ABSTRACT
Colistin is regarded as a last-resort agent to combat infections caused by multidrug-resistant (MDR) Gram-negative bacteria, especially carbapenem-resistant isolates. In recent years, reports of colistin-resistant Klebsiella pneumoniae (CoRKp) are increasing. However, the molecular mechanism and relevance of colistin resistance and virulence remain unclear. Fourteen CoRKp strains were retrospectively screened from 1884 clinical K. pneumoniae isolates during 2017–2018 in China. Six CoRKp strains belonging to ST11 were MDR strains. Plasmid-mediated mobile colistin-resistance genes had a low prevalence in CoRKp. Our results revealed that up-regulated expression of two-component systems, especially phoPQ, contributed more to colistin resistance. mgrB mutation was the most common molecular mechanism of colistin resistance, caused by either nonsense mutations or insertion sequences, which drove the overexpression of phoPQ system. This study also identified three novel point mutations in pmrAB system, in which D313N mutation in pmrB was proved to increase the MIC to colistin by 16-fold. In addition, 6 out of 14 CoRKP strains independently carried hypervirulence genes. All six strains showed medium-to-high virulence phenotype compared with NTUH-K2044 strain in mice intraperitoneal challenge models. We found that 4 strains were biofilm strong producers and transcriptome analysis revealed that three of them significantly up-regulated expression of type III fimbrial shaft gene mrkA. In conclusion, our result revealed the emergence of colistin-resistant and hypervirulent MDR K. pneumoniae, which is a noticeable superbug and could cause a severe challenge to public health.
Introduction
Colistin was a human medication to treat clinical infections before the 1950s, while it was limited to be used only in agriculture and graziery due to its renal toxicity and neurotoxicity [Citation1]. Recently, with the emergence of multidrug-resistant (MDR) gram-negative bacteria, particularly carbapenem-resistant Enterobacteriaceae (CRE) all over the world, colistin returned to the clinical field as “the last option” to treat carbapenem-resistant Klebsiella pneumoniae (CRKP) and other MDR pathogenic bacterial infections [Citation2]. At the same time, reports of colistin-resistant Klebsiella pneumoniae (CoRKp) have been increasing, raising a significant challenge to healthcare [Citation1,Citation3,Citation4].
In K. pneumoniae, lipopolysaccharide (LPS) modification leads to the reduction of LPS negative charge, which reduces its affinity to colistin, resulting in colistin resistance [Citation5]. Plasmid-mediated mobile-resistance genes (mcr-1 to mcr-10) and chromosome-mediated regulation pathways are two main mechanisms of LPS modification [Citation5,Citation6]. The MCR protein, a phosphoethanolamine (PEtN) transferase enzyme encoded by mcr gene, could catalyze the addition of PEtN to lipid A and result in a more cationic LPS [Citation7]. Meanwhile, mutations of genes in pmrAB and phoPQ two-component systems (TCS), or their regulators (such as mgrB) could increase the addition of 4-amino-4-deoxy-l-arabinose (l-Ara4N) and PEtN to lipid A in LPS, and consequently elevate the MICs [Citation8].
Hypervirulent K. pneumoniae (hvKp) was usually reported as a life-threatening pathogen. The sequence and serotype of hvKp were often reported as ST23-K1 and ST86/65-K2. Compared with the classic K pneumoniae, hvKp was usually less relevant with resistance [Citation9]. As the most prevalent MDR lineage in Asia [Citation10], K. pneumoniae of ST11 was first reported to become a hypervirulent strain in China [Citation11]. There have been increasing reports of MDR K. pneumoniae with virulence genes and related fatal hospital outbreaks [Citation11–13], indicating the harm of MDR hvKp.
In this study, we retrospectively identified 14 CoRKp strains from 1884 non-repetitive clinical K. pneumoniae isolates in China during 2017–2018 Study for Monitoring Antimicrobial Resistance Trends (SMART) surveillance. Molecular and genetic analysis revealed that chromosome-mediated resistance was the predominant mechanism of CoRKp. Thus, we focused on investigating the impacts of transcription and genetic variants of genes involved in TCSs and their regulators mentioned above on colistin resistance. In addition, our results revealed the cooccurrence of colistin resistance and hypervirulence in K. pneumoniae, especially in ST11 lineage. These findings provide evidence that high-level colistin-resistant MDR K. pneumoniae could become hypervirulent and hint at the importance of monitoring colistin-resistance mechanism and virulence factors of CoRKp.
Materials and methods
Stains and antimicrobial susceptibility testing
From 2017 to 2018, 1884 non-repetitive K. pneumoniae strains from patients of 22 multi-centre tertiary class-A hospitals in China were collected in the SMART surveillance project [Citation14]. All isolates were sent to Peking Union Medical College Hospital for re-identification by using Vitek MALDI-TOF MS (BioMérieux, France). The minimum inhibitory concentrations (MICs) of polymyxin B and colistin were determined by broth microdilution (BMD) which is the gold-standard reference method according to the Clinical and Laboratory Standards Institute (CLSI) M100-ED30 [Citation15]. Considering that the standard has replaced the resistance breakpoints with intermediate, we used the Antimicrobial Susceptibility Testing Breakpoint v10.0 [Citation16] made by the European Committee to facilitate calculation. The polymyxin B and colistin MICs for K. pneumoniae were interpreted in this manner: susceptible ≤ 2 mg/L, resistant > 2 mg/L, with Escherichia coli ATCC 25922, Pseudomonas aeruginosa ATCC 27853 and colistin-resistant E. coli NCTC 13846 (mcr-1 positive) as quality control strains. Antimicrobial susceptibility testing for other drugs was performed by BD PhoenixTM M50 (Becton Dickson Diagnostics, USA).
Whole-genome sequencing and assembly of colistin-resistant isolates
We used TIANamp Bacteria DNA Kit (DP302) to extract genomic DNA from colistin-resistant isolates, which were inoculated in LB medium (Sangon Biotech, China), and cultured at 37°C for 18 h. Then we quantified and qualified the yield of extracted DNA by NanoDrop 2000 and Qubit 2.0 Fluorometer. Whole-genome sequencing was performed on Illumina Novaseq6000 (Illumina Inc, USA) with 150-bp paired-end library. The raw sequencing reads were trimmed by Trimmomatic v0.38 [Citation17] and then assembled de novo using Shovill v1.0.1 (https://github.com/tseemann/shovill) with a minimum contig length of 200.
