ABSTRACT
Three or four intramuscular doses of the inactivated human rabies virus vaccines are needed for pre- or post-exposure prophylaxis in humans. This procedure has made a great contribution to prevent human rabies deaths, which bring huge economic burdens in developing countries. Herein, a recombinant adeno-associated virus serotype 9, AAV9-RABVG, harbouring a RABV G gene, was generated to serve as a single dose rabies vaccine candidate. The RABV G protein was stably expressed in the 293T cells infected with AAV9-RABVG. A single dose of 2 × 1011 v.p. of AAV9-RABVG induced robust and long-term positive seroconversions in BALB/c mice with a 100% survival from a lethal RABV challenge. In Cynomolgus Macaques vaccinated with a single dose of 1 × 1013 v.p. of AAV9-RABVG, the titres of rabies VNAs increased remarkably from 2 weeks after immunity, and maintained over 31.525 IU/ml at 52 weeks. More DCs were activated significantly for efficient antigen presentations of RABV G protein, and more B cells were activated to be responsible for antibody responses. Significantly more RABV G specific IFN-γ-secreting CD4+ and CD8+ T cells, and IL-4-secreting CD4+ T cells were activated, and significantly higher levels of IL-2, IFN-γ, IL-4, and IL-10 were secreted to aid immune responses. Overall, the AAV9-RABVG was a single dose rabies vaccine candidate with great promising by inducing robust, long-term humoral responses and both Th1 and Th2 cell-mediated immune responses in mice and non-human primates.
Introduction
Rabies remains a great public health problem in developing countries and is responsible for at least 60,000 human deaths every year worldwide [Citation1,Citation2]. Rabies is an ancient zoonotic viral infectious disease caused by rabies virus (RABV) and has been recorded in 150 territories [Citation3]. The RABV is a highly neurotropic virus that affects the central nervous system (CNS) in the mammalian host and the outcome is usually fatal once clinical manifestations are apparent [Citation4,Citation5]. RABV could naturally infect all the warm-blooded animals, including companion animals, carnivores, wildlife animals, and humans. The bats and other wildlife can also act as important reservoir hosts and transmitters for RABV, and are associated with most of human rabies cases found in North America [Citation6]. However, dogs are the most transmissible hosts for RABV, and the dog bites account for >99% of human cases in the rabies epidemic countries in Asia and Africa [Citation7–9]. Rabies is a vaccine-preventable fatal disease in human and animals. The prevention of canine rabies through vaccination is essential to eliminating human rabies in countries where dogs are the primary source of human infections [Citation10]. In the past decades, the massive canine vaccination programmes and widespread immunization of humans have significantly reduced the number of human rabies deaths in industrialized countries and many urbanized areas of developing countries [Citation11]. However, the pre-exposure prophylaxis (PrEP) currently consists of a 3-dose series of injections by intramuscular administration on days 0, 7, and 21 or 28, and the annual regular booster doses are required to maintain the immunity [Citation12,Citation13]. The simplified 4-dose regimen recommended by WHO is currently widely applied for post exposure prophylaxis (PEP) following exposure to a rabid or potentially rabid animal by intramuscular administration on days 0, 3, 7, and 14. The vaccinations of PEP are mainly injected in combination with human (HRIG) or equine (ERIG) anti-rabies immunoglobulin, which is often short supplied in rural areas in developing countries, and adds to the economic burden [Citation14]. Many other efforts have been made in the assessment of anti-viral drugs to cure the rabies patients, but there is no effective treatment [Citation15,Citation16]. Given the inactivated human rabies vaccines were poor immunogenic and did not induce strong inflammatory response required for effective T- and B- cells mediated responses [Citation17]. The complicated immunity programmes of the inactivated human rabies vaccine also increased the cost of immunization. Therefore, it is urgent to develop a new reduced-dose, safe, and effective rabies vaccine to simplify the rabies vaccination programme and lessen costs.
Recently, the adeno-associated virus vectors have been proved to be an efficient platform for delivering biological countermeasures against pandemic and biological threats [Citation18,Citation19]. In 2012, the European Medicines Agency (EMA) approved Glybera, an AAV-based gene therapy drug, for the treatment of inherited lipoprotein lipase deficiency (LPLD) [Citation20]. In 2017, the Food and Drug Administration (FDA) approved Spark Therapeutics LUXTURNA, a recombinant adeno-associated virus serotype 2 of rAAV2-hRPE65 expressing human retinal pigment epithelium 65 kDa protein to apply in treatment of patients with confirmed biallelic RPE65 mutation-associated retinal dystrophy [Citation21,Citation22]. The AAV vectors have been also widely used to deliver viral antigen or antibody genes in vivo to cope with the viral infectious diseases, such as human immunodeficiency virus (HIV) [Citation23], hepatitis B virus (HBV) [Citation24], hepatitis C virus (HCV) [Citation25], nipah virus (NiV) and hendra virus (HeV) [Citation26], influenza A virus subtype (H1N1) [Citation27], and dengue virus (DENV) [Citation28].
The AAV serotype 9 vectors have been frequently observed to undergo robust axonal transport in vivo and could penetrate the blood–brain barrier, which was widely applied for gene delivery in different tissues [Citation29,Citation30]. The RABV G protein is the only viral envelope glycoprotein, and is responsible for viral invasion, viral pathogenicity [Citation31], viral escape from the host immune responses [Citation32], and plays a critical role in inducing protective immune responses [Citation1]. To address a dose-reduced, safe, and effective rabies vaccine candidate, in the present study, a recombinant adeno-associated virus serotype 9 of AAV9-RABVG expressing a RABV G gene was generated and evaluated its immunogenicity in mice and NHPs. All those data suggested that the AAV9-RABVG would be a promising single dose, safe, and effective rabies vaccine candidate for PrEP in both animals and humans.
Materials and methods
Ethics statement
All the mice studies were carried out strictly in accordance with prior approval from the Ethics Committee of Preventive Medicine of Shandong University (NO. 20160301). The Cynomolgus Macaques (Macaca fascicularis) studies were conducted with prior approval from the Animal Ethics Committee of Institute of Materia Medical, Shandong Academy of Medical Sciences (NO. AEC2018-006).
