toxin were reported to bind epithelial cells of the digestive tract in brine shrimp larvae, the toxin binding might have induced immunological response and prevented the subsequent attachment and/or entry of pathogenic bacteria. Alternatively, as reported in sea bass larvae (Dicentrarchus labrax), the gut epithelial enterocytes containing lysosomes mediates intracellular elimination of pathogenic V. anguillarum cells [Citation74,Citation75]. And the putative engulfment of bacterial structures through the shedding of intestinal epithelial cells, as an defence strategy in larval fish, were regularly visualized in the gut lumen at the later stage of bacterial challenge [Citation74]. Hence, the observed antagonistic interaction of PirABVP toxin with Vibrio spp., might be based on either toxin-induced enhanced immune response or on shedding of epithelial cells eliminating the pathogenic bacteria, resulting in decreased in vivo virulence of V. campbellii LMG21363, V. parahaemolyticus CAIM170, V. proteolyticus LMG10942, and V. anguillarum NB10 strain ((B–E)). Yet, such interaction did not happen with all the studied Vibrio strains suggesting that the interaction is strain-dependent and might be influenced by the virulence level of bacteria, the mechanistic of which remains to be unravelled.
Moreover, a stochastic pattern of interaction was observed in our in vitro experiment. The results showed that addition of PirABVP toxin significantly increase cell count and dead cell (%) in V. harveyi MM30, V. alginolyticus AQ13-91, V. campbellii LMG21363, and V. proteolyticus LMG10942, while decreased value was recorded from V. harveyi JMH603, V. parahaemolyticus CAIM170, and V. anguillarum NB10 strain ( and ). Similar to cell viability observations, the in vitro virulence results showed that PirABVP toxin addition significantly increases the swimming motility (V. harveyi BB120, BB152, MM30, JMH603, and V. parahaemolyticus CAIM170), caseinase activity (V. harveyi BB120 and BB152) and lipase activity (V. harveyi BB120 and JMH634). However, decreased haemolysis activity in V. harveyi BB120 and V. parahaemolyticus CAIM170, caseinase activity V. harveyi MM30 and JMH603 were also recorded in the presence of PirABVP toxin ( and ). Though, these observations do not help to explain the in vivo results, the preliminary observations suggest that PirABVP toxin influences the cell viability and in vitro virulence of the studied Vibrio spp.
In essence, the results presented here underscore that presence of PirABVP toxin modulates the virulence of Vibrio spp. in both in vivo and in vitro conditions. Although, it remains to be established how PirABVP toxin interacts with Vibrio spp., to the authors’ knowledge, these findings are the first of its kind, possibly pointing towards new insights in the infection process of Vibrio spp. in presence of AHPND-causing PirABVP. Since, in recent years the incidence of AHPND mediated by PirABVP toxin has increased enormously, further studies that focus on the interaction mechanism of PirABVP toxin and Vibrio spp. in commercial shrimp species (e.g. Litopenaeus vannamei, Penaeus monodon or Macrobrachium rosenbergii) will help to better understand PirABVP toxins and Vibrio spp. mediated infection process.
Supporting_information.docx
Download ()Acknowledgements
The authors like to acknowledge the technical assistant provided by Brigitte Van Moffaert, Anita De Haese and Brecht Stechele. Vikash Kumar is supported by Netaji Subhas international doctoral fellowship from Indian Council of Agricultural Research (ICAR), New Delhi. We highly acknowledge the Special Research Fund of Ghent University (BOF12/BAS/042) for procuring the chemiluminescence detection system. The flow cytometry results presented in this publication was carried out with infrastructure funded by EMBRC Belgium - FWO agreement 20151029–03 and Hercules agreement 20140910-03.
Disclosure statement
No potential conflict of interest was reported by the author(s).
Additional information
Funding
References
- FAO. State of fisheries and aquaculture in the world. Rome, Italy; 2019. doi:10.1109/BMEI.2010.5639447.
- Roy S, Kumar V, Manna RK, et al. Sundarbans mangrove deltaic system – an overview of its biodiversity with special reference to fish diversity. J Appl Nat Sci. 2016;8:1090–1099. doi: 10.31018/jans.v8i2.926
- Roy S, Kumar V, Mitra A, et al. Amylase and protease activity in shrimps and prawn of sundarbans, west bengal, India. Indian J Geo-Marine Sci. 2018;47:53–59.
- Chatterjee S, Haldar S. Vibrio related diseases in aquaculture and development of rapid and accurate identification methods. J Mar Sci Res Dev. 2012;s1:1–7.