Multi-locus sequence typing (MLST) and O and K antiserum typing (serotypes)
The assembled genomes were used for in silico multi-locus sequence typing (MLST) and O and K antiserum typing. MLST analysis was conducted using MLST software (https://github.com/tseemann/mlst) with contig files compared against traditional PubMLST typing schemes [Citation18]. Meanwhile, O and K serotypes were determined by Serotype Finder v2.0.1 (https://cge.cbs.dtu.dk/services/SerotypeFinder/), based on sequence alignment against its public database v1.0.0 [Citation19].
SNP calling and phylogeny reconstruction
A total of 46 genomes were used in the phylogeny reconstruction, including 14 newly sequenced genomes of colistin-resistant isolates, and 32 public sequences downloaded from NCBI, of which 31 genomes were selected from major clones of K. pneumoniae population, referred in Wyres et al. [Citation20]. The public genome of NTUH-K2044 (Accession: NC_012731.1) was used as a reference.
All genomes were aligned against the reference genome using MUMmer (v3.0) [Citation21] to generate whole-genome alignments and to identify single-nucleotide polymorphisms (SNPs) in the core genome, with repetitive regions removed. In total, 126,058 SNPs were identified in these 46 genomes. Based on the concatenated SNPs, a maximum-likelihood tree with 1000 fast bootstrap replicates was inferred using IQ-TREE under GTR + G4 substitution model [Citation22].
Identification of antibiotic-resistance genes
ResFinder v4.0 [Citation24]; and PointFinder [Citation23] were used to identify antibiotic-resistance genes or mutations with a minimum coverage of 80% and a minimum identity of 80%. As reported, variations located in coding regions and upstream intergenic regions of the TCS and transferase genes including pmrABCK, phoPQ, crrAB, and its negative regulator mgrB could be related with chromosome-mediated colistin resistance through its effects on transcription. We recalled SNPs for these genes and identified insertion sequences involved in mgrB gene based on BLAST alignment against the reference genome of K. pneumoniae NTUH-K2044. K. pneumoniae MGH 78578 (Accession no. NC_009648.1) was also used as a reference, due to that crrAB genes were accessory genes and absent in NTUH-K2044. Nonsynonymous and nonsense substitutions and insertion mutations were selected for further analysis. Meanwhile, plasmid-mediated mobile colistin-resistance genes (mcr-1 to mcr-10) were screened by both multiplex PCR and genome comparison [Citation24–27]. Specific mcr typing was finally determined by aligning assemblies against different mcr-1 to mcr-10 gene sequences in the public database of NCBI AMRFinderPlus [Citation28] using blastn v2.2.28+.
Chromosomal regulon gene expression analysis using real-time quantitative PCR (RT-qPCR)
The expression of chromosome-mediated colistin-resistant genes was detected to explore the relationship between regulon and resistance. A total of 41 K. pneumoniae isolates were divided into the colistin-resistant group (COL-R) and colistin-susceptible group (COL-S) based on their colistin MICs. Briefly, all isolates were grown to the logarithmic phase, and then their total RNA was extracted using RNA pure Bacteria Kit (DNase I) (CoWin Biosciences, China). Reverse transcription was performed using FastKing RT Kit (With gDNase) (TIAGEN, China) to produce cDNA from 1500 ng of total RNA for each isolate. Finally, the gene expression data were obtained by RT-qPCR using LightCycler 480 II (Roche, Switzerland) and TB Green Premix DimerEraserTM (TaKaRa, Japan). The housekeeping gene rpoB was used as the internal reference. Each experiment was repeated three times, and the relative expression of each gene in different strains was calculated, denoted by 2−ΔΔCt.
Functional confirmation of pmrA and pmrB novel point mutation
The construction of point mutation was performed in K. pneumoniae SZS128 strain (the MIC of colistin was 0.5 mg/L and harboured wide-type TCS genes) using CRISPR-Cas9-mediated genome-editing method containing pCasKP-apr and pSGKP-spe plasmid [Citation29]. Mutations of G611 T in pmrA gene, A853 T and G937A in pmrB gene were constructed through two-step genome-editing procedure. Firstly, deleting ∼400 bp sequences around the point mutation by co-transforming a linear donor sequence and the spacer-introduced pSGKP-spe plasmid, individually, into the l-arabinose-induced pCasKP-apr-harbouring cells to partially delete the target sequence. After gene partial deletion, the pSGKp plasmid was cured by culturing in the LB agar plate containing 50 mg/L apramycin and 5% sucrose at 30°C. Secondly, the sequence containing point mutation was complemented into the cells using pSGKP whose spacer was located in the interfaces of upstream and downstream sequences of deleted sequences in the first step. The MICs of colistin were evaluated in the colony in which successful point mutation was confirmed by both PCR and sequencing after pCasKP and pSGKP plasmid curing. Preparation of competent cells, electroporation and spacer cloning into pSGKP plasmid were performed as Wang et al. [Citation29] described. The primers used in this study were listed in Sup Table 2. BMD was used to detect the MIC of colistin against transformants.
Identification of virulence genes
The virulence gene profiles of the 14 colistin-resistant strains were characterized through sequence alignment against the VFDB database with a minimum coverage of 80% and a minimum identity of 80% [Citation30]. Meanwhile, the 14 genomes were also aligned against pLVPK plasmid genome (Accession: NC_005249.1) using blastn v2.2.28 + to detect the presence and absence of hvKp-related genes, including rmpA, rmpA2, iucABCD, iutA, iroBCDN, and peg-344, and to determine whether the isolates were hvKp or not. Finally, BRIG v0.95 software was used to visually display the plasmid genome comparison results, including six identified hvKp isolates, along with the plasmid genome of NTUH-K2044, and the reference pLVPK.
Mouse intraperitoneal infection models
The animal protocols were reviewed and approved by the Animal Ethics Committee and Administration Institutional Animal Care Committee of Tsinghua University. All experiments were carried out in Tsinghua University Animal Biosafety Level 2 (ABSL-2) under the guidelines of the “Ethics of Animal Experimentation Statement.”
ICR female mice at the age of six orseven weeks were obtained from Vitalriver. Bacteria were grown in LB broth until logarithmic phase and stored in −80°C. Mice intraperitoneal (IP) infection models were modified from the previously published studies [Citation31,Citation32] to identify the MDR hypervirulence K. pneumoniae. NTUH-K2044 was used as a hypervirulent control strain, and QD110, a strain confirmed by whole-genome sequencing that did not contain any virulence genes, as a low-virulence control strain. Five mice were injected with 5×107 CFU in a group of NTUH-K2044 and other experimental strains, or with approximately 1×108 CFU of QD110, respectively. Then the mortality of mice was observed up to seven days.