Plasmids, viruses, cells and antibodies
The plasmids of pAV-CMV-P2A-GFP, pAAV-rep/cap and Ad helper vector were purchased from Vigene biotechnology Co., Ltd. The BHK-21 cells and 293T cells were both cultured in Dulbecco’s Modified Eagle Medium (DMEM, Gibco, USA) supplemented with 10% fetal bovine serum (FBS, Gibco, Australia). Rabies virus CVS-11 strain (GenBank No. GQ918139.1) and street virus HuNPB3 strain were propagated in BHK-21 cells. The mouse anti-RABV G monoclonal antibody was purchased from Millipore (Massachusetts, USA). The Alexa Fluor 488-conjugated goat anti-mouse IgG (H+L) was purchased from Proteintech (Abcam, UK). The donkey anti-mouse IgG H&L (HRP) was purchased from Abcam (Maharashtra, India). The antibodies of APC-CD19, FITC-CD40, PE-Cy7-CD11c, PE-CD86, FITC-MHCI, and PE-MHCII used for flow cytometry were purchased from Franklin, USA.
Generation of recombinant AAV9-RABVG
The G gene of RABV SRV9 strain was cloned into the AAV plasmid of pAV-CMV-P2A-GFP between AsiS I and Mlu I sites, and obtained pAV-CMV-RABVG-P2A-GFP. The 293T cells were co-transfected with pAV-CMV-RABVG-P2A-GFP, Ad Helper plasmid and pAAV-rep/cap, and incubated with replaced serum-free DMEM for 24 h at 37°C. Virus packages were completed at 72 h after transfection and recombinant virus AAV9-RABVG was obtained.
Indirect immunofluorescence (IFA) and immunoblot
The 293T cells in a 6-well plate were infected with AAV9-RABVG or AAV9-GFP at a multiple of infection (MOI) of 105, respectively. The cells inoculated with DMEM were the mock cells. As for IFA, the cells were fixed with 80% ice-cold acetone for 12 h at −20°C after being cultured for 72 h at 37°C, and then stained with primary antibody of mouse anti-RABV G monoclonal antibody (Millipore, USA) and second antibody of Alexa Fluor 488-conjugated goat anti-mouse IgG (H + L) (Proteintech, China). The fluorescent signals were observed under an inverted fluorescence microscope (Olympus, IX71, Japan). As for immunoblot, the 293T cells infected with AAV9-RABVG, AAV9-GFP, or mock, respectively, were lysed in 1 ml RIPA (Solarbio, China) per 106 cells with 0.5 mM proteinase inhibition of Phenylmethyl sulphonyl Fluoride (PMSF). The supernatants of cell lysates were separated by a 10% SDS-PAGE and transferred onto polyvinylidene fluoride (PVDF) membrane (Millipore, USA). The membrane was incubated with primary antibody of mouse anti-RABV G monoclonal antibody (Millipore, USA) and secondary antibody of donkey anti-mouse IgG H&L (HRP) (Abcam, UK), and visualized by the enhanced chemiluminescent (ECL) HRP substrate (Thermo, USA).
Immunity studies in mice
The 6–8-week old female BALB/c mice were purchased from the Shandong University Laboratory Animal Center (Jinan, China), and were divided randomly into 3 groups. The grouped mice were vaccinated with 2 × 1011 v.p. AAV9-RABVG or AAV9-GFP in 100 μl by intramuscularly injection (i.m.). The mock mice were inoculated i.m. with 100 μl DMEM. Body weights and clinical signs were monitored daily for 21 days post immunity (p.i.) for the safety observation of AAV9-RABVG. The blood samples were collected from orbital venous plexus at 0, 2, 4, 8, 12, 24 weeks p.i.. Six mice per group were challenged i.m. with 50 IMLD50 of HuNPB3 strain in skeletal muscle of opposite hind legs at 3 weeks p.i., and observed for 21 days for clinical signs of rabies and survivals.
Immunity studies in Cynomolgus Macaques
The twelve 3-year-old female Cynomolgus Macaques were purchased from Guangdong Landau Biotechnology Co., Ltd and divided randomly into three groups. The Cynomolgus Macaques were intragluteally injected 1 × 1013 v.p. AAV9-RABVG or AAV9-GFP in 1 ml, the mock group was injected 1 ml DMEM. The blood samples were collected from subcutaneous veins of hind limbs at 0, 2, 4, 8, 12, 24, 36, and 52 weeks p.i.
Fluorescent antibody virus neutralization (FAVN) test
The titres of rabies virus neutralizing antibodies (RVNAs) were determined with fluorescent antibody virus neutralization (FAVN) assays as described previously [Citation33, Citation34]. Briefly, 2-fold dilutions of the heat-inactivated sera were incubated with 150 TCID50 of RABV for 1 h at 37°C in 96-well plates. Then, 50 µl Vero cells (2 × 105/ml) were added to each well and cultured in a 5% CO2 incubator at 37°C for 48 h. The cells were fixed with 80% ice-cold acetone, and then stained with FITC labelled mouse anti-RABV N monoclonal antibody (Fujirebio, Japan). The fluorescent signals were observed by an inverted fluorescence microscope, and the titres of RVNAs were calculated as IU/ml by comparison with the WHO standard.
Flow cytometry assay
The inguinal lymph nodes or spleens of the vaccinated BALB/c mice (n = 4 in each group) were collected at the indicated time point. Single-cell suspension of 106 cells/ml was prepared in RPMI-1640 (Gibco, USA) at 1-week p.i. with 0.2% FBS by pressing through the nylon filters, and then stained with antibodies APC-CD19, FITC-CD40, PE-Cy7-CD11c, PE-CD86, FITC-MHCI and PE-MHCII at 4°C for 30 min. Data were collected by Beckman Coulter Cytoflex S flow cytometer (USA) and analyzed using the CytExpert 2.0 software (Beckman Coulter, USA).
Intracellular cytokine staining
Intracellular cytokine staining was performed to quantitate the cytokine secretions in CD4+ and CD8+ T cells from spleens of the immunized mice. At 2 weeks p.i., single-cell suspensions of 2 × 106 cells/ml from spleens of four mice in each group were stimulated with inactivated SRV9 (10 μg/ml) and incubated with a protein transport inhibitor BD cytofix/cytoperm (BD Biosciences, USA) for 6 h at 37°C. And then the cells were stained with antibody of FITC-CD4 and PE-CD8a (BD Biosciences, USA) for cell surface antigens and stained with APC-IL-4 and PE-Cy7-IFN-γ for intracellular cytokines for 30 min at 4°C. The positive signals of lymphocyte population were analyzed by flow cytometer (CytoFLEXS, Beckman Coulter, USA).
