ABSTRACT
With the development of multidrug resistance in Salmonella spp. in recent years, ciprofloxacin, ceftriaxone, and azithromycin have become the principal antimicrobial agents used for the treatment of Salmonella infections. The underlying mechanisms of plasmid-mediated ciprofloxacin and ceftriaxone resistance have attracted extensive research interest, but not much is focused on azithromycin resistance in Salmonella. In this study, we investigated the genetic features of two conjugative plasmids and a non-transferable virulence plasmid that encode azithromycin resistance in food-borne Salmonella strains. We showed that the azithromycin resistance phenotype of these strains was conferred by erm(B) gene and/or the complete genetic structure IS26-mph(A)-mrx-mphR-IS6100. Comparative genetic analysis showed that these conjugative plasmids might originate from Escherichia coli and play a role in the rapid dissemination of azithromycin resistance in Salmonella. These conjugative plasmids may also serve as a reservoir of antimicrobial resistance (AMR) genes in Salmonella in which these AMR genes may be acquired by the virulence plasmids of Salmonella via genetic transposition events. Importantly, the formation of a novel macrolide-resistance and virulence-encoding plasmid, namely pS1380-118 kb, was observed in this study. This plasmid was found to exhibit transmission potential and pose a serious health threat as the extensive transmission of azithromycin resistant and virulent Salmonella strains would further compromise the effectiveness of treatment for salmonellosis. Further surveillance and research on the dissemination and evolution routes of pS1380-118kb-like plasmids in potential human pathogens of the family of Enterobacteriaceae are necessary.
Introduction
Salmonella is a primary cause of food-borne diseases and is considered to be a major public health threat worldwide [Citation1,Citation2]. Most cases of salmonellosis are self-limiting, yet antimicrobial treatment is necessary in cases of systematic and serious infection, especially in immune-compromised and elderly patients [Citation3]. Due to the increasing incidence of resistance to ciprofloxacin and ceftriaxone, azithromycin is regarded as a last sort, FDA-approved antimicrobial agent for the treatment of systemic Salmonella infections, especially those caused by S. Typhimurium [Citation4]. Azithromycin is a semisynthetic macrolide antibiotic derived from erythromycin by methyl substitution of a nitrogen atom in the macrolide ring. It differs structurally from erythromycin and exhibits stability in an acidic environment, a wide antibacterial spectrum, negligible irritability, and rapid absorption from gastrointestinal (GI) tract upon oral administration [Citation5], and has become one of the most commonly used antimicrobials after being launched in 1991 [Citation6]. The excellent pharmacological characteristics and favourable membrane permeability of azithromycin allow it to be used for the treatment of infections caused by various members of Enterobacteriaceae, especially these common foodborne pathogens of E. coli, Shigella spp., or Salmonella spp. [Citation7,Citation8]. The key antimicrobial mechanism of azithromycin involves inhibition of protein synthesis and bacterial growth by binding to the bacterial ribosome and therefore hindering mRNA translation. To date, two other mechanisms of azithromycin resistance have been reported: one is associated with extruding antibiotics from bacterial cytoplasm by efflux. Relevant bacterial efflux system families are ABC, MFS, MATE, RND, and SMR, among which genes encoding the ABC family efflux pumps including msr(A), msr(D), and msr(E), and those which encode the MFS family, such as the mef(A) and mef(B) genes, are transferable among different bacterial pathogens [Citation9,Citation10]. The second mechanism involves the modification of either the bacterial ribosome or the molecular structure of macrolides by rRNA methylases, esterases, and phosphorylases, which are encoded by the erm, ere(A)/ere(B) and mph(A)/mph(B) genes, respectively [Citation11]. However, plasmids carrying these determinants mediating azithromycin resistance are sporadically reported in Salmonella strains, among which plasmids of IncHI2 and IncC types were reported in recent years [Citation12–14], and most of these plasmids originate from other Enterobacteriaceae. In addition, extensively drug resistant (XDR) S. typhimurium with a single point mutation in the efflux pump AcrB (R717Q/L) is the main epidemic azithromycin resistant isolate in recent years in Southeast Asian countries, especially in Nepal, India, and Pakistan [Citation15].
In this study, we performed genetic characterization of two conjugative plasmids that carry an rRNA methylase gene erm(B) and a phosphorylase-encoding cluster with the structure IS26-mph(A)-mrx-mphR-IS6100. One was an IncI1 type plasmid that can acquire macrolide-resistance genes and was responsible for causing an increasing prevalence of azithromycin resistance in Salmonella. The other was an IncFIC/IncFIB type plasmid, which was commonly detected in E. coli strains and responsible for transferring azithromycin resistance genes to a typical virulence plasmid, forming a novel azithromycin resistance and virulence-encoding plasmid in S. typhimurium. These events would lead to an increase in the incidence of life-threatening Salmonella infections and pose a serious threat to human health.