Biofilm formation
In order to reveal biofilm formation in CoRKp, we used crystal violet to analyze the biofilm-production capacity. Biofilm formation protocol was modified from the previous protocol [Citation33] by using 1% crystal violet to dye the biofilm, and 96% ethanol to solubilize the dyestuff and then determined optical density at 590 nm. Three biological replicates were performed to ensure that all results were valid.
Biofilm formation capabilities were evaluated according to a previous report [Citation34]. Three standard deviations above the mean OD of the negative control were regarded as the OD cut-off (ODc). Biofilm formation capabilities were classified into the following categories: non-biofilm capabilities (OD ≤ ODc), weak biofilm capabilities (ODc < OD ≤ 2ODc), moderate biofilm capabilities (2ODc < OD ≤ 4ODc), and strong biofilm capabilities (4ODc < OD).
Transcriptome and data analysis
Total RNA was extracted using RNA pure Bacteria Kit (DNase I) (CoWin Biosciences, China) from three biofilm-positive strains, and one colistin-susceptible and weak biofilm strain, ATCC13883, which was used as control. Purification was performed under the manufacturer’s instructions. Each strain had three replicates. RNA-seq was performed on Illumina Novaseq6000 (Illumina Inc, USA) with 150-bp paired-end library. After trimming raw reads using Trimmomatic software, we aligned the reads to the reference genome of NTUH-K2044. Then, HTSeq (v0.6.1) [Citation35] was used to annotate and count aligned reads for each gene. Differentially expressed genes (DEGs) were identified using DESeq2 [Citation36] with thresholds of absolute log2 fold change >1 and Benjamini–Hochberg adjusted P-value (p. adjust) < 0.05. DEGs were displayed in volcano plot using the R package ggrepel. Finally, we used clusterProfiler [Citation37] to analyze gene clusters for up-regulated and down-regulated DEGs in Kyoto Encyclopedia of Genes and Genomes (KEGG) pathways.
Statistical analysis
Linear correlation between transcription level of chromosomal regulators and colistin MICs was analyzed in GraphPad Prism 8. Mann–Whitney U-test Spearman’s correlation and multiple linear regression were performed by SPSS v25.0. Mantel–Haenszel χ2-test was used to test association between virulence and MICs. Log-rank test (Mantel–Cox) was used to analyze the survival curve of mice infection models in GraphPad Prism 8. P-value <0.05 was regarded to be statistically significant, and P < 0.001 was extremely significant.
Results
Prevalence of colistin-resistant K. pneumoniae during 2017–2018 surveillance
A total of 1884 non-repetitive clinical K. pneumoniae were collected during 2017–2018 SMART surveillance survey with 22 hospitals from seven regions (north, 8.7%; northeast:16%; central, 8.2%; east non-Jiangzhe, 20.7%; east Jiangzhe, 20.1%; south: 9.4%; southwest, 16.9%) in China. The proportion of clinical isolates from intra-abdominal tract, respiratory tract, urinary tract, and blood were 24.2%, 45.0%, 17.1%, and 13.7%, respectively. Medical intensive care units (ICUs) collected 20.6% isolates, including general unspecified ICU (7.5%), surgery ICU (6.1%), medicine ICU (5.1%), and pediatric ICU (2.0%). The age of patients ranged from 0 to 101 years, > 60 years accounting for 54.8%, 31–60 years 38.5%, and < 30 years 6.8%.
Antimicrobial susceptibility testing was reperformed for 14 CoRKp strains. The prevalence of CoRKp was 0.74% (n = 14/1884). These strains were collected from seven provinces, five of them isolated from Zhejiang Province (see ). The colistin MICs range of CoRKp was 4 to > 64 mg/L with a wide variety of specimen types including sputum (28.6%), bile (21.4%), blood (14.3%), and other sterile body fluids (see ).
Table 1. Antimicrobial susceptibility profiles, isolates information and biofilm-production ability of colistin-resistance K. pneumoniae.
Antimicrobial susceptibility testing results also revealed that 64% (9/14) of the strains were resistant to cephalosporins (see ), and 50% (7/14) resistant to carbapenem, carrying blaKPC-2 gene. Additionally, the tigecycline-resistance gene tmexCD1-toprJ1 was identified in a tigecycline-intermediate isolate (see Sup. Figure 1 and ).
Notably, 13 of 14 strains were MDR and four of them were extensively drug-resistant (XDR) isolates. One ST11 strain, isolated from the blood of a 69-year-old male in Zhejiang Province, was pan-drug-resistant (PDR), except for an intermediate resistance to chloramphenicol (see ).
Phylogenetic analysis of CoRKp and prevalence of mcr genes
The phylogeny of newly sequenced colistin-resistant isolates was generated, together with representative strains of global problem clones of K. pneumoniae referred in Wyres et al. [Citation20]. Phylogenetic analysis indicated that the 14 CoRKp strains had an extensive genetic diversity as they were assigned to eight lineages (see ). ST11 was the dominant sequence type of CoRKp (see ), with six genomes involved, of which four strains were isolated from Zhejiang Province. In addition, only one hypervirulent clone strain was CG86, and the rest were classified as ST15, ST111, ST395, ST2459, ST3812, and ST5253 scattering across the phylogenetic tree.
Figure 1. K. pneumoniae population structure and the prevalence of mcr gene and hvKp marker genes. The phylogeny in the left represents the population structure of 14 colistin-resistant isolated and 31 representative genomes of major global problem clones, with six hypervirulent clones (CG23, CG25, CG65, CG66, CG86, CG380) highlighted in blue branches, and 14 colistin-resistant isolated coloured in red text. Bootstrap values are also labelled on the nodes of the tree. The prevalence of mcr gene and hvKp marker genes are shown in the heatmap with whitespace separated. Different colors of the square in the heatmap represent the alignment identity for each gene (see the legend in the lower right section), with grey colour represents gene absence.