- Kumar V, Roy S. Aquaculture drugs: sources, active ingredients, pharmaceutic preparations and methods of administration. J Aquac Res Dev. 2017;08:1–13.
- Lee KK, Liu PC, Chuang WH. Pathogenesis of gastroenteritis caused by Vibrio carchariae in cultured marine fish. Mar Biotechnol. 2002;4:267–277. doi: 10.1007/s10126-002-0018-9
- Aguirre-Guzmán G, Ruíz HM, Ascencio F. A review of extracellular virulence product of Vibrio species important in diseases of cultivated shrimp. Aquac Res. 2004;35:1395–1404. doi: 10.1111/j.1365-2109.2004.01165.x
- Defoirdt T, Boon N, Sorgeloos P, et al. Alternatives to antibiotics to control bacterial infections: luminescent vibriosis in aquaculture as an example. Trends Biotechnol. 2007;25:472–479. doi: 10.1016/j.tibtech.2007.08.001
- Kesarcodi-Watson A, Kaspar H, Lategan MJ, et al. Two pathogens of greenshellTM mussel larvae, perna canaliculus: Vibrio splendidus and a V. coralliilyticus/neptunius-like isolate. J Fish Dis. 2009;32:499–507. doi: 10.1111/j.1365-2761.2009.01006.x
- Lee C-T, Chen I-T, Yang Y-T, et al. The opportunistic marine pathogen Vibrio parahaemolyticus becomes virulent by acquiring a plasmid that expresses a deadly toxin. Proc Natl Acad Sci. 2015;112:10798–10803. doi: 10.1073/pnas.1503129112
- Tran L, Nunan L, Redman RM, et al. Determination of the infectious nature of the agent of acute hepatopancreatic necrosis syndrome affecting penaeid shrimp. Dis Aquat Organ. 2013;105:45–55. doi: 10.3354/dao02621
- Dong X, Bi D, Wang H, et al. pirABvp-bearing Vibrio parahaemolyticus and Vibrio campbellii pathogens isolated from the Same AHPND-affected pond possess highly similar pathogenic plasmids. Front Microbiol. 2017;8:1–9.
- Kumar V, Bossier P. Novel plant-based compounds could be useful in protecting shrimp species against AHPND Vibrio parahaemolyticus. J Inl Fish Soc India. 2019;51:03–05.
- Kumar V, Bossier P. Importance of plant-derived compounds and/or natural products in aquaculture. Aquafeed. 2018;10:28–31.
- Tran L, Nunan L, Redman RM, et al. EMS/AHPNS: infectious disease caused by bacteria. Glob Aquac Advocate. 2013: 19–20.
- Nunan L, Lightner D, Pantoja C, et al. Detection of acute hepatopancreatic necrosis disease (AHPND) in Mexico. Dis Aquat Organ. 2014;111:81–86. doi: 10.3354/dao02776
- De La Peña LD, Cabillon NAR, Catedral DD, et al. Acute hepatopancreatic necrosis disease (AHPND) outbreaks in Penaeus vannamei and p. monodon cultured in the Philippines. Dis Aquat Organ. 2015;116:251–254. doi: 10.3354/dao02919
- Shinn AP, Pratoomyot J, Griffiths D, et al. Asian shrimp production and the economic costs of disease. Asian Fish Sci J. 2018;31S:29–58.
- Kumar V, Roy S, Baruah K, et al. Environmental conditions steer phenotypic switching in acute hepatopancreatic necrosis disease-causing Vibrio parahaemolyticus, affecting PirAVP/PirBVP toxins production. Environ Microbiol. 2020.
- Lai HC, Ng TH, Ando M, et al. Pathogenesis of acute hepatopancreatic necrosis disease (AHPND) in shrimp. Fish Shellfish Immunol. 2015;47:1006–1014. doi: 10.1016/j.fsi.2015.11.008
- Kumar V, Viet D, Baruah K, et al. Probing the mechanism of VP AHPND extracellular proteins toxicity purified from Vibrio parahaemolyticus AHPND strain in germ-free Artemia test system. Aquaculture. 2019;504:414–419. doi: 10.1016/j.aquaculture.2019.02.029
- Lin SJ, Hsu KC, Wang HC. Structural insights into the cytotoxic mechanism of Vibrio parahaemolyticus PirAvpand PirBvptoxins. Mar Drugs. 2017;15:9–12. doi: 10.3390/md15010009
- Chonsin K, Matsuda S, Theethakaew C, et al. Genetic diversity of Vibrio parahaemolyticus strains isolated from farmed pacific white shrimp and ambient pond water affected by acute hepatopancreatic necrosis disease outbreak in Thailand. FEMS Microbiol Lett. 2015;363:1–8.