ELISA assay
The secretions of IL-2, IL-4, IL-10, and IFN-γ in splenocytes from the immunized mice were detected in the presence of the specific stimulant. At 2 weeks p.i., single-cell suspensions (2 × 106 cells/ml) from spleens of four mice per group were stimulated with inactivated and purified SRV9 (10 μg/ml) in 24-well plate at 37°C for 60 h. The supernatants were collected and measured by mouse IL-2, IL-4, IL-10, and IFN-γ ELISA kit (Mabtech, Sweden) according to manufacturer instructions.
IFN-γ and IL-4 enzyme-linked immunospot (ELISpot) assays
At 2 weeks p.i., single cell suspensions (2.5 × 106 cells/ml) of spleens collected from the immunized mice (n = 4) were prepared in 96-well ELISpot plates. The lymphocytes were stimulated with the inactivated and purified SRV9 (10 μg/ml) for 36 h at 37°C. Secreted IFN-γ or IL-4 was quantitated using ELISpot assays (Mabtech, Sweden) according to the manufacturer’s instructions. The resultant spot forming cells (SFCs) were counted with an ELISpot reader (AID ELISpot reader-iSpot, AID GmbH, GER).
Statistical analysis
Data are expressed as the mean ± standard deviation (SD). The statistical difference between the data in each group was analyzed by one-way ANOVA in SPSS 22.0 software (SPSS Inc., Chicago, IL, USA). Statistical significance was considered at *p < 0.05, ** p < 0.01, ***p < 0.001.
Results
Generation and characteristic of AAV9-RABVG
The G gene of RABV SRV9 strain was cloned into the pAV-CMV-P2A-GFP at AsiS I and Mlu I sites and resulted in pAV-CMV-RABVG-P2A-GFP. By co-transfection with pAV-CMV-RABVG-P2A-GFP, Ad Helper plasmid, and pAAV-rep/cap in 293T cells, the recombinant virus AAV9-RABVG was obtained ((A). The GFP was expressed in 293T cells infected with both AAV9-GFP ((C)) and AAV9-RABVG ((D)). The green fluorescence signals specific to the RABV G protein were only observed in AAV9-RABVG infected 293T cells ((G)), and no fluorescence was seen in AAV9-GFP ((F)) or mock ((E)) infected 293T cells. In (H), a 70 kDa band of RABV G protein was detected in AAV9-RABVG infected 293T cells, while no band was seen in AAV9-GFP or mock infected 293T cells. Thus, the recombinant AAV9-RABVG was successfully generated and could express the RABV G protein in vitro.
Figure 1. Constructions and identifications of the recombinant virus AAV9-RABVG in infected 293T cells. The G gene of RABV SRV9 strain was cloned into the AAV plasmid of pAV-CMV-P2A-GFP between AsiS I and Mlu I sites, and resulted in pAV-CMV-RABVG-P2A-GFP. The recombinant virus AAV9-RABVG (A) was obtained by co-transfection with pAV-CMV-RABVG-P2A-GFP, Ad Helper plasmid and pAAV-rep/cap in 293T cells. The 293T cells were infected with mock (B and E), AAV9-GFP (C and F) or AAV9-RABVG (D and G) at an MOI of 105, respectively, and the eGFP expressions were observed at 72 h post infection in AAV9-GFP (C), AAV9-RABVG (D) or mock (B) infected 293T cells (×200) with a fluorescent microscope before fixed. Then, the cells were fixed with 80% ice-cold acetone, and stained with primary antibody of mouse anti-RABV G monoclonal antibody and second antibody of Alexa Fluor 488-conjugated goat anti-mouse IgG (H + L). The fluorescent signals specific to the RABV G protein in 293T cells (×200) infected with mock (E), AAV9-GFP (F) or AAV9-RABVG (G) was observed with a fluorescent microscope. The specific G protein band was detected by western blot in the AAV9-GFP infected 293T cells (H).
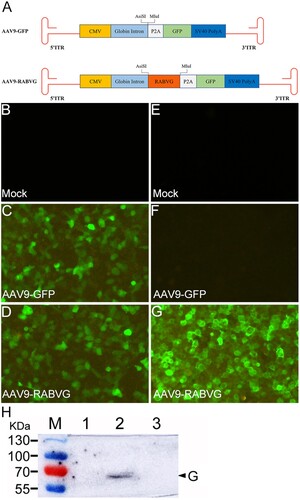
AAV9-RABVG induced robust and long-term protective humoral responses in mice
The body weight changes of three groups of the vaccinated BALB/c mice were monitored daily for 21 days to assess the safety of AAV9-RABVG. As shown in (B), compared to the mock group, the mice inoculated with AAV9-RABVG or AAV9-GFP showed slightly body weight changes. All the three grouped mice showed no abnormal behaviour or neurological signs during the observation period. As shown in (D), the titres of RVNAs in sera from the AAV9-RABVG vaccinated mice reached 13.66 IU/ml at 2 weeks post a single dose immunity, and demonstrated a persistent increase, and peaked 215.76 IU/ml at 8 weeks p.i.. The titres of RVNAs still maintained over 100 IU/ml that was much higher than 0.5 IU/ml the minimum adequately protective antibody titres recommended by WHO at 24 weeks p.i.. No RVNAs were detected in the AAV9-GFP or mock-inoculated mice. All the mice vaccinated with AAV9-RABVG survived without any rabies signs for 21 days after challenge ((C)), but the mice inoculated with AAV9-GFP or mock died of rabies. Unsurprisingly, the AAV9-RABVG provided adequate protective humoral immune responses against lethal rabies infection. However, it was interesting that the AAV9-RABVG induced a significantly higher and long-term RABV-specific neutralizing antibodies maintained in mice. Therefore, we investigated the molecular mechanism of this antibody production and maintenance.