Materials and methods
Bacterial isolation and antimicrobial susceptibility tests
Salmonella strains S1330 and S1380 were isolated from retail pork samples collected from supermarkets in Shenzhen, China in 2013. Species identification was performed by detection of the Salmonella-specific invA gene and by using the MALDI-TOF-MS Biotyper System (Bruker, Germany). The serotypes of the two strains were then identified according to the Kauffmann-White scheme, using a commercial antiserum (Difco, Detroit, MI). Antimicrobial susceptibility tests were performed by following the microdilution method recommended by Clinical and Laboratory Standards Institute [Citation16]. Escherichia coli strain ATCC 25922 and Staphylococcus aureus strain ATCC 29213 were used as quality control.
Cloning of two resistance determinant-bearing clusters IS26-mph(A)-mrx-mphR-IS6100, IS26-mph(A)-mrx-ΔmphR-IS26, and mph(A) gene
The corresponding gene fragments were amplified using the primers listed in . Briefly, sequences of 200 bp located up- and downstream of the target genes, including the natural promoters, were amplified. PCR products were then ligated to the cloning vector pBackZero-T, generating pBackZero-mph(A), pBackZero-IS26-mph(A)-mrx-mphR-IS6100 and pBackZero-IS26-mph(A)-mrx-ΔmphR-IS26 respectively, which were then transformed into E. coli strain DH5α. Transformants were selected on LB plates containing 50μg mL−1 kanamycin, followed by confirmation of their genetic identity through PCR screening with the cloning primers described above.
Conjugation and pulsed-field gel electrophoresis (PFGE) analysis
Conjugation experiments were performed to evaluate the transmission potential of azithromycin resistance in Salmonella strains, with a sodium azide resistant E. coli strain J53 being used as the recipient. In brief, overnight culture of the test Salmonella strains and the recipient were diluted 100-fold in fresh Luria Bertani (LB) broth and incubated at 37°C for 4 h until the logarithmic phase was reached. The Salmonella strains and the recipient were mixed at a ratio of 1:4 and inoculated onto a 0.45 µm membrane placed on LB agar. After incubation for 24 h at 37°C, serial dilutions of the bacterial mixture were incubated onto Eosin Methylene Blue (EMB) agar supplemented with 100 µg mL−1 sodium azide and 16 µg mL−1 azithromycin for selection of transconjugants. The transconjugants were then identified by antimicrobial susceptibility tests and pulsed-field gel electrophoresis (PFGE) as previously described [Citation17].
Plasmid sequencing and bioinformatics analysis
Plasmids harboured by Salmonella strains S1330 and S1380 were extracted using the Qiagen Plasmid Midi Kit (Qiagen, Valencia, CA) and then sequenced by the NextSeq Illumina platform (San Giego, CA) and Nanopore MinION (long-read) sequencing platform, using the MinION R9.4.1 flow cell [Citation18]. The complete plasmid sequence was acquired by assembling the short and long reads obtained from both Illumina and Nanopore platforms by Unicycler v0.4.9b [Citation19], and then annotated by Rapid Annotation using Subsystem Technology (RAST) version 2.0 [Citation20]. Antimicrobial resistance genes, insertion sequences and plasmid incompatibility types were identified by ResFinder [Citation21], ISFinder [Citation22], and PlasmidFinder [Citation23] tools. Sequence comparison of plasmids was conducted using BLAST Ring Image Generator (BRIG) [Citation24] and Easyfig [Citation25].
Results
Phenotypic characterization of Salmonella strains
Salmonella Derby strain S1330 and Salmonella Typhimurium strain S1380 were isolated from retail pork samples in Shenzhen and found to be resistant to most of the antibiotics tested, including azithromycin, chloramphenicol, ciprofloxacin, kanamycin, nalidixic acid, trimethoprim/sulfamethoxazole, and tetracycline, but the strains remained susceptible to amikacin and meropenem (). Conjugation assays indicated that the azithromycin resistance phenotype of both isolates could be transferred to the recipient E. coli J53 upon selection by azithromycin. The transconjugants S1330-TC and S1380-TC exhibited dramatic increase in minimum inhibitory concentration (MIC) of azithromycin when compared with the recipient E. coli J53, with more than 128-fold increase in azithromycin MIC being observed (). S1 nuclease pulsed-field gel electrophoresis (S1-PFGE) illustrated that both the S. Derby strain S1330 and the corresponding transconjugant contained a single plasmid of ∼110 kb, which was designated as plasmid pS1330-110 kb. In addition, S1-PFGE results showed that S. Typhimurium strain S1380 harbored two plasmids, the size of which were found to be ∼118 and ∼135 kb by plasmid sequencing. However, only the larger plasmid (∼135 kb) could be transferred to the recipient by conjugation (). These two plasmids were designated as plasmid pS1380-118 kb and pS1380-135 kb, respectively.