- Dong X, Wang H, Xie G, et al. An isolate of Vibrio campbellii carrying the pirVP gene causes acute hepatopancreatic necrosis disease. Emerg Microbes Infect. 2017;6:e2.
- Liu L, Xiao J, Xia X, et al. Draft genome sequence of Vibrio owensii strain SH-14, which causes. Genome Announc. 2015;3:3354.
- Restrepo L, Bayot B, Arciniegas S, et al. PirVP genes causing AHPND identified in a new Vibrio species (Vibrio punensis) within the commensal Orientalis clade. Sci Rep. 2018;8:1–14. doi: 10.1038/s41598-018-30903-x
- Baruah K, Norouzitallab P, Phong HPPD, et al. Enhanced resistance against Vibrio harveyi infection by carvacrol and its association with the induction of heat shock protein 72 in gnotobiotic Artemia franciscana. Cell Stress Chaperones. 2017;22:377–387. doi: 10.1007/s12192-017-0775-z
- Baruah K, Huy TT, Norouzitallab P, et al. Probing the protective mechanism of poly-ß-hydroxybutyrate against vibriosis by using gnotobiotic Artemia franciscana and Vibrio campbellii as host-pathogen model. Sci Rep. 2015;5:1–8.
- Roy S, Bossier P, Norouzitallab P, et al. Trained immunity and perspectives for shrimp aquaculture. Rev Aquac. 2020. doi:10.1111/raq.12438.
- Triantaphyllidis G, Abatzopoulos T, Sorgeloos P. Review of the biogeography of the genus Artemia (Crustacea, Anostraca). J Biogeogr. 1998;25:213–226. doi: 10.1046/j.1365-2699.1998.252190.x
- Marques A, Ollevier F, Verstraete W, et al. Gnotobiotically grown aquatic animals: opportunities to investigate host-microbe interactions. J Appl Microbiol. 2006;100:903–918. doi: 10.1111/j.1365-2672.2006.02961.x
- Sung YY, Van Damme EJM, Sorgeloos P, et al. Non-lethal heat shock protects gnotobiotic Artemia franciscana larvae against virulent vibrios. Fish Shellfish Immunol. 2007;22:318–326. doi: 10.1016/j.fsi.2006.05.008
- Han B, Kaur VI, Baruah K, et al. High doses of sodium ascorbate act as a prooxidant and protect gnotobiotic brine shrimp larvae (Artemia franciscana) against Vibrio harveyi infection coinciding with heat shock protein 70 activation. Dev Comp Immunol. 2019;92:69–76. doi: 10.1016/j.dci.2018.11.007
- King AM, MacRae TH. The small heat shock protein p26 aids development of encysting Artemia embryos, prevents spontaneous diapause termination and protects against stress. PLoS One. 2012;7:e43723. doi: 10.1371/journal.pone.0043723
- King AM, Toxopeus J, Macrae TH. Functional differentiation of small heat shock proteins in diapause-destined Artemia embryos. FEBS J. 2013;280:4761–4772. doi: 10.1111/febs.12442
- Baruah K, Duy Phong HPP, Norouzitallab P, et al. The gnotobiotic brine shrimp (Artemia franciscana) model system reveals that the phenolic compound pyrogallol protects against infection through its prooxidant activity. Free Radic Biol Med. 2015;89:593–601. doi: 10.1016/j.freeradbiomed.2015.10.397
- Iryani MTM, MacRae TH, Panchakshari S, et al. Knockdown of heat shock protein 70 (Hsp70) by RNAi reduces the tolerance of Artemia franciscana nauplii to heat and bacterial infection. J Exp Mar Bio Ecol. 2017;487:106–112. doi: 10.1016/j.jembe.2016.12.004
- De Vos S. Genomic tools and sex determination in the extremophile brine shrimp Artemia franciscana [PhD thesis]. Ghent, Belgium: Ghent University; 2014.