Figure 2. Immunogenicity effects of AAV9-RABVG in BALB/c mice. (A) Mouse study design. Female, 6–8 weeks old, BALB/c mice (n = 50 in each group) were inoculated i.m. with 100 μl of 2 × 1011 v.p. AAV9-GFP or AAV9-RABVG, respectively, and the mock mice were inoculated i.m. with 100 μl DMEM. 3 weeks later, the vaccinated mice were challenged with 50 IMLD50 of HuNPB3 strain in skeletal muscle of opposite hind legs. (B) The weight change percentages of the mice (n = 6 in each group) within 3 weeks p.i.. (C) Survival curves of the vaccinated mice after challenging (n = 6 in each group) with the street RABV HuNPB3 strain. (D) The titres of RVNAs in serum samples (n = 6 in each group at each time point) were detected respectively at 2, 4, 8, 12, and 24 weeks p.i.. (*p < 0.05, **p < 0.01, ns: no statistically significant)
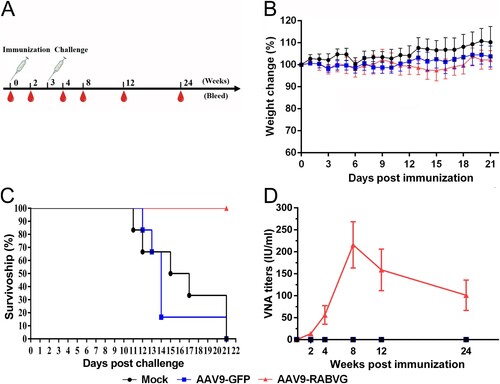
AAV9-RABVG activated more DC and B cells.
More CD11c+ CD80+, CD11c+ CD86+, CD11c+ MHC II+ DC cells in lymph nodes of AAV9-RABVG grouped mice were significantly detected than those of the mock grouped mice ((B,C)). These results demonstrated that AAV9-RABVG activated more DC cells and the expression of MHC II+ molecules to present viral-specific antigens. The percentages of CD19+ CD40+ B cells in inguinal lymph nodes ((B,C)) and in spleen ((E,F)) from the AAV9-RABVG grouped mice were significantly higher than those of the mock grouped mice at 1-week p.i., indicated that the more B cells were significantly activated by AAV9-RABVG.
Figure 3. More DCs in the inguinal lymph nodes from the AAV9-RABVG vaccinated mice were activated. At 1 week p.i., inguinal lymph nodes were collected from the vaccinated BALB/c mice (n = 6 in each group) and single cell suspensions (106 cells/ml) were stained with antibodies of DCs activation markers and analyzed via flow cytometry. (A) The gating strategies for analyzing the DCs. (B) Presentative flow cytometric plots for measuring the percentages of recruited and/or activated DCs. (C) Analyses for activated DCs (CD11c+ CD80+, CD11c+ CD86+, CD11c+ MHCI+, CD11c+ MHCII+). (*p < 0.05, ***p < 0.001, ns: no statistically significant).
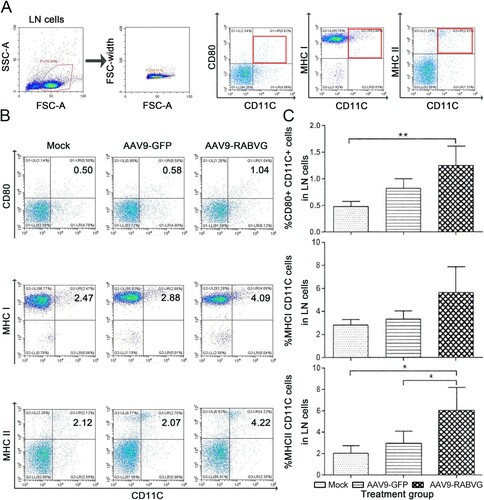
Figure 4. More B cells in inguinal lymph nodes and spleen were recruited and/or activated by AAV9-RABVG. At 1 week p.i., the inguinal lymph nodes and spleen were collected from the vaccinated BALB/c mice (n = 6 in each group) and single cell suspensions (106 cells/ml) were stained with antibodies of B cells activation markers and analyzed via flow cytometry. The gating strategies for analyzing the B cells of inguinal lymph nodes (A) or spleen (D). Presentative flow cytometric plots for measuring recruitment and/or activations of B cells of inguinal lymph nodes (B) or spleen (E). Analyses of activated B cells (CD19+ CD40+) of inguinal lymph nodes (C) or spleen (F). (*p < 0.05, **p < 0.01, ***p < 0.001)
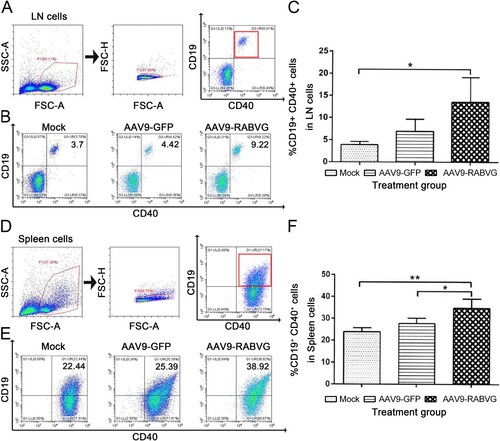
AAV9-RABVG induced both Th1 and Th2 immune responses
Compared to AAV9-GFP and mock groups, the AAV9-RABVG elicited significantly more IFN-γ-secreting CD4+ and CD8+ T cells and IL-4-secreting CD4+ T cells ((B,C)), and enhanced activations of IFN-γ- or IL-4- secreting CD4+ and CD8+ T cells at 2 weeks p.i. Meanwhile, the AAV9-RABVG induced significantly higher levels of IL-2, IL-4, IL-10, IL-1β, TNF-α, and IFN-γ secretion by spleen cells than those of the AAV9-GFP and mock mice at 2 weeks p.i. (). Significantly more IFN-γ ((A,B)) and IL-4 ((C,D)) SFCs in spleens collected from the AAV9-RABVG vaccinated mice were recruited at 2 weeks p.i. All together, the AAV9-RABVG induced high levels of both Th1 (IL-2 and IFN-γ) and Th2 cytokines (IL-4 and IL-10) and AAV9-RABVG induced both Th1 and Th2 cell-mediated immune responses in mice.