Figure 1. PFGE profiles of Salmonella strains S1330 and S1380 and the corresponding transconjugants. XbaI-PFGE and S1-PFGE profiles of Salmonella strains S1330, S1380 and their transconjugants are shown.
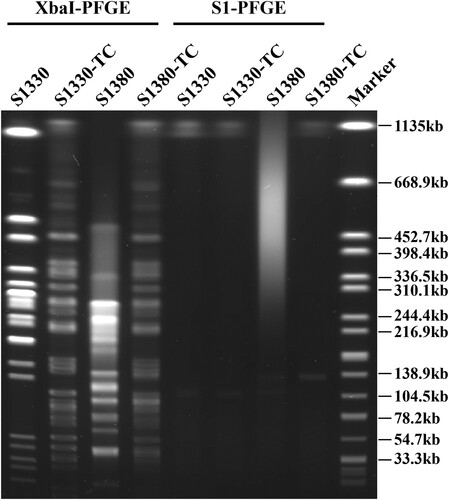
Table 1. Phenotypic characteristics of Salmonella strains tested in this study.
Genetic analysis of plasmids that confer phenotypic resistance to azithromycin in Salmonella
Complete sequences of plasmids pS1330-110 kb, pS1380-118 kb and pS1380-135 kb were acquired using both NextSeq Illumina and Nanopore MinION sequencing platforms. Plasmid pS1330-110 kb was 110,440 bp in length, exhibited a GC content of 50.2% and comprised 147 predicted coding sequences (CDS). The plasmid was found to belong to IncI1 type. This plasmid was found to harbour several resistance genes including mph(A), aadA1, aac(3)-IId, and erm(B), which may confer resistance to azithromycin and kanamycin, respectively. BLASTN analysis showed that plasmid pS1330-110 kb exhibited the highest degree of homology to a 115,776 bp IncI1 plasmid, namely pS68 (Accession: KU130396), with 99.98% identity and 91% coverage (a), as well as plasmid pNDM33-2 (Accession: MN915012) and pST1030-1A (Accession: MT507877), both recovered from Escherichia coli strains and shared similar plasmid backbones (b), with 99.8% identity and 85–94% coverage. Sequencing analysis showed that the backbones of these plasmids were extremely conserved after subtracting the multidrug resistance (MDR) region, a mosaic region that comprised various resistance genes including mph(A) and erm(B). The mph(A) cluster in plasmid pS1330-110 kb was incomplete in which the mphR in the complete mph(A) cluster (IS26-mph(A)-mrx-mphR-IS6100) was truncated and the followed mobile element IS6100 was replaced by an IS26. We confirmed that deletion of the mphR gene would affect the function of the mph(A)-bearing cluster and restore azithromycin sensitivity, as E. coli strain DH5α which acquired IS26-mph(A)-mrx-ΔmphR-IS26 and mph(A) exhibited sensitivity to azithromycin (azithromycin MIC of both strains were 1μg mL−1), whereas the azithromycin MIC of E. coli DH5α which acquired the complete structure of IS26-mph(A)-mrx-mphR-IS6100 increased to over 128μg mL−1 (). Our data confirmed that erm(B) on pS1330-110 kb could mediate azithromycin resistance by cloning experiments, which suggested that erm(B) was a key genetic element that encoded resistance to azithromycin in Salmonella S1330. The role of erm(B) was also consistent with our previous clonal study in which transconjugant E. coli DH5α-erm(B) exhibited a 128-fold increased MIC to azithromycin [Citation26].