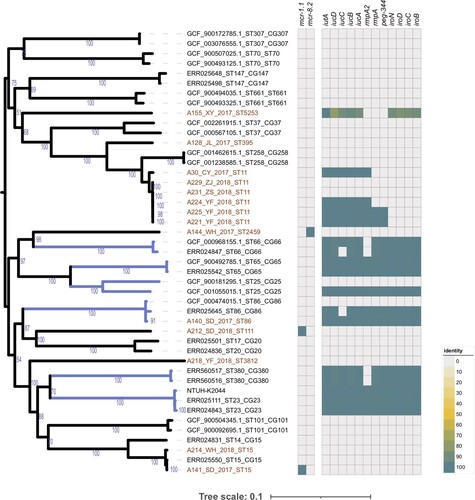
Only three isolates carried mobile colistin-resistance genes, which indicated that 21% (n = 3/14) of CoRKp isolates were mcr-positive. Sequence alignment showed that two isolates, A212 (ST111) and A141 (ST15), sampled from the same hospital of Shandong Province, harbouring mcr-1.1 gene. A144 (ST2459) from Hubei Province, contained mcr-8.2 gene (see and ). All three mcr-carrying isolates were colistin-resistant, with MICs of 4, 8, and 16 mg/L, respectively (see ). The promoter sequences of mcr-1.1 and mcr-8.2 genes were also compared in these three sains, which could affect the expression of mcr gene and eventually result in various colistin MICs. Our results revealed variations between mcr-1.1 and mcr-8.2 promoters. However, the two mcr-1.1 carrying strains with different MICs had identical genetic contexts (see Sup. Figure 4).
Molecular mechanism of chromosome-mediated colistin resistance
As only 3 CoRKp strains harboured plasmid-mediated colistin-resistance genes, we further studied the relationship among mutation, transcription, and colistin resistance based on pmrA, pmrC, pmrK, phoP, phoQ, and mgrB. Significant differences were found between resistant and susceptible groups in pmrA, phoQ, and phoP (Mann–Whitney U-test, P <0.05) (see ). The expressions of pmrA, phoP, and phoQ in all colistin-resistant strains were 3.23-fold, 9.64-fold, and 8.82-fold higher than colistin-susceptible strains. The results were also supported by Spearman’ correlation test, as there was high correlation coefficient between MICs and the expression of phoP (0.593, P < 0.05) and phoQ (0.766, P < 0.0001) (see ). We used multiple linear regression to analyze the relationship between the transcriptional level of TCS and MICs, and obtained a formula, MIC = 4.121× pmrA + 3.501× phoQ-0.34 (P < 0.001). The influence coefficient of TCS on MICs was 0.691 (P < 0.0001). Moreover, we found that the transcriptional level of phoQ was positively correlated with pmrA (R2 = 0.250) and phoP (R2 = 0.541). Although there was no significant linear trend between mgrB and phoP, it still exhibited a negative correlation (see Sup Figure 5B). ST11 strains had no expression of mgrB gene except A231 expressed a low level. However, their expressions of phoQ were all elevated, which reflected the negative correlation between mgrB and phoQ in turn.
Figure 2. Relative expression of the pmrA, phoP, phoQ, mgrB, pmrC, and pmrK genes in colistin-resistant group and colistin-susceptible group. Values were represented by means of three independent replicates. Relative expression of pmrA, phoP, and phoQ in colistin-resistant group was significantly higher than colistin-susceptible group. There were 6 strains that less expressed mgrB gene, five strains harboured modified-mgrB gene and the rest strain were WT. However, because of early termination, overexpression of mgrB in one strain from ST11 could not form a protein with a negative regulatory function. *, P < 0.05; ns, not significantly different.
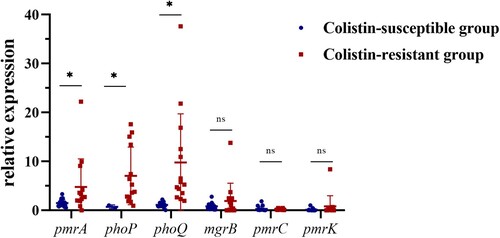
Table 2. Correlation coefficient between MIC and Chromosomal regulon gene expression.
Alteration in genome sequence is an important factor of transcription, which could determine the level of gene expression. Thus, we analyzed mutations of the genes responsible for colistin resistance. Compared with the reference genome of NTUH-K2044, 14 CoRKp strains presented no variation in phoP gene (named wild-type (WT)), while they all harboured D150G amino substitution in phoQ gene. In addition, six strains carried modified-mgrB containing Q30stop mutation and insert mutations mediated by ISKpn26 and other IS elements was detected (see and ). M27 K mutation in mgrB has been reported to confer colistin resistance, which did not affect the expression of mgrB in our study. Additional mutations were identified in core genes of pmrABCDK and accessory genes crrAB.
Figure 3. Transcriptional level and substitution mutations of chromosomal regulators in colistin-resistant isolates. The top six bar plots show the transcriptional level of six chromosomal regulators, with the x-axis represents 14 colistin-resistant isolates, ordered according to their colistin MICs, see the colour scale under the bar plot of pmrK. The prevalence of substitution mutations identified in nine chromosomal regulators are displayed in the heatmap following the colistin MICs. Each row shows a mutation site of corresponding gene (see the rightmost text), with mutated isolated colored in purple and those with no corresponding variation in grey. Genes absent in isolates are colored in white. Mutation reported in previous studies are marked with * in the rightmost. Three newly identified mutations which we speculated might have relation to colistin resistance are highlighted in red text.
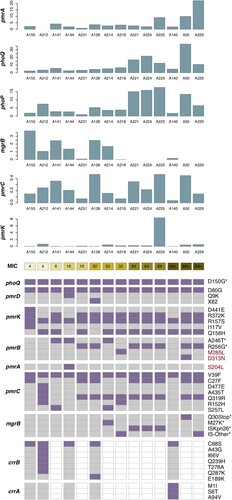
Table 3. Colistin-resistant genes mediated by plasmid and mutations in the chromosomal genes in K. pneumoniae.
Functional confirmation of pmrA and pmrB novel point mutation
Except for two known mutations, R256G and A246 T in pmrB gene, we suspected that three novel variations, containing M285L and D313N in pmrB and S204L in pmrA, might contribute to colistin resistance (see ). PCR and sequencing confirmed site-specific mutagenesis of amino acid substitution in pmrAB. The amino substitution D313N in pmrB in SZS128 elevated the MIC to 8 mg/L which was 16-fold higher than the original strain. However, M285L in pmrB and S204L in pmrA did not increase MICs of colistin against the original strain (see Sup Table 3).