Figure 5. Intracellular cytokine detections. At 2 weeks p.i., single cell suspensions of 2×106 cells/ml from spleens (n = 4 in each group) were stimulated with inactivated and purified SRV9 (10 μg/ml), and incubated with a protein transport inhibitor for 6 h at 37°C. And then the cells were stained with antibodies of FITC-CD4 and PE-CD8a for cell surface antigens and stained with APC-IL-4 and PE-Cy7-IFN-γ for intracellular cytokines for 30 min at 4°C. The positive signals of lymphocyte population were analyzed by flow cytometer. (A) The gating strategies for analyzing the IFN-γ and IL-4 secreting CD4+ and CD8+ cells. (B) Presentative flow cytometric plots for measuring the percentages of IFN-γ and IL-4 secreting CD4+ and CD8+ T cells. (C) Analyses for positive IFN-γ and IL-4 secreting CD4+ and CD8+ T cells. (*p < 0.05, **p < 0.01, ***p < 0.001).
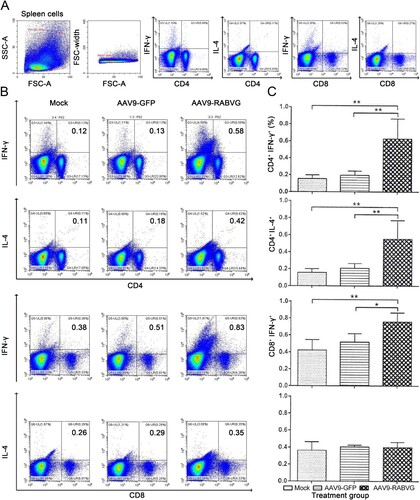
Figure 6. RABV specific cytokines responses were induced by AAV9-RABVG in mice with ELISA assay. At 2 weeks p.i., single cell suspensions of 2 × 106 cells/ml from spleens were stimulated by inactivated and purified RABV, and the secretion of IFN-γ (A), IL-2 (B), IL-4 (C), IL-10 (D), IL-1β (E) and TNF-α (F) was detected by ELISA assay. (*p < 0.05, **p < 0.01, and ***p < 0.001).
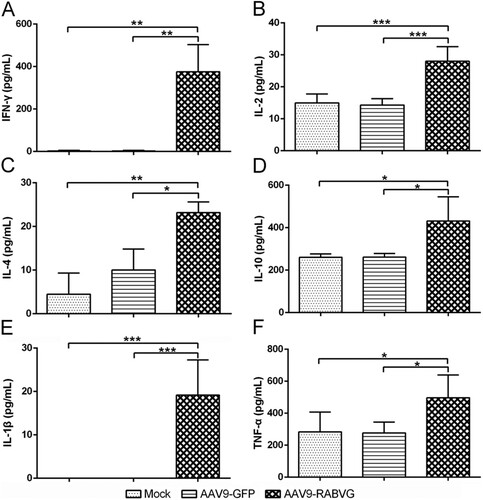
Figure 7. RABV specific T cell-mediated immune responses by AAV9-RABVG in mice. At 2 weeks p.i., single cell suspensions of 2.5 × 106 cells/ml from spleens were stimulated by inactivated and purified RABV, and the RABV specific IFN-γ or IL-4 SFCs was quantitated using ELISpot assays (Mabtech, Sweden). (A) RABV specific IFN-γ SFCs. (C) RABV specific IL-4 SFCs. Representative images for IFN-γ or IL-4 in each group were shown below the graph (B and D). (*p < 0.05, **p < 0.01, and ***p < 0.001).
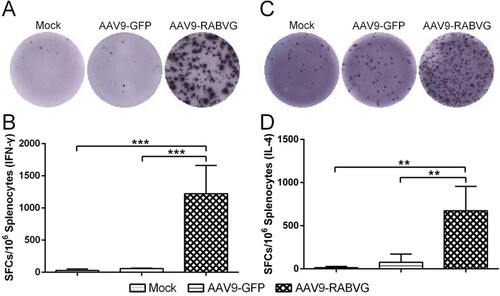
AAV9-RABVG induced robust and long-lasting RVNA response in Cynomolgus Macaques
Furtherly, the immunogenicity of AAV9-RABVG was evaluated in Cynomolgus Macaques, and the RVNA titres were measured with the FAVN assay. As shown in , the RVNA titres of the serum samples from the AAV9-RABVG vaccinated Cynomolgus Macaques were detectable at 2 weeks p.i., and then increased rapidly and continuously, and peaked 160.365 IU/ml at 8 weeks p.i. The RVNA titres remained above 31.525 IU/ml at 52 weeks p.i., which indicated that the AAV9-RABVG elicited a robust and long term over one-year antibody maintenances. No RVNAs were detected in theAAV9-GFP and mock grouped Cynomolgus Macaques.
Figure 8. RABV specific neutralizing antibodies responses in Cynomolgus Macaques. Twelve 3-year-old female Cynomolgus Macaques were divided randomly into three groups, and were intragluteally injected with 1 ml of 1 × 1013 v.p. AAV9-RABVG or AAV9-GFP. The mock group was injected with 1 ml DMEM as control. The titres of RVNAs in serum (n = 4) were detected at 0, 2, 4, 8, 12, 24, 36, 52 weeks p.i., respectively.
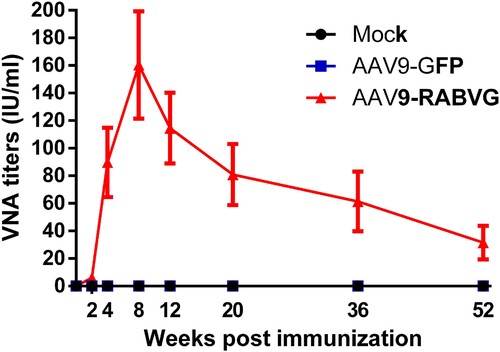
Discussion
The RABV G protein is the only viral surface glycoprotein and plays a critical role in eliciting the protective humoral immune responses. The WHO have recommended that the titres of RVNAs over 0.5 IU/ml are adequate for protection against a lethal RABV infection [Citation9]. Many studies proved that the chemokine, inflammatory factor, and cell-associated immune responses, also played important roles in improving rabies vaccines efficacy and virus clearance [Citation35]. The inactivated human rabies vaccine contains no adjuvant, and needs 3- or 4-times booster immunizations. Several avirulent recombinant RABV expressing GM-CSF, MIP-1α, IL-7, HMGB1, CXCL13 were generated to develop an affordable efficient rabies vaccine, but the live attenuated rabies vaccine was still at risk for unforeseen occurrence and difficult to be accepted by the public [Citation2]. Therefore, it is still necessary to explore a genetically engineered, dose-reduced, safety, and effective rabies vaccine.