Figure 2. Genetic structure of plasmids that confer azithromycin resistance in Salmonella strain S1330. Circular (a) and liner (b) alignment of plasmid pS1330-110 kb in S. Derby strain S1330 with plasmids deposited in the NCBI database including pNDM33-2 (MN915012), pS86 (KU130396), and pST1030-1A (MT507877) using Blast Ring Image Generator (BRIG) and EasyFig. (c) Liner alignment of the MDR region of plasmid pS1330-110 kb with the plasmids pYPE12 and pCTXM3-020032. Dark blue, gene encoding replication initiation protein; red, resistance gene; yellow, insertion sequence; green, gene encoding plasmid conjugative transfer protein Tra and Pil.
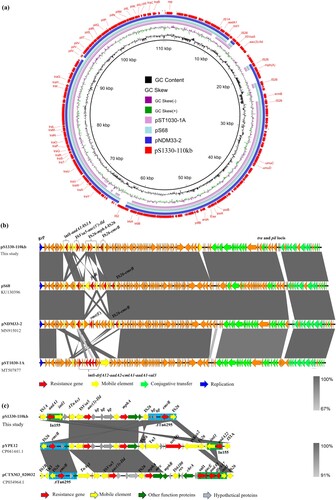
Table 2. MICs of DH5α strains that contained mph(A)-bearing fragments of different structures and relevant primers used.
Plasmid pS1380-135 kb was 134,634 bp in length, with a GC content of 52.1%. The plasmid comprised 184 predicted coding sequences and was found to belong to a IncFIC/IncFIB type plasmid. BLASTN screening against the resistance gene database showed that plasmid pS1380-135 kb harbored multidrug resistance genes, including the aminoglycoside resistance genes aph(3’’)-I, aph(3’)-Ia, aph(6’)-Id, aadA5 and acc(3)-II, the phenicol resistance gene catA2, the trimethoprim resistance gene dfrA17, the tetracycline resistance gene tet(A), the sulfonamide resistance genes sul1 and sul2, and the macrolide resistance genes mph(A) and erm(B) (a). These resistant determinants located in a ∼40 kb MDR region surrounded by various IS elements with the genetic environments of int-dfrA17-aadA5-sul1, IS26-mph(A)-mrx-mphR-IS6100, IS26-erm(B)-groEL-IS26, Tn3-aph(6’)-Id-aph(3’)-I-sul2-IS5075, and IS26-aph(3’)-la-ΔIS26-IS26, respectively. Plasmid pS1380-135 kb exhibited the highest degree of sequence homology (83% overage and 99.87% identity) to a 163,427 bp IncFIC/IncFIB plasmid pMUB-MIN12-1 (Accession: CP069658) recovered from a clinical E. coli strain collected from the wound of a patient. The IncFIC/IncFIB plasmid pSMS35_130 (Accession: CP000971) and plasmid p2 (Accession: LR890271), both recovered from E. coli, also exhibited similar sequence coverage and identity (a). The aph and tet(A) genes were found in all the IncFIC/IncFIB plasmids, even though a high degree of structural divergence was observed in the mosaic resistance regions of these plasmids. Importantly, the backbone sequences of these plasmids shared a common virulence gene cluster, sitABCD, indicating that such plasmids might have originated from pathogenic avian Escherichia coli (APEC) strains, as sitABCD is commonly located in large conjugative plasmids in APEC strains.
Figure 3. Genetic structure of plasmids that confer azithromycin resistance in Salmonella strain S1380. (a) Circular alignment of plasmid pS1380-118 kb in S. typhimurium strain S1380 with plasmids deposited in NCBI database including pST56-2 (CP050741), pST53-1 (CP050746), and pST90-2 (CP050736), using Blast Ring Image Generator (BRIG). (b) Circular alignment of plasmid and pS1380-135 kb in S. Typhimurium strain S1380 with plasmids deposited in NCBI database including pSMS35_130 (CP000971), p2 (LR890271), and pMUB-MIN12-1 (CP069658), using Blast Ring Image Generator (BRIG).
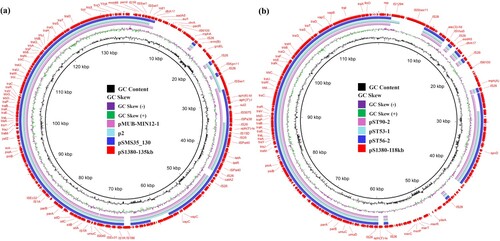
Figure 4. Genetic structure of plasmids that confer azithromycin resistance in Salmonella strain S1380. Liner alignment of plasmids pS1380-135 kb (a) and pS1380-118 kb (b) with plasmids deposited in NCBI database using Easyfig. (c) Alignment of plasmid pS1380-118 kb with plasmid pS1380-135 kb and virulence plasmid pSLT (CP060170). Dark blue, gene encoding replication initiation protein; red, resistance gene; pink, virulence gene; yellow, insertion sequence; green, genes encoding the plasmid conjugative transfer proteins Tra and Pil.