Identification of hvKp among colistin-resistant clinical strains
Whole-genome sequencing was used to reveal the hypervirulent plasmid in CoRKp. The pLVPK-like virulence plasmid identified in 6 CoRKp strains harbouring the hypervirulent biomarker genes (peg344, iroB, iucA, rmpA, and rmpA2) were from ST11, ST86, and ST5253 strains (see and ). The strain, A140, from ST86-K2 harbouring all five hypervirulent biomarkers showed the highest virulence potential. Three strains from ST11-K64 and one from ST11-K47 carrying hypervirulent genes showed middle-virulence between NTUH-K2044 and low-virulent control QD110 (see ). The strains, A221, and A225, carried iroB, iucA, rmpA, and rmpA2 while A224 only carried iroB and iucA and A30 from K47 only carried iucA (see and ). The hvKp from ST5253-K28 possessing iucA and iroB showed low virulence in mice IP model. Compared with related gene sequences in representative hvKp plasmid, the alignment identity of ST5253-K28 was among 78%−93%, which suggested a horizontal gene transcription event from other species (see and Sup Figure 2). A231 and A229 from ST11 strains lacked the hvKp marker genes mentioned above (see Sup Figure 3), while the virulent phenotype of these two strains was the same as strains from ST11 harboured hypervirulent genes (see Sup Figure 3 and Figure 4). Notably, ybt gene which encodes yersiniabactin was present in all six MDR strains in ST11 lineage. The strain from ST111-K63 harbouring mcr-1.1 could lead to mice’s death in IP model in 24 h. The rest five isolates showed low virulence in mice IP model. Other virulence factors identified in the 14 colistin-resistant isolates were shown in Sup Figure 3. A significant positive correlation could be found in CoRKp (P < 0.0001) by using Mantel–Haenszel χ2-test to reveal the association between virulence and MICs.
Figure 4. Survival curve of six weeks CD1 mice after IP infection with colistin-resistance K. pneumoniae. Each group, with five mice included with one K. pneumoniae strain, either 5×107 CFU of 14 CoRKp strains or NTUH-K2044, or 1×108 CFU of low-virulent control QD110. Strain of ST86-K2 presented the highest level of virulence, more virulent than the reference strain NTUH-K2044, and six strains from ST11-K64/K47 showed intermediate virulence, except for ST111-K63 isolate, which showed weak virulenceto some extent. Isolate from ST5253-K28 was classic virulence. Data of classic-virulent strains from, with no death of mice occurring within 7 days, were not shown, as the survival consistent with QD110. P-values from the log-rank (Mantel-Cox) test were indicated as follows: *, P < 0.05; **, P <0.01; ns, not significantly different.
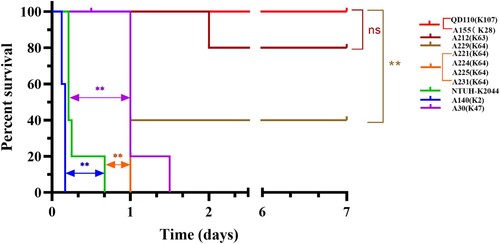
Biofilm-forming capacity in colistin-resistant strains
The mean OD590 nm values obtained by the quantitative biofilm-production assay were itemized in . Four strains were strong biofilm producers. Five strains showed moderate biofilm-forming capacities. Two strains were weak biofilm producers and the rest two strains showed non-biofilm capabilities. We regarded strains with colistin MIC ≥8 mg/L as high MIC group, while others with MIC <8 mg/L as low MIC group. The results showed that high MIC group of CoRKp showed lower biofilm-forming capacity (0.6647 ± 0.12494) than low MIC group (1.1673 ± 0.86826).
Transcriptome analysis of strong biofilm producers of CoRKp
In order to investigate DEGs of strong biofilm producers isolates, we selected three isolates with strong biofilm-forming ability and a weak biofilm producer strain, ATCC13883, for RNA-seq analysis. A total of 623 DEGs (with absolute log2 fold change >1 and p. adjust < 0.05) were identified, including 416 up-regulated genes and 207 down-regulated genes (A and Supplementary Table 1). The most significant up-regulated gene mrkA whose log2fold change was 8.64, encodes type III fimbrial shaft MrkA, which could facilitate biofilm formation [Citation37]. Meanwhile, for down-regulated genes, asnA encoding aspartate-ammonia ligasem and KP1_RS01530 encoding lytic transglycosylase F, was differentially expressed. The up-regulation of asnA was reported to be involved in biofilm formation of Streptococcus pneumoniae [Citation39]. For biological functions of these differently expressed genes, 416 up-regulated genes were enriched in 4 KEGG pathways, i.e. starch and sucrose metabolism, phosphotransferase system (PTS), butanoate metabolism and selenocompound metabolism (B), while the 207 down-regulated genes were enriched in 9 KEGG pathways, including the most enriched pathways of microbial metabolism in diverse environments, ribosome, and carbon metabolism (C).
Discussion
Colistin could be used to cure the infection caused by MDR K. pneumoniae, but the misuse of colistin, in turn, has caused a prevalence of colistin resistance (CHINET data, 2017–2020. http://www.chinets.com/Data/AntibioticDrugFast). The 14 colistin-resistant isolates were assigned to various lineage, which indicates that the CoRKp has a wide genetic diversity and geographic distribution. ST11 was the dominant sequence type of CoRKp. The MDR percentage of CoRKp strains in our study was 92.8%, which will bring difficulties to further clinical treatment. One PDR strain was determined from ST11 isolated from blood, which highlights the increasing multidrug resistance in clinical K. pneumoniae isolates in China. These findings suggested that colistin-resistant K. pneumoniae has become a serious public problem.
In addition, only three of the 14 colistin-resistant isolates carried mobile colistin-resistance gene, mcr-1.1 or mcr-8.2, with different colistin MICs. The mcr prevalence in colistin-resistant K. pneumoniae (21%) was significantly lower than that in E. coli (87.5%, data unpublished). Unlike the first reported mcr-8.2-bearing strain in ST395 isolated from animal [Citation40], the first mcr-8.2-positive clinical isolate in our study was ST2459, a novel K. pneumoniae ST submitted by our study.
Compared with plasmid-mediated colistin resistance by mcr, the overexpression of TCSs regulator genes played a more important role in resistance. Our results speculated that phoPQ regulatory factors contributed most to chromosome-mediated colistin resistance in K. pneumoniae because of correlation. Previous reports revealed that the various overexpression of TCSs such as pmrAB [Citation41], phoPQ [Citation41], pmrC [Citation42], and pmrK [Citation42,Citation43] might lead to colistin resistance, but it is still unclear which regulator is the most important one. Besides, multiple linear regression equation was first proposed in our study, which could intuitively reflect that pmrA and phoQ played important roles in colistin resistance. Whereas, the formula still needs clinical isolates with various MICs to verify. In conclusion, the chromosome-mediated resistance to colistin in K. pneumoniae was mainly caused by up-regulated expression phoPQ system, which was proved to be a key element affecting MICs.