AAV vectors have a relatively long history used for gene therapy applications, and only recently emerged in the vaccine field [Citation36, Citation37]. For many years, the AAV vectors have been considered as low immunogenic vectors due to inability of inducing long-term expression of non-self proteins and generating humoral or cellular immune responses by i.m. injections [Citation38, Citation39]. The specific immune responses to AAV vectored antigen depended on its accessibility into different local antigen-presenting cells [Citation40], and associated with immunization route and transgene type [Citation41]. Controversial observations were subsequently reported that AAV vectors induced CD8+ T cell-mediated immune response to transgenic antigen by i.m., intranasal, intraperitoneal, and subcutaneous injection in mice or NHPs [Citation36]. AAV2/rh32.33, expressing a truncated envelope protein (79E) of DENV, induced long-lasting antibody response that was still detectable at 20 weeks after immunization [Citation42]. A single dose of rAAV5L1h, encoding the major capsid protein L1 (L1h) from the human papillomavirus type 16 (HPV16), was sufficient to induce high titres of L1-specific serum antibodies, as well as mucosal antibodies in vaginal washes, and the seroconversion was maintained for at least 1 year [Citation43]. In this study, AAV9-RABVG induced specific, robust, and long-term RVNA responses in mice and NHPs. The titres of RVNAs maintained over 100 IU/ml at 24 weeks p.i. in AAV9-RABVG vaccinated mice, and stably maintained 31.525 IU/ml at 52 weeks p.i. in Cynomolgus Macaques. The titre of RVNA ≥0.5 IU/ml in sera was considered to be sufficient for protection from rabies infections, and the RVNAs are mainly specific to its surface G protein [Citation44]. Thus, the AAV9 was a high-efficiency vector for gene delivery of the RABV G in mice and in NHPs.
DCs take up and present antigens to both CD8 and CD4 T cells [Citation45]. Recent study uncovered that the ecto-domains of the RABV G protein mediated its DCs bindings and activations [Citation1]. In this study, significantly more DCs of inguinal lymph nodes from the AAV9-RABVG vaccinated mice were activated, and significantly more MHC II molecular were expressed in DCs, where the RABV G protein was processed, to present antigen to CD4+ T cells. Meanwhile, significantly more MHCI molecular was detected, suggesting that the RABV G protein was also cross-presented by MHC I to CD8+ T cells triggering cytotoxic T cell responses (CTL). However, AAV9-GFP did not elicit significantly more DCs and B cells. We speculated that these differences may be caused by different exogenous proteins expressed by AAVs. The G expressed by AAV9-RABVG is the main antigenic components of RABV, which can induce strong immune responses. Therefore, the recombinant AAV9-RABVG strongly activated or recruited the DCs and T lymphocytes in the periphery blood from the immunized mice.
Naïve CD4+ T cells are activated after interaction with antigen-MHC complex and differentiate into the classical Th1 and Th2 cells [Citation46, Citation47]. Th1 cells mainly secreted IFN-γ and IL-2, while the key effector-cytokines IL-10 and IL-4 polarized naïve T cells to Th2 cells [Citation48]. CD4+ T cells enter B cell follicles and help B cells product antibodies [Citation49]. CD8+ T cells are responsible for the clearance of RABV by controlling infection together with antibodies by enhancing IFN-γ production [Citation13]. In this study, significantly more IFN-γ-secreting CD4+ and CD8+ T cells, and IL-4-secreting CD4+ T cells specific to RABV G protein were detected and induced an enhanced CTL effect in AAV9-RABVG vaccinated mice. In addition, IL-2 and IFN-γ indicating Th1 type cytokines, and IL-4 and IL-10 indicating Th2 type cytokines, were significantly secreted by splenocytes from AAV9-RABVG vaccinated mice. All these findings indicated that AAV9-RABVG strongly elicited both Th1 and Th2 cell-associated immune responses in mice, which indirectly contributed to the understanding of the robust and one year long-lasting antibodies sustains in Cynomolgus Macaques.
The main issue of AAV vectors application is that of the pre-existing immunity in the human population to the wild-type AAV serotype 2. Employing alternative serotypes of AAV vectors may circumvent this problem [Citation50]. In conclusion, the recombinant AAV9-RABVG inducing robust and long-lasting antibody responses in mice and in NHPs, proved a great potential to develop as a new reduced-dose, safety, and high-efficiency rabies vaccine candidate in humans and animals.
Author contributions
The study was conceptualized and funding acquisition by X.Z. The biological experiments and analyses were carried out by C.S., L.T., W.Z. and Y.Z. The data visualization and analyses were conducted by L.L., W.L., and P.S. This project was administrated by Y.S. and X.Z.X. The manuscript was written by X.H.X, and reviewed, commented, and approved by all the authors.
Disclosure statement
No potential conflict of interest was reported by the author(s).
Additional information
Funding
References
- Huang J, Zhang Y, Huang Y, et al. The ectodomain of rabies virus glycoprotein determines dendritic cell activation. Antiviral Res. 2017 May;141:1-6. doi:10.1016/j.antiviral.2017.01.022 PubMed PMID: 28161580.
- El-Sayed A. Advances in rabies prophylaxis and treatment with emphasis on immunoresponse mechanisms. Int J Vet Sci Med. 2018 Jun;6(1):8–15. doi:10.1016/j.ijvsm.2018.05.001 PubMed PMID: 30255072; PubMed Central PMCID: PMCPMC6149183.
- Potratz M, Zaeck LM, Weigel C, et al. Neuroglia infection by rabies virus after anterograde virus spread in peripheral neurons. Acta Neuropathol Commun. 2020 Nov 23;8(1):199, doi:10.1186/s40478-020-01074-6 PubMed PMID: 33228789; PubMed Central PMCID: PMCPMC7684951.
- Zhenfang F. Rabies and rabies- research past, present and future. Vaccine. 1997;15:20–24. doi:10.1016/S0264-410X(96)00122-3
- Wirblich C, Schnell MJ. Rabies virus (RV) glycoprotein expression levels are not critical for pathogenicity of RV. J Virol. 2011 Jan;85(2):697–704. doi:10.1128/JVI.01309-10 PubMed PMID: 21068252; PubMed Central PMCID: PMCPMC3020019.
- World Health Organization. WHO expert consultation on rabies: third report. Geneva: WHO technical report series; 2018; 1012.