- Roy S, Kumar V, Bossier P, et al. Phloroglucinol treatment induces transgenerational epigenetic inherited resistance against Vibrio infections and thermal stress in a brine shrimp (Artemia franciscana) model. Front Immunol. 2019;10:2745. doi: 10.3389/fimmu.2019.02745
- Lin B, Wang Z, Malanoski AP, et al. Comparative genomic analyses identify the Vibrio harveyi genome sequenced strains BAA-1116 and HY01 as Vibrio campbellii. Environ Microbiol Rep. 2010;2:81–89. doi: 10.1111/j.1758-2229.2009.00100.x
- Hoffmann M, Monday SR, Fischer M, et al. Genetic and phylogenetic evidence for misidentification of Vibrio species within the Harveyi clade. Lett Appl Microbiol. 2012;54:160–165. doi: 10.1111/j.1472-765X.2011.03183.x
- Bradford MM. A rapid and sensitive method for the quantitation of microgram quantities of protein utilizing the principle of protein-dye binding. Anal Biochem. 1976;72:248–254. doi: 10.1016/0003-2697(76)90527-3
- Kumar V, Baruah K, Nguyen DV, et al. Phloroglucinol mediated Hsp70 production in crustaceans : protection against Vibrio parahaemolyticus in Artemia franciscana and Macrobrachium rosenbergii. Front Immunol. 2018;9:1091. doi: 10.3389/fimmu.2018.01091
- Sung YY, Dhaene T, Defoirdt T, et al. Ingestion of bacteria overproducing DnaK attenuates Vibrio infection of Artemia franciscana larvae. Cell Stress Chaperones. 2009;14:603–609. doi: 10.1007/s12192-009-0112-2
- Defoirdt T, Bossier P, Sorgeloos P, et al. The impact of mutations in the quorum sensing systems of Aeromonas hydrophila, Vibrio anguillarum and Vibrio harveyi on their virulence towards gnotobiotically cultured Artemia franciscana. Environ Microbiol. 2005;7:1239–1247. doi: 10.1111/j.1462-2920.2005.00807.x
- Kumar V, Bels LD, Couck L, et al. PirABVP toxin binds to epithelial cells of the digestive tract and produce pathognomonic AHPND lesions in germ-free brine shrimp. Toxins (Basel). 2019;11:717. doi: 10.3390/toxins11120717
- Stepanovic S, Vukovic D, Bonaventura GDI, et al. Quantification of biofilm in microtiter plates: overview of testing conditions and practical recommendations for assessment of biofilm production by staphylococci. Apmis. 2007;115:891–899. doi: 10.1111/j.1600-0463.2007.apm_630.x
- Yang Q, Defoirdt T. Quorum sensing positively regulates flagellar motility in pathogenic Vibrio harveyi. Environ Microbiol. 2015;17:960–968. doi: 10.1111/1462-2920.12420
- Natrah FMI, Ruwandeepika HAD, Pawar S, et al. Regulation of virulence factors by quorum sensing in Vibrio harveyi. Vet Microbiol. 2011;154:124–129. doi: 10.1016/j.vetmic.2011.06.024
- Defoirdt T, Boon N, Bossier P, et al. Disruption of bacterial quorum sensing: an unexplored strategy to fight infections in aquaculture. Aquaculture. 2004;240:69–88. doi: 10.1016/j.aquaculture.2004.06.031
- Defoirdt T. Quorum-sensing systems as targets for antivirulence therapy. Trends Microbiol. 2018;26:313–328. doi: 10.1016/j.tim.2017.10.005
- Dong X, Wang H, Zou P, et al. Complete genome sequence of Vibrio campbellii strain 20130629003S01 isolated from shrimp with acute hepatopancreatic necrosis disease. Gut Pathog. 2017;9:1–5. doi: 10.1186/s13099-016-0151-z
- Wechprasit P, Panphloi M, Thitamadee S, et al. Complete genome sequence of Shewanella sp. strain TH2012, isolated from shrimp in a cultivation pond exhibiting early mortality syndrome. Microbiol Resour Announc. 2019;8:e01703-18. doi: 10.1128/MRA.01703-18
- Sirikharin R, Taengchaiyaphum S, Sanguanrut P, et al. Characterization and PCR detection of binary, pir-like toxins from vibrio parahaemolyticus isolates that cause acute hepatopancreatic necrosis disease (AHPND) in shrimp. PLoS One. 2015;10:1–16. doi: 10.1371/journal.pone.0126987
- Choi M, Stevens AM, Smith SA, et al. Strain and dose infectivity of Vibrio parahaemolyticus: the causative agent of early mortality syndrome in shrimp. Aquac Res. 2017;48:3719–3727. doi: 10.1111/are.13197
- Kumar V, Wille M, Lourenço TM, et al. Biofloc-Based enhanced survival of litopenaeus vannamei upon AHPND-causing Vibrio parahaemolyticus challenge is partially mediated by reduced expression of its virulence genes. Front Microbiol. 2020;11. doi:10.3389/fmicb.2020.01270.