Several mutations in chromosomal regulators were detected by the whole-genome analysis . Modified-mgrB, including insertion of diverse IS fragments and amino acid substitution are now primary colistin-resistance mechanisms in K. pneumoniae [Citation44–46]. In addition, amino acid substitutions, such as T157P in pmrB [Citation47], D191Y in phoP [Citation48] and L26P in phoQ [Citation49] were reported to increase MICs by 24–1000 folds among clinical isolates, while D150G in phoQ, a common mutation in CoRKp, could not impact colistin resistance [Citation49]. In this study, 3 unreported substitutions were found. As these mutations had correlation of expression of pmrA, we speculated that they might contribute to colistin resistance. D313N in pmrB was verified as an available mutation in colistin resistance. The rest of unreported mutations in pmrA and pmrB did not contribute to resistance. D313N and M285L were located on catalytic and ATP-binding (CA) domain in pmrB. M285L was predicted as a neutral mutation by PROVEAN (http://provean.jcvi.org/index.php). D313N was predicted as a deleterious mutation that was speculated to enhance the ATP-binding capability [Citation50], thus could increase the MIC of colistin. A recent study reported that a missense mutation in crrB contributed to colistin resistance [Citation51]. However, it was an accessory genome and was not detected in all colistin-resistant strains [Citation44]. Thus, the expression of crrAB system was not taken into consideration in our study. According to our results, we speculated that colistin resistance in K. pneumoniae results from a combination of multiple mutations, although the molecular mechanisms of some amino substitutions are still unknown. Further investigations are needed to confirm the relationship between these mutations and colistin resistance.
Consistent with previous researches, we found a cluster of colistin- and carbapenem-resistant K. pneumoniae (CoR-CRKp) strains in ST11 lineage and most of them were hvKp as well [Citation52]. Premature stop mutation and insertion sequence (mainly ISKpn26) in mgrB were the main cause of colistin resistance. Importantly, previous study characterized ISKpn72 element in a mobile plasmid and chromosomal gene, which suggested chromosomal colistin-resistant mechanism could be transmitted by plasmid [Citation53]. In further investigations, we should focus on whether all types of insertion sequences could lead to the transmission of IS elements to chromosomes. Besides, it is reported that phoPQ system and mgrB could elevate virulence. mgrB inactivation of K. pneumoniae increases virulence in Galleria mellonella infection model [Citation54], and deletion of phoPQ could decrease invasion ability in Shigella flexner [Citation55]. We used hypervirulent biomarkers to identify the hvKp, and 83.3% hv-CoRKp showed virulence in mice IP model. Instead, ybt seems not to play a particular role because of the existence in all ST11 isolates. Brazil reported a ST11-K64 K. pneumoniae fatal bacteremia while isolates without hypervirulent biomarkers [Citation56], as our two ST11-K64 CoRKp (A229 and A231) also showed virulent phenotype speculated that capsule might also correlate with virulence. Furthermore, we found that MICs had a positive correlation with virulence, consistent with the report in CoRKp of various sequence types [Citation57]. The emergence of modified-mgrB-mediated colistin-resistant K. pneumoniae should be monitored to prevent it from becoming a severe public health challenge.
We also studied the biofilm-forming capacity of colistin against K. pneumoniae in vitro and found that it was reduced in isolates with high colistin MICs, which was consistent with several reports in other pathogens. As it was documented, the biofilm formation was significantly reduced in colistin-resistant isolate in Salmonella typhimurium, when compared with colistin-susceptible isolates [Citation58]. The conclusion in Acinetobacter baumannii was the same. Both in vitro and vivo analysis revealed that isolates with lower MICs had stronger biofilms which were associated with mutations in colistin-modified TCS pmrB [Citation59]. Besides, we speculated there were some relationships between biofilm and TCSs. In Pseudomonas aeruginosa, a study showed that multiple extracellular DNA acidify in biofilm could induce the expression of phoPQ and pmrAB. However, it did not reveal whether colistin resistance could elevate biofilm formation capability [Citation60]. We conjectured that there would be a similar phenotype in K. pneumoniae, and more researches are still needed. In this study, we used RNA-seq to reveal genes differentially express between strong biofilm producers in CoRKp and ATCC13883. We found mrkA, mrkD and mrkC were significantly up-regulated, which all participated in type III fimbria [Citation61]. Our study showed that colistin-resistant MDR strains could have strong biofilm-forming ability which might make colonized K. pneumoniae more difficult to treat.
In conclusion, we found a low prevalence of mcr genes in CoRKp during 2017–2018 in China, which means that chromosome-mediated mechanism was still the main cause of colistin-resistance in K. pneumoniae. The up-regulated expression of TCS, especially phoPQ, played an essential role in colistin-resistant mechanism. And mgrB inactivation was a major contributor to the overexpression of chromosomal regulators. Additionally, most of the CoR-CRKp strains, clustered in ST11 lineage, were hvKp as well, which might be associated with the currently reported high mortality rate in CoR-CRKp. Thus, continuous surveillance of K. pneumoniae, especially hypervirulent CoR-CRKp, is an urgent priority to help prevent their spread.
Supplemental Material
Download Zip (9.2 MB)Acknowledgments
This work was supported by National Natural Science Foundation of China (No. 82072318), National Key Research and Development Program of China (2021YFC2301000, 2021YFC2301002, 2018YFE0101800), Beijing Key Clinical Specialty for Laboratory Medicine - Excellent Project (No. ZK201000).
Disclosure statement
No potential conflict of interest was reported by the author(s).
Data availability
The raw data of both whole-genome DNA and RNA sequencing data have been deposited in the NCBI Sequence Read Archive under BioProject ID PRJNA762527.
Additional information
Funding
References
- Wang X, Liu Y, Qi X, et al. Molecular epidemiology of colistin-resistant Enterobacteriaceae in inpatient and avian isolates from China: high prevalence of mcr-negative Klebsiella pneumoniae. Int J Antimicrob Agents. 2017 Oct;50(4):536–541.
- Cai Y, Lee W, Kwa AL. Polymyxin B versus colistin: an update. Expert Rev Anti Infect Ther. 2015;13(12):1481–1497.
- Mobasseri G, Teh CSJ, Ooi PT, et al. The emergence of colistin-resistant Klebsiella pneumoniae strains from swine in Malaysia. J Glob Antimicrob Resist. 2019 Jun;17:227–232.