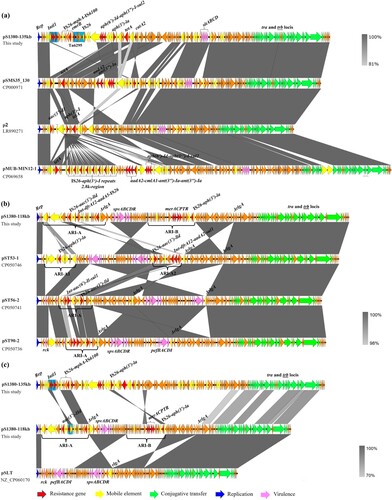
Plasmid pS1380-118 kb was 118,931 bp in length, exhibited a GC content of 53.9% and comprised 190 predicted coding sequences. This plasmid was found to belong to the IncFII type. BLASTN analysis demonstrated that plasmid pS1380-118 kb exhibited homology to various plasmids originated from Salmonella Typhimurium, including pST56-2 (Accession: CP050741), with 100.00% identity and 75% coverage, plasmid pST53-1 (Accession: CP050746) with 99.98% identity and 73% coverage, and plasmid pST90-2 (Accession: CP050736) with 99.95% identity and 75% coverage (b and b). In addition, two antimicrobial resistance islands (ARI) (designated as ARI-A and ARI-B) conferring resistance to aminoglycoside and azithromycin were detected in plasmid pS1380-118 kb. Blast against the ResFinder [Citation21] and ISfinder [Citation22] showed that the genetic structure of ARI-A was int-dfrA17-aadA5, ISVas5-aac(3’)-IId, followed by a macrolide resistance cluster IS26-mph(A)-mrx-mphR-IS6100, whereas ARI-B contained a mercury resistance cluster merACPTR and an aminoglycoside resistance-encoding transposon with a structure of IS26-aph(3’)-la-IS26 (c). The ∼17 kb ARI-B fragment only exhibited high level homology (99.34% similarity, 94% coverage) to plasmid pII (Accession number: LT795504), indicating that the ARI-B region of plasmid pS1380-118 kb might originate from E. coli strains such as KV7 [Citation27]. In addition, the plasmid backbone exhibited a high degree of sequence homology to a virulence plasmid, pSLT (Accession number: NZ_CP060170), after removing both ARI regions. Both plasmids contained the virulence locus spvABCDR, which encodes important virulence factors reported to play a role in enhancing the growth rate of Salmonella strains in mouse model during the systemic phase of infection [Citation28]. Compared with the virulence plasmid pSLT, the ARI-A region in plasmid pS1380-118 kb was found to be replaced by a ∼14 kb region containing the original virulence cluster pefBACDI and a virulence gene rck (c). The pef operon is associated with fimbriae biosynthesis and contributes to adherence to the intestinal epithelium, whereas the rck gene is associated with resistance to complement killing [Citation29]. The formation of ARI-B in plasmid pS1330-118 kb might be associated with the rlgA gene, which exhibited high sequence homology to several previously reported resolvase-like protein-encoding genes. The process probably involved sequence insertion and reversal, in which rlgA was truncated into two ΔrlgA genes and then orientated in opposite direction with the adjacent fragments (c). Spontaneous reversal of the rlgA gene is commonly observed in other virulence plasmids such as plasmid pST56-2, pST53-1, and pST90-2, even though they lacked the insertion fragment of ARI-B (b). In addition, pS1380-118kb-like plasmids only conferred phenotypic resistance to aminoglycoside antibiotics, since AMR genes including aph(3’)-la, aac(3’)-lld, dfrA12, and aadA2 were detected in the ARI-A region. BLASTN analysis showed that the ARI-A region from plasmid pS1380-118 kb exhibited homology (100% similarity, 68% ∼ 74% coverage) to plasmid pCTXM15_020026 (Accession number: CP034956), pECSE_01 (Accession number: KX683283) and pGMI17-003_3 (Accession number: CP031137), which were recovered from E. coli and lacked the genetic structure IS26-mph(A)-mrx-mphR-IS6100. This observation indicates that the macrolide-resistance encoding fragment might be derived from an exogenous plasmid, such as plasmid pS1380-135 kb.