- Fooks AR, Cliquet F, Finke S, et al. Rabies. Nat Rev Dis Primers. 2017 Nov 30;3:17091, doi:10.1038/nrdp.2017.91 PubMed PMID: 29188797.
- Davis BM, Rall GF, Schnell MJ. Everything you always wanted to know about rabies virus (but were afraid to ask). Annu Rev Virol. 2015;2(1):451–471. doi:10.1146/annurev-virology-100114-055157.
- Du Pont V, Plemper RK, Schnell MJ. Status of antiviral therapeutics against rabies virus and related emerging lyssaviruses. Curr Opin Virol. 2019 Apr;35:1–13. doi:10.1016/j.coviro.2018.12.009 PubMed PMID: 30753961; PubMed Central PMCID: PMCPMC6556400.
- Davlin SL, Vonville HM. Canine rabies vaccination and domestic dog population characteristics in the developing world: a systematic review. Vaccine. 2012 May 21;30(24):3492–3502. doi:10.1016/j.vaccine.2012.03.069 PubMed PMID: 22480924.
- Franka R, Smith TG, Dyer JL, et al. Current and future tools for global canine rabies elimination. Antiviral Res. 2013 Oct;100(1):220–225. doi:10.1016/j.antiviral.2013.07.004 PubMed PMID: 23871797.
- Hicks DJ, Fooks AR, Johnson N. Developments in rabies vaccines. Clin Exp Immunol. 2012 Sep;169(3):199–204. doi:10.1111/j.1365-2249.2012.04592.x PubMed PMID: 22861358; PubMed Central PMCID: PMCPMC3444995.
- Katz ISS, Guedes F, Fernandes ER, et al. Immunological aspects of rabies: a literature review. Arch Virol. 2017 Nov;162(11):3251–3268. doi:10.1007/s00705-017-3484-0 PubMed PMID: 28726129.
- Both L, Banyard AC, van Dolleweerd C, et al. Passive immunity in the prevention of rabies. Lancet Infect Dis. 2012;12(5):397–407. doi:10.1016/S1473-3099(11)70340-1.
- Tarantola A. Four thousand years of concepts relating to rabies in animals and humans. Its Prevention and Its Cure. Tropical Medicine and Infectious Disease. 2017;2(4):5, doi:10.3390/tropicalmed2020005.
- Banyard AC, Mansfield KL, Wu G, et al. Re-evaluating the effect of Favipiravir treatment on rabies virus infection. Vaccine. 2019 Aug 2;37(33):4686–4693. doi:10.1016/j.vaccine.2017.10.109 PubMed PMID: 29132993.
- McGettigan JP. Experimental rabies vaccines for humans. Expert Rev Vaccines. 2010 Oct;9(10):1177–1186. doi:10.1586/erv.10.105 PubMed PMID: 20923268; PubMed Central PMCID: PMCPMC3072237.
- Verdera HC, Kuranda K, Mingozzi F. AAV vector immunogenicity in humans: a long journey to successful gene transfer. Mol Ther. 2020 Mar 4;28(3):723–746. doi:10.1016/j.ymthe.2019.12.010 PubMed PMID: 31972133; PubMed Central PMCID: PMCPMC7054726.
- Nidetz NF, McGee MC, Tse LV, et al. Adeno-associated viral vector-mediated immune responses: understanding barriers to gene delivery. Pharmacol Ther. 2020 Mar;207:107453, doi:10.1016/j.pharmthera.2019.107453 PubMed PMID: 31836454; PubMed Central PMCID: PMCPMC6980784.
- Glybera-product-information. Summary of product characteristics. European Medicines Agency. 2012;1–35.
- Bi L. (2017). Summary-Basis-for-Regulatory-Action – LUXTURNA. . Food and drug adminstration.
- Weleber RG, Pennesi ME, Wilson DJ, et al. Results at 2 years after gene therapy for RPE65-deficient leber congenital amaurosis and severe early-childhood-onset retinal dystrophy. Ophthalmology. 2016 Jul;123(7):1606–1620. doi:10.1016/j.ophtha.2016.03.003 PubMed PMID: 27102010.
- Xin K-Q, Urabe M, Yang J, et al. A novel recombinant adeno-associated virus vaccine induces a long-term humoral immune response to human immunodeficiency virus. Hum Gene Ther. 2001;12(9):1047–1061. doi:10.1089/104303401750214276
- Kan F, Ye L, Yan T, et al. Proteomic and transcriptomic studies of HBV-associated liver fibrosis of an AAV-HBV-infected mouse model. BMC Genomics. 2017 Aug 22;18(1):641, doi:10.1186/s12864-017-3984-z PubMed PMID: 28830339; PubMed Central PMCID: PMCPMC5568174.
- Yang X, Marcucci K, Anguela X, et al. Preclinical evaluation of an anti-HCV miRNA cluster for treatment of HCV infection. Mol Ther. 2013 Mar;21(3):588–601. doi:10.1038/mt.2012.247 PubMed PMID: 23295950; PubMed Central PMCID: PMCPMC3589168.
- Ploquin A, Szecsi J, Mathieu C, et al. Protection against henipavirus infection by use of recombinant adeno-associated virus-vector vaccines. J Infect Dis. 2013 Feb 1;207(3):469–478. doi:10.1093/infdis/jis699 PubMed PMID: 23175762; PubMed Central PMCID: PMCPMC7107322.
- Limberis MP, Adam VS, Wong G, et al. Intranasal antibody gene transfer in mice and ferrets elicits broad protection against pandemic influenza. Sci Transl Med. 2013 May 29;5(187):187ra72, doi:10.1126/scitranslmed.3006299. PubMed PMID: 23720583; PubMed Central PMCID: PMCPMC4596530.
- Magnani DM, Ricciardi MJ, Bailey VK, et al. Dengue virus evades AAV-mediated neutralizing antibody prophylaxis in rhesus monkeys. Mol Ther. 2017 Oct 4;25(10):2323–2331. doi:10.1016/j.ymthe.2017.06.020 PubMed PMID: 28750738; PubMed Central PMCID: PMCPMC5628771.
- Samaranch L, Salegio EA, San Sebastian W, et al. Adeno-associated virus serotype 9 transduction in the central nervous system of nonhuman primates. Hum Gene Ther. 2012 Apr;23(4):382–389. doi:10.1089/hum.2011.200 PubMed PMID: 22201473; PubMed Central PMCID: PMCPMC3327605.