- Olaitan AO, Diene SM, Kempf M, et al. Worldwide emergence of colistin resistance in Klebsiella pneumoniae from healthy humans and patients in Lao PDR, Thailand, Israel, Nigeria and France owing to inactivation of the PhoP/PhoQ regulator mgrB: an epidemiological and molecular study. Int J Antimicrob Agents. 2014 Dec;44(6):500–507.
- Trimble MJ, Mlynarcik P, Kolar M, et al. Polymyxin: alternative mechanisms of action and resistance. Cold Spring Harb Perspect Med. 2016 Oct 3;6(10).
- Moffatt JH, Harper M, Boyce JD. Mechanisms of polymyxin resistance. Adv Exp Med Biol. 2019;1145:55–71.
- Liu Y-Y, Wang Y, Walsh TR, et al. Emergence of plasmid-mediated colistin resistance mechanism MCR-1 in animals and human beings in China: a microbiological and molecular biological study. Lancet Infect Dis. 2016;16(2):161–168.
- Yang Q, Pogue JM, Li Z, et al. Agents of last resort: An update on polymyxin resistance. Infect Dis Clin North Am. 2020 Dec;34(4):723–750.
- Bialek-Davenet S, Criscuolo A, Ailloud F, et al. Genomic definition of hypervirulent and multidrug-resistant Klebsiella pneumoniae clonal groups. Emerg Infect Dis. 2014 Nov;20(11):1812–1820.
- Zou H, Jia X, He X, et al. Emerging threat of multidrug resistant pathogens from neonatal sepsis. Front Cell Infect Microbiol. 2021;11:694093.
- Gu D, Dong N, Zheng Z, et al. A fatal outbreak of ST11 carbapenem-resistant hypervirulent Klebsiella pneumoniae in a Chinese hospital: a molecular epidemiological study. Lancet Infect Dis. 2018;18(1):37–46.
- Guducuoglu H, Gursoy NC, Yakupogullari Y, et al. Hospital outbreak of a colistin-resistant, NDM-1- and OXA-48-producing Klebsiella pneumoniae: high mortality from pandrug resistance. Microb Drug Resist. 2018 Sep;24(7):966–972.
- Huang YH, Chou SH, Liang SW, et al. Emergence of an XDR and carbapenemase-producing hypervirulent Klebsiella pneumoniae strain in Taiwan. J Antimicrob Chemother. 2018 Aug 1;73(8):2039–2046.
- Zhang H, Johnson A, Zhang G, et al. Susceptibilities of gram-negative bacilli from hospital- and community-acquired intra-abdominal and urinary tract infections: a 2016-2017 update of the Chinese SMART study. Infect Drug Resist. 2019;12:905–914.
- CLSI. CLSI document M100-ED30 Performance standards for antimicrobial susceptibility testing; thrityth informational supplement.
- EUCAST. European Committee on Antimicrobial Susceptibility Testing Breakpoint tables for interpretation of MICs and zone diameters Version 10.0.
- Bolger AM, Lohse M, Usadel B. Trimmomatic: a flexible trimmer for Illumina sequence data. Bioinformatics. 2014 Aug 1;30(15):2114–2120.
- Jolley K, Bray J, Maiden M. Open-access bacterial population genomics: BIGSdb software, the PubMLST.org website and their applications. Wellcome open research. 2018. 3:124.
- Joensen KG, Tetzschner AM, Iguchi A, et al. Rapid and easy In silico serotyping of Escherichia coli isolates by Use of whole-genome sequencing data. J Clin Microbiol. 2015 Aug;53(8):2410–2426.
- Wyres KL, Lam MMC, Holt KE. Population genomics of Klebsiella pneumoniae. Nat Rev Microbiol. 2020 Jun;18(6):344–359.
- Kurtz S, Phillippy A, Delcher A, et al. Versatile and open software for comparing large genomes. Genome Biol. 2004;5(2):R12.
- Nguyen LT, Schmidt HA, von Haeseler A, et al. IQ-TREE: a fast and effective stochastic algorithm for estimating maximum-likelihood phylogenies. Mol Biol Evol. 2015 Jan;32(1):268–274.
- Zankari E, Allesoe R, Joensen KG, et al. Pointfinder: a novel web tool for WGS-based detection of antimicrobial resistance associated with chromosomal point mutations in bacterial pathogens. J Antimicrob Chemother. 2017 Oct 1;72(10):2764–2768.
- Bortolaia V, Kaas RS, Ruppe E, et al. Resfinder 4.0 for predictions of phenotypes from genotypes. J Antimicrob Chemother. 2020 Dec 1;75(12):3491–3500.
- Rebelo AR, Bortolaia V, Kjeldgaard JS, et al. Multiplex PCR for detection of plasmid-mediated colistin resistance determinants, mcr-1, mcr-2, mcr-3, mcr-4 and mcr-5 for surveillance purposes. Euro Surveill. 2018 Feb;23(6).
- Borowiak M, Baumann B, Fischer J, et al. Development of a novel mcr-6 to mcr-9 Multiplex PCR and Assessment of mcr-1 to mcr-9 occurrence in colistin-resistant Salmonella enterica isolates from environment, feed, animals and food (2011-2018) in Germany. Front Microbiol. 2020;11:80.
- Wang C, Feng Y, Liu L, et al. Identification of novel mobile colistin resistance gene mcr-10. Emerg Microbes Infect. 2020;9(1):508–516.
- Feldgarden M, Brover V, Gonzalez-Escalona N, et al. AMRFinderPlus and the reference gene catalog facilitate examination of the genomic links among antimicrobial resistance, stress response, and virulence. Sci Rep. 2021 Jun 16;11(1):12728.
- Wang Y, Wang S, Chen W, et al. CRISPR-Cas9 and CRISPR-assisted cytidine deaminase enable precise and efficient genome editing in Klebsiella pneumoniae. Appl Environ Microbiol. 2018;84(23).
- Liu B, Zheng D, Jin Q, et al. VFDB 2019: a comparative pathogenomic platform with an interactive web interface. Nucleic Acids Res. 2019 Jan 8;47(D1):D687–D692.
- Tian D, Wang W, Li M, et al. Acquisition of the conjugative virulence plasmid from a CG23 hypervirulent Klebsiella pneumoniae strain enhances bacterial virulence. Front Cell Infect Microbiol. 2021;11:752011.
- Russo TA, MacDonald U, Hassan S, et al. An assessment of siderophore production. Mucoviscosity, and mouse infection models for defining the virulence spectrum of hypervirulent Klebsiella Pneumoniae. MSphere. 2021 Mar 24;6(2).