Discussion
non-typhoidal Salmonella is an important pathogen that causes food-borne diseases, the prevalence of which ranked second among a total of 31 pathogens that cause 9.4 million cases of food poisoning in the United State each year [Citation30]. Salmonella is the etiologic agent of salmonellosis in humans causing severe diseases, especially in immunocompromised patients as well as children and the elderly [Citation3]. Ciprofloxacin, ceftriaxone, and azithromycin are antibiotics commonly used for the treatment of salmonellosis. Upon decades of evolution and accumulation of multidrug resistance-encoding elements in the bacterial genomes, novel structures in multidrug resistance plasmids have emerged and were found to be responsible for causing a sharp increase in the rate of resistance to these three antibiotics in Salmonella. Our recent work demonstrated that the IncI1 plasmids could acquire various β-lactamase encoding determinants such as the blaCTX-M group variants, resulting in an increasing prevalence of ceftriaxone resistance [Citation31]. This type of plasmid was subjected to extensive studies as they may act as helper plasmids that mediate further acquisition of ciprofloxacin resistance-encoding genes by fusing with non-conjugative MDR plasmids or fragments containing such genes [Citation32,Citation33]. In addition, a recent report has recovered a conjugative IncI1 plasmid carrying the erm(B) and blaCTX-M-104 genes that encode resistance to both cefotaxime and azithromycin from a clinical Klebsiella pneumoniae strain [Citation26]. These findings indicate that IncI1 type plasmids might play critical roles in the spreading of resistance genes and are worthy of further investigation. The emergence of novel ciprofloxacin resistance-encoding genetic elements has limited the usage of ciprofloxacin in the treatment of salmonellosis [Citation34]; hence azithromycin is increasingly regarded as an important last-line antibiotic for the treatment of Salmonella infections.
To date, vast majority of Salmonella enterica serovar Typhimurium strains (88%) harbor a virulence plasmid of approximately 90 kb [Citation35]. This virulence plasmid was reported to be non-conjugative, as it does not contain AMR genes and cannot be selected by antibiotics in conjugative assay [Citation36]. However, Ahmer et al. confirmed that it was a self-transmissible plasmid in Salmonella typhimurium, exhibiting a transmission frequency of 2.9 × 10−4 transconjugants per donor [Citation37]. Besides, virulence plasmids play significant roles in host adaptation and often affect bacterial vertical transmission among their preferred hosts [Citation38]. Unlike virulence plasmids in other non-typhoidal Salmonella that are only detected in fecal samples and cause gastroenteritis, S. typhimurium and S. enteritidis containing virulence plasmids can recover from blood specimens and cause severe Salmonella bacteremia [Citation39]. Furthermore, the absence of Salmonella plasmid virulence (spv) operons does not cause fatal infections in mice models, since virulence genes spvBCD are key factors that ruin host immunity [Citation40]. In the past two decades, the genetic structures of these virulence plasmids have been extremely conserved, only several plasmids deposited in the NCBI database were found to have acquired aminoglycoside resistance genes.
In this study, we performed genetic characterization of two azithromycin-resistant Salmonella isolates that can successfully transfer their azithromycin resistance phenotype to E. coli J53 via filter mating experiments. We found that the azithromycin resistance phenotype of these two strains was encoded by IncI1 and IncFIC/IncFIB type conjugative plasmids that harbored the erm(B) gene, as well as the IS26-mph(A)-mrx-mphR-IS6100 or IS26-mph(A)-mrx-ΔmphR-IS26 cluster. We confirmed that these genetic elements could confer azithromycin-resistance, as E. coli DH5α strains which acquired the erm(B) gene [Citation26] or the IS26-mph(A)-mrx-mphR-IS6100 fragment exhibited an increase in MICs of azithromycin to over 128 fold, but those which acquired the mph(A) gene alone or the structure of IS26-mph(A)-mrx-ΔmphR-IS26 did not. Importantly, we recovered a Salmonella virulence plasmid carrying the azithromycin resistance cluster IS26-mph(A)-mrx-mphR-IS6100; we hypothesized that it was the evolution product of azithromycin resistance plasmids, such as the plasmid pS1380-135 kb, upon extensive dissemination in S. Typhimurium.
In summary, this study identified two conjugative plasmids and a virulence plasmid that encoded resistance to azithromycin. Transmission of such azithromycin resistance-encoding plasmids in Salmonella not only limits the therapeutic options of Salmonella infections but also helps establish an AMR gene reservoir in Salmonella. The formation of novel multidrug resistance and virulence-encoding plasmids in S. typhimurium strains also leads to acceleration in the rate of dissemination of virulence plasmids as they would become more transmissible under the selection pressure of antimicrobial agents. Further surveillance and research on the transmission and evolution of virulence plasmids in Salmonella are necessary.