- Samaranch L, Perez-Canamas A, Soto-Huelin B, et al. Adeno-associated viral vector serotype 9-based gene therapy for niemann-pick disease type A. Sci Transl Med. 2019 Aug 21;11(506), doi:10.1126/scitranslmed.aat3738. PubMed PMID: 31434754; PubMed Central PMCID: PMCPMC7285630.
- Astray RM, Jorge SA, Pereira CA. Rabies vaccine development by expression of recombinant viral glycoprotein. Arch Virol. 2017 Feb;162(2):323–332. doi:10.1007/s00705-016-3128-9 PubMed PMID: 27796547.
- Wang ZW, Sarmento L, Wang Y, et al. Attenuated rabies virus activates, while pathogenic rabies virus evades, the host innate immune responses in the central nervous system. J Virol. 2005 Oct;79(19):12554–12565. doi:10.1128/JVI.79.19.12554-12565.2005 PubMed PMID: 16160183; PubMed Central PMCID: PMCPMC1211539.
- Cliquet F, Aubert M, Sagne L. Development of a fluorescent antibody virus neutralisation test (FAVN test) for the quantitation of rabies-neutralising antibody. J Immunol Methods. 1998 Mar 1;212(1):79–87. PubMed PMID: 9671155; eng. doi:10.1016/S0022-1759(97)00212-3
- Tian D, Luo Z, Zhou M, et al. Critical role of K1685 and K1829 in the large protein of rabies virus in viral pathogenicity and immune evasion. J Virol. 2015;90(1):232–244. doi:10.1128/JVI.02050-15 PubMed PMID: 26468538; eng.
- Wang Z, Li M, Zhou M, et al. A novel rabies vaccine expressing CXCL13 enhances humoral immunity by recruiting both T Follicular Helper and Germinal Center B Cells. J Virol. 2017 Feb 1;91(3), doi:10.1128/JVI.01956-16. PubMed PMID: 27852854; PubMed Central PMCID: PMCPMC5244327.
- Nieto K, Salvetti A. AAV vectors vaccines against Infectious diseases. Front Immunol. 2014;5:5, doi:10.3389/fimmu.2014.00005 PubMed PMID: 24478774; PubMed Central PMCID: PMCPMC3896988.
- Hanlon KS, Meltzer JC, Buzhdygan T, et al. Selection of an efficient AAV vector for robust CNS transgene expression. Mol Ther Methods Clin Dev. 2019 Dec 13;15:320–332. doi:10.1016/j.omtm.2019.10.007 PubMed PMID: 31788496; PubMed Central PMCID: PMCPMC6881693.
- Fisher KJ, Jooss K, Alsto J, et al. Recombinant adena-associated virus for muscle directed gene therapy. Nature Publishing Group. 1997;3(3):306–312.
- Jooss K, Yang Y, Fisher KJ, et al. Transduction of dendritic cells by DNA viral vectors directs the immune response to transgene products in muscle fibers. J Virol. 1998 May;72(5):4212–4223. PubMed PMID: 9557710; PubMed Central PMCID: PMCPmc109650. eng. doi:10.1128/JVI.72.5.4212-4223.1998
- Sarukhan A, Soudais C, Danos O, et al. Factors influencing cross-presentation of non-self antigens expressed from recombinant adeno-associated virus vectors. J Gene Med. 2001 May-Jun;3(3):260–270. doi:10.1002/jgm.175. PubMed PMID: 11437331; eng.
- Ponnazhagan S. Adenoassociated virus vectors for genetic immunization. Immunol Res. 2002;26(1-3):247–253. doi:10.1385/IR:26:1-3:247 PubMed PMID: 12403362; eng.
- Li X, Cao H, Wang Q, et al. Novel AAV-based genetic vaccines encoding truncated dengue virus envelope proteins elicit humoral immune responses in mice. Microbes Infect. 2012 Sep;14(11):1000–1007. doi:10.1016/j.micinf.2012.05.002 PubMed PMID: 22626929.
- Kuck D, Lau T, Leuchs B, et al. Intranasal vaccination with recombinant adeno-associated virus type 5 against human papillomavirus type 16 L1. J Virol. 2006 Mar;80(6):2621–2630. doi:10.1128/JVI.80.6.2621-2630.2006 PubMed PMID: 16501072; PubMed Central PMCID: PMCPMC1395428.
- Yan L, Zhao Z, Xue X, et al. A bivalent human adenovirus type 5 vaccine expressing the rabies virus glycoprotein and canine distemper virus hemagglutinin protein confers protective immunity in mice and foxes. Front Microbiol. 2020;11:1070–1012. doi:10.3389/fmicb.2020.01070 PubMed PMID: 32612580; PubMed Central PMCID: PMCPMC7309451.
- Balan S, Saxena M, Bhardwaj N. Dendritic cell subsets and locations. Int Rev Cell Mol Biol. 2019;348:1–68. doi:10.1016/bs.ircmb.2019.07.004
- Mosmann TR, Cherwinski H, Bond MW, et al. Two types of murine helper T cell clone. I. Definition According to Profiles of Lymphokine Activities and Secreted Proteins. J Immunol. 1986;136(7):2348–2357.
- Luckheeram RV, Zhou R, Verma AD, et al. CD4(+)T cells: differentiation and functions. Clin Dev Immunol. 2012;2012:925135, doi:10.1155/2012/925135 PubMed PMID: 22474485; PubMed Central PMCID: PMC3312336.
- Durai V, Murphy KM. Functions of murine dendritic cells. Immunity. 2016 Oct 18;45(4):719–736. doi:10.1016/j.immuni.2016.10.010 PubMed PMID: 27760337; PubMed Central PMCID: PMCPMC5145312.
- Swain SL, McKinstry KK, Strutt TM. Expanding roles for CD4(+) T cells in immunity to viruses. Nat Rev Immunol. 2012 Jan 20;12(2):136–148. doi:10.1038/nri3152 PubMed PMID: 22266691; PubMed Central PMCID: PMCPMC3764486.
- Li C, Narkbunnam N, Samulski RJ, et al. Neutralizing antibodies against adeno-associated virus examined prospectively in pediatric patients with hemophilia. Gene Ther. 2012 Mar;19(3):288–294. doi:10.1038/gt.2011.90 PubMed PMID: 21697954.