- Crofts AA, Giovanetti SM, Rubin EJ, et al. Enterotoxigenic E. coli virulence gene regulation in human infections. Proc Natl Acad Sci U S A. 2018 Sep 18;115(38):E8968–E8976.
- Vuotto C, Longo F, Pascolini C, et al. Biofilm formation and antibiotic resistance in Klebsiella pneumoniae urinary strains. J Appl Microbiol. 2017 Oct;123(4):1003–1018.
- Anders S, Pyl P, Huber W. HTSeq–a python framework to work with high-throughput sequencing data. Bioinformatics (Oxford. England). 2015;31(2):166–169.
- Love M, Huber W, Anders S. Moderated estimation of fold change and dispersion for RNA-seq data with DESeq2. Genome Biol. 2014;15(12):550.
- Yu G, Wang L, Han Y, et al. Clusterprofiler: an R package for comparing biological themes among gene clusters. Omics: A Journal of Integrative Biology. 2012;16(5):284–287.
- Langstraat J, Bohse M, Clegg S. Type 3 fimbrial shaft (MrkA) of Klebsiella pneumoniae, but not the fimbrial adhesin (MrkD), facilitates biofilm formation. Infect Immun. 2001 Sep;69(9):5805–5812.
- Allan RN, Skipp P, Jefferies J, et al. Pronounced metabolic changes in adaptation to biofilm growth by Streptococcus pneumoniae. PLoS One. 2014;9(9):e107015.
- Yang X, Peng K, Zhang Y, et al. Characterization of a novel mcr-8.2-bearing plasmid in ST395 Klebsiella pneumoniae of chicken origin. Infect Drug Resist. 2020;13:1781–1784.
- Yan W, Zhang Q, Zhu Y, et al. Molecular mechanism of polymyxin resistance in multidrug-resistant Klebsiella pneumoniae and Escherichia coli isolates from henan province, China: A multicenter study. Infect Drug Resist. 2021;14:2657–2666.
- Haeili M, Javani A, Moradi J, et al. Mgrb alterations mediate colistin resistance in Klebsiella pneumoniae isolates from Iran. Front Microbiol. 2017;8:2470.
- Xu Q, Xu T, Zhuang Y, et al. In vivo development of polymyxin B resistance in Klebsiella pneumoniae owing to a 42 bp deletion in the sequence of phoQ. Biomed Res Int. 2020;2020:5868479.
- Giordano C, Barnini S, Tsioutis C, et al. Expansion of KPC-producing Klebsiella pneumoniae with various mgrB mutations giving rise to colistin resistance: the role of ISL3 on plasmids. Int J Antimicrob Agents. 2018 Feb;51(2):260–265.
- Shamina OV, Kryzhanovskaya OA, Lazareva AV, et al. Emergence of a ST307 clone carrying a novel insertion element MITEKpn1 in the mgrB gene among carbapenem-resistant Klebsiella pneumoniae from Moscow, Russia. Int J Antimicrob Agents. 2020 Feb;55(2):105850.
- Yang TY, Wang SF, Lin JE, et al. Contributions of insertion sequences conferring colistin resistance in Klebsiella pneumoniae. Int J Antimicrob Agents. 2020 Mar;55(3):105894.
- Jayol A, Poirel L, Brink A, et al. Resistance to colistin associated with a single amino acid change in protein PmrB among Klebsiella pneumoniae isolates of worldwide origin. Antimicrob Agents Chemother. 2014 Aug;58(8):4762–4766.
- Jayol A, Nordmann P, Brink A, et al. Heteroresistance to colistin in Klebsiella pneumoniae associated with alterations in the PhoPQ regulatory system. Antimicrob Agents Chemother. 2015 May;59(5):2780–2784.
- Cheng YH, Lin TL, Pan YJ, et al. Colistin resistance mechanisms in Klebsiella pneumoniae strains from Taiwan. Antimicrob Agents Chemother. 2015 May;59(5):2909–2913.
- Gao R, Stock AM. Biological insights from structures of two-component proteins. Annu Rev Microbiol. 2009;63:133–154.
- Cheng YH, Lin TL, Lin YT, et al. Amino acid substitutions of CrrB responsible for resistance to colistin through CrrC in Klebsiella pneumoniae. Antimicrob Agents Chemother. 2016 Jun;60(6):3709–3716.
- Rojas LJ, Salim M, Cober E, et al. Colistin resistance in carbapenem-resistant Klebsiella pneumoniae: Laboratory detection and impact on mortality. Clin Infect Dis. 2017 Mar 15;64(6):711–718.
- Zhang B, Yu B, Zhou W, et al. Klebsiella pneumoniaemobile plasmid mediated transition from colistin-sensitive to resistant phenotype in. Front Microbiol. 2021;12:619369.
- Kidd TJ, Mills G, Sa-Pessoa J, et al. A Klebsiella pneumoniae antibiotic resistance mechanism that subdues host defences and promotes virulence. EMBO Mol Med. 2017 Apr;9(4):430–447.
- Lin Z, Cai X, Chen M, et al. Virulence and stress responses of Shigella flexneri regulated by PhoP/PhoQ. Front Microbiol. 2017;8:2689.
- de Campos TA, Goncalves LF, Magalhaes KG, et al. A fatal bacteremia caused by hypermucousviscous KPC-2 producing extensively drug-resistant K64-ST11 Klebsiella pneumoniae in Brazil. Front Med (Lausanne. 2018;5:265.
- Esposito EP, Cervoni M, Bernardo M, et al. Molecular epidemiology and virulence profiles of colistin-resistant Klebsiella pneumoniae blood isolates from the Hospital agency “Ospedale dei Colli,” Naples, Italy. Front Microbiol. 2018;9:1463.
- Li L, Li R, Qi C, et al. Mechanisms of polymyxin resistance induced by Salmonella typhimurium in vitro. Vet Microbiol. 2021 Jun;257:109063.
- Farshadzadeh Z, Taheri B, Rahimi S, et al. Growth rate and biofilm formation ability of clinical and laboratory-evolved colistin-resistant strains of Acinetobacter baumannii. Front Microbiol. 2018;9:153.
- Wilton M, Charron-Mazenod L, Moore R, et al. Extracellular DNA acidifies biofilms and induces aminoglycoside resistance in Pseudomonas aeruginosa. Antimicrob Agents Chemother. 2016 Jan;60(1):544–553.
- Alcantar-Curiel MD, Blackburn D, Saldana Z, et al. Multi-functional analysis of Klebsiella pneumoniae fimbrial types in adherence and biofilm formation. Virulence. 2013 Feb 15;4(2):129–138.