Acknowledgments
MMX performed the experiments and drafted the manuscript; KCC performed sequencing and bioinformatic analysis; KCC, EWCC and SC participated in research design and manuscript editing; SC supervised the project.
Disclosure statement
No potential conflict of interest was reported by the author(s).
Data availability statement
The plasmids sequencing data of pS1330-110 kb, pS1380-118 kb, and pS1380-135 kb have been deposited into GenBank under the accession numbers OM048933, OM048934, and OM048935, respectively.
Additional information
Funding
References
- Gomez TM, Motarjemi Y, Miyagawa S, et al. Foodborne salmonellosis. World Health Statistics Quarterly Rapport Trimestriel de Statistiques Sanitaires Mondiales. 1997;50(1–2):81.
- Boyen F, Haesebrouck F, Maes D, et al. Non-typhoidal Salmonella infections in pigs: a closer look at epidemiology, pathogenesis and control. Vet Microbiol. 2008;130(1–2):1–19.
- Acheson D, Hohmann EL. Nontyphoidal salmonellosis. Clin Infect Dis. 2001;32(2):263–269.
- Sjölund-Karlsson M, Joyce K, Blickenstaff K, et al. Antimicrobial susceptibility to azithromycin among Salmonella enterica isolates from the United States. Antimicrob Agents Chemother. 2011;55(9):3985–3989.
- Anandabaskar N. Protein synthesis inhibitors. Introduction to basics of pharmacology and toxicology. Springer; 2021. p. 835-868.
- Hicks LA, Taylor Jr TH, Hunkler RJ. US outpatient antibiotic prescribing, 2010. N Engl J Med. 2013;368(15):1461–1462.
- Trivedi N, Shah P. A meta-analysis comparing the safety and efficacy of azithromycin over the alternate drugs used for treatment of uncomplicated enteric fever. J Postgrad Med. 2012;58(2):112.
- Pons M, Gomes C, Martínez-Puchol S, et al. Antimicrobial resistance in Shigella spp. causing traveller's diarrhoea (1995–2010): a retrospective analysis. Travel Med Infect Dis. 2013;11(5):315–319.
- Arthur M, Andremont A, Courvalin P. Distribution of erythromycin esterase and rRNA methylase genes in members of the family Enterobacteriaceae highly resistant to erythromycin. Antimicrob Agents Chemother. 1987;31(3):404–409.
- Ojo K, Ulep C, Van Kirk N, et al. The mef (A) gene predominates among seven macrolide resistance genes identified in gram-negative strains representing 13 genera, isolated from healthy Portuguese children. Antimicrob Agents Chemother. 2004;48(9):3451–3456.
- Gomes C, Martínez-Puchol S, Palma N, et al. Macrolide resistance mechanisms in enterobacteriaceae: focus on azithromycin. Crit Rev Microbiol. 2017;43(1):1–30.
- Chen K, Yang C, Chan EW, et al. Emergence of conjugative IncC type plasmid simultaneously encoding resistance to ciprofloxacin, ceftriaxone, and azithromycin in salmonella. Antimicrob Agents Chemother. 2021;65(9):e01046–21.
- Zhang Z, Yang J, Xu X, et al. Co-existence of mphA, oqxAB and blaCTX-M-65 on the IncHI2 plasmid in highly drug-resistant Salmonella enterica serovar indiana ST17 isolated from retail foods and humans in China. Food Control. 2020;118:107269.
- Hong Y-P, Chen Y-T, Wang Y-W, et al. Integrative and conjugative element-mediated azithromycin resistance in multidrug-resistant Salmonella enterica serovar albany. Antimicrob Agents Chemother. 2021;65(5):e02634–20.
- Sajib MS, Tanmoy AM, Hooda Y, et al. Tracking the emergence of azithromycin resistance in multiple genotypes of typhoidal salmonella. Mbio. 2021;12(1):e03481–20.
- CLSI C. Performance standards for antimicrobial susceptibility testing. Clinical Lab Standards Institute. 2016.
- Tenover FC, Arbeit RD, Goering RV, et al. Interpreting chromosomal DNA restriction patterns produced by pulsed-field gel electrophoresis: criteria for bacterial strain typing. J Clin Microbiol. 1995;33(9):2233–2239.
- Li R, Xie M, Dong N, et al. Efficient generation of complete sequences of MDR-encoding plasmids by rapid assembly of MinION barcoding sequencing data. Gigascience. 2018;7(3):gix132.