- Norouzitallab P, Baruah K, Vanrompay D, et al. Teaching shrimps self-defense to fight infections. Trends Biotechnol. 2019;37:16–19. doi: 10.1016/j.tibtech.2018.05.007
- Fuqua WC, Winans SC, Greenberg EP. Quorum sensing in bacteria: the luxR-luxI family of cell density- responsive transcriptional regulators. J Bacteriol. 1994;176:269–275. doi: 10.1128/JB.176.2.269-275.1994
- Xu L, Li H, Vuong C, et al. Role of the luxS quorum-sensing system in biofilm formation and virulence of staphylococcus epidermidis. Infect Immun. 2006;74:488–496. doi: 10.1128/IAI.74.1.488-496.2006
- Bassler BL, Greenberg EP, Stevens AM. Cross-species induction of luminescence in the quorum-sensing bacterium Vibrio harveyi. J Bacteriol. 1997;179:4043–4045. doi: 10.1128/JB.179.12.4043-4045.1997
- Miller MB, Bassler BL. Quorum sensing in bacteria. Annu Rev Microbiol. 2001;55:165–199. doi: 10.1146/annurev.micro.55.1.165
- Schauder S, Bassler BL. The langauges of bacteria. Genes Dev. 2001;15:1468–1480. doi: 10.1101/gad.899601
- Henke JM, Bassler BL. Three parallel quorum-sensing systems regulate gene expression in Vibrio harveyi. J Bacteriol. 2004;186:6902–6914. doi: 10.1128/JB.186.20.6902-6914.2004
- Bassler BL, Wright M, Silverman MR. Multiple signalling systems controlling expression of luminescence in Vibrio harveyi: sequence and function of genes encoding a second sensory pathway. Mol Microbiol. 1994;13:273–286. doi: 10.1111/j.1365-2958.1994.tb00422.x
- Srikanth C V, McCormick BA. Interactions of the intestinal epithelium with the pathogen and the indigenous microbiota: a three-way crosstalk. Interdiscip Perspect Infect Dis. 2008;2008:1–14. doi: 10.1155/2008/626827
- Almagro-Moreno S, Pruss K, Taylor RK. Intestinal colonization dynamics of Vibrio cholerae. PLoS Pathog. 2015;11:1–11. doi: 10.1371/journal.ppat.1004787
- Quintana-Hayashi M, Padra M, Padra J, et al. Mucus-pathogen interactions in the gastrointestinal tract of farmed animals. Microorganisms. 2018;6:55. doi: 10.3390/microorganisms6020055
- Hong X, Lu L, Xu D. Progress in research on acute hepatopancreatic necrosis disease (AHPND). Aquac Int. 2016;24:577–593. doi: 10.1007/s10499-015-9948-x
- Lin SJ, Chen YF, Hsu KC, et al. Structural insights to the heterotetrameric interaction between the vibrio parahaemolyticus pirAvp and pirBvp toxins and activation of the cry-like pore-forming domain. Toxins (Basel). 2019;11, doi:10.3390/toxins11040233.
- Dharmani P, Srivastava V, Kissoon-Singh V, et al. Role of intestinal mucins in innate host defense mechanisms against pathogens. J Innate Immun. 2009;1:123–135. doi: 10.1159/000163037
- Campa-Córdova AI, León-Gallo AF, Romero-Maldonado A, et al. Recombinant PirA-like toxin protects shrimp against challenge with Vibrio parahaemolyticus, the aetiological agent of acute hepatopancreatic necrosis disease. J Fish Dis. 2017;40:1725–1729. doi: 10.1111/jfd.12625
- Reyes-Becerril M, Maldonado-García M, Guluarte C, et al. Evaluation of ToxA and Vibrio parahaemolyticus lysate on humoral immune response and immune-related genes in Pacific red snapper. Fish Shellfish Immunol. 2016;56:310–321. doi: 10.1016/j.fsi.2016.07.014
- Reyes-Becerril M, Guluarte C, Ceballos-Francisco D, et al. Enhancing gilthead seabream immune status and protection against bacterial challenge by means of antigens derived from Vibrio parahaemolyticus. Fish Shellfish Immunol. 2017;60:205–218. doi: 10.1016/j.fsi.2016.11.053
- Rekecki A, Ringø E, Olsen R, et al. Luminal uptake of Vibrio (Listonella) anguillarum by shed enterocytes - a novel early defence strategy in larval fish. J Fish Dis. 2013;36:419–426. doi: 10.1111/jfd.12009
- Rekecki A, Gunasekara RAYSA, Dierckens K, et al. Bacterial host interaction of GFP-labelled Vibrio anguillarum HI-610 with gnotobiotic sea bass, Dicentrarchus labrax (L.), larvae. J Fish Dis. 2012;35:265–273. doi: 10.1111/j.1365-2761.2011.01342.x