- Wick RR, Judd LM, Gorrie CL, et al. Unicycler: resolving bacterial genome assemblies from short and long sequencing reads. PLoS Comput Biol. 2017;13(6):e1005595.
- Aziz RK, Bartels D, Best AA, et al. The RAST server: rapid annotations using subsystems technology. BMC Genomics. 2008;9(1):1–15.
- Kleinheinz KA, Joensen KG, Larsen MV. Applying the ResFinder and VirulenceFinder web-services for easy identification of acquired antibiotic resistance and E. coli virulence genes in bacteriophage and prophage nucleotide sequences. Bacteriophage. 2014;4(2):e27943.
- Siguier P, Varani A, Perochon J, et al. Exploring bacterial insertion sequences with ISfinder: objectives, uses, and future developments. Mobile Genetic Elements. 2012;859:91–103.
- Carattoli A, Zankari E, García-Fernández A, et al. In silico detection and typing of plasmids using PlasmidFinder and plasmid multilocus sequence typing. Antimicrob Agents Chemother. 2014;58(7):3895–3903.
- Alikhan N-F, Petty NK, Zakour NLB, et al. BLAST ring image generator (BRIG): simple prokaryote genome comparisons. BMC Genomics. 2011;12(1):1.
- Sullivan MJ, Petty NK, Beatson SA. Easyfig: a genome comparison visualizer. Bioinformatics. 2011;27(7):1009–1010.
- Yang X, Liu X, Yang C, et al. A conjugative IncI1 plasmid carrying erm (B) and bla CTX-M-104 that mediates resistance to azithromycin and cephalosporins. Microbiol Spectr. 2021;9(2):e00286–21.
- Bateman MD, de Vries SP, Gupta S, et al. Genome and plasmid sequences of Escherichia coli KV7, an extended-spectrum β-lactamase isolate derived from feces of a healthy pig. Genome Announc. 2017;5(38):e00595–17.
- McClelland M, Sanderson KE, Spieth J, et al. Complete genome sequence of Salmonella enterica serovar Typhimurium LT2. Nature. 2001;413(6858):852–856.
- Rotger R, Casadesús J. The virulence plasmids of salmonella. Int Microbiol. 1999;2:177–184.
- Bell RL, Jarvis KG, Ottesen AR, et al. Recent and emerging innovations in Salmonella detection: a food and environmental perspective. Microb Biotechnol. 2016;9(3):279–292.
- Wong M-y, Kan B, Chan E-c, et al. Inci1 plasmids carrying various bla CTX-M genes contribute to ceftriaxone resistance in Salmonella enterica serovar Enteritidis in China. Antimicrob Agents Chemother. 2016;60(2):982–989.
- Chen K, Dong N, Chan EW-C, et al. Transmission of ciprofloxacin resistance in Salmonella mediated by a novel type of conjugative helper plasmids. Emerg Microbes Infect. 2019;8(1):857–865.
- Yang C, Chen K, Chan EW-C, et al. Transmission of chromosomal MDR DNA fragment encoding ciprofloxacin resistance by a conjugative helper plasmid in salmonella. Front Microbiol. 2020;11:2215.
- Chen K, Yang C, Dong N, et al. Evolution of ciprofloxacin resistance-encoding genetic elements in salmonella. Msystems. 2020;5(6):e01234–20.
- Helmuth R, Stephan R, Bunge C, et al. Epidemiology of virulence-associated plasmids and outer membrane protein patterns within seven common Salmonella serotypes. Infect Immun. 1985;48(1):175–182.
- Jones G, Rabert D, Svinarich D, et al. Association of adhesive, invasive, and virulent phenotypes of Salmonella typhimurium with autonomous 60-megadalton plasmids. Infect Immun. 1982;38(2):476–486.
- Ahmer BM, Tran M, Heffron F. The virulence plasmid of Salmonella typhimurium is self-transmissible. J Bacteriol. 1999;181(4):1364–1368.
- Fierer J. Invasive Non-typhoidal Salmonella (iNTS) infections. Clin Infect Dis. 2022. DOI: 10.1093/cid/ciac035
- Fierer J, Krause M, Tauxe R, et al. Salmonella typhimurium bacteremia: association with the virulence plasmid. J Infect Dis. 1992;166(3):639–642.
- Guiney DG, Fang FC, Krause M, et al. Biology and clinical significance of virulence plasmids in Salmonella serovars. Clin Infect Dis. 1995;21(Suppl._2):S146–S151.