ABSTRACT
The pandemic caused by severe acute respiratory syndrome coronavirus 2 (SARS-CoV-2) has resulted in significant morbidity and mortality worldwide. Despite a successful vaccination programme, the emergence of mutated variants that can escape current levels of immunity mean infections continue. Herein, we report the development of CT-P63, a broad-spectrum neutralizing monoclonal antibody. In vitro studies demonstrated potent neutralizing activity against the most prevalent variants, including Delta and the BA.1 and BA.2 sub-lineages of Omicron. In a transgenic mouse model, prophylactic CT-P63 significantly reduced wild-type viral titres in the respiratory tract and CT-P63 treatment proved efficacious against infection with Beta, Delta, and Omicron variants of SARS-CoV-2 with no detectable infectious virus in the lungs of treated animals. A randomized, double-blind, parallel-group, placebo-controlled, Phase I, single ascending dose study in healthy volunteers (NCT05017168) confirmed the safety, tolerability, and pharmacokinetics of CT-P63. Twenty-four participants were randomized and received the planned dose of CT-P63 or placebo. The safety and tolerability of CT-P63 were evaluated as primary objectives. Eight participants (33.3%) experienced a treatment-emergent adverse event (TEAE), including one grade ≥3 (blood creatine phosphokinase increased). There were no deaths, treatment-emergent serious adverse events, TEAEs of special interest, or TEAEs leading to study drug discontinuation in the CT-P63 groups. Serum CT-P63 concentrations rapidly peaked before declining in a biphasic manner and systemic exposure was dose proportional. Overall, CT-P63 was clinically safe and showed broad-spectrum neutralizing activity against SARS-CoV-2 variants in vitro and in vivo.
Introduction
The unprecedented levels of coronavirus disease 2019 (COVID-19) caused by the severe acute respiratory syndrome coronavirus 2 (SARS-CoV-2) has led to global morbidity and mortality [Citation1]. Novel therapies, including neutralizing monoclonal antibodies (mAb), have been developed for the prevention and treatment of COVID-19 [Citation2]. The primary target for mAbs has been the angiotensin-converting enzyme 2 (ACE2) receptor binding domain (RBD) of the SARS-CoV-2 spike protein, which is crucial for infectivity and pathogenesis [Citation2–4]. To date, several SARS-CoV-2 RBD-specific antibodies have been authorized for full or emergency use by the US Food and Drug Administration and by the European Commission following demonstration of clinical benefits for the treatment of COVID-19 [Citation5–9].
Emergence of new SARS-CoV-2 variants is of concern as mutations in the spike protein may impact the neutralizing activity of antibodies elicited by vaccination or the efficacy of therapeutic neutralizing antibodies [Citation10,Citation11]. Since 2020, SARS-CoV-2 variants of concern have been identified and monitored by the World Health Organization [Citation12]. The Omicron variant, first detected in South Africa in November 2021, rapidly became the dominant variant around the globe [Citation13–15]. Three major sub-lineages of Omicron have been identified: BA.1, BA.2, and BA.3 [Citation14–16]. Studies have shown that Omicron may be able to escape immunity stimulated by both natural infection and vaccines [Citation14,Citation17]. Computational and in vitro studies have also suggested that Omicron may evade approved therapeutic neutralizing antibodies [Citation18–21]. A recent pseudovirus study concluded that of 19 therapeutic mAbs tested, only one was able to neutralize all the Omicron sub-lineages, indicating that new broadly neutralizing antibodies are needed [Citation22].
We used a phage library, constructed from blood isolated from convalescent patients with COVID-19, to screen for mAbs targeting the RBD of the SARS-CoV-2 spike protein. We have previously reported a highly potent fully human neutralizing antibody, CT-P59 (regdanvimab) [Citation23]. Screening continued to identify antibodies retaining broad-spectrum in vitro neutralizing activity against emerging variants and CT-P63 was found to bind with high affinity to all major SARS-CoV-2 variants of concern. Here, we report in vitro and in vivo characterization studies plus a randomized, Phase I, single ascending dose study conducted to evaluate the safety, tolerability, and pharmacokinetics (PK) of CT-P63 in healthy participants.
Materials and methods
Viruses for in vitro experiments
Wild-type bearing D614G (hCoV-19/South Korea/KUMC17/2020), Gamma (hCoV-19/Korea/KDCA95637/2021), Delta (hCoV-19/Korea/KDCA5439/2021), Epsilon (hCoV-19/Korea/KDCA1792/2020 and hCoV-19/Korea/KDCA1793/2020), and Kappa (hCoV-19/Korea/KDCA2950/2021) variant viruses were obtained and cultured as previously described [Citation23,Citation24]. Additionally, Alpha (hCoV-19/South Korea/KDCA0838/2020), Beta (hCoV-19/South Korea/KDCA0463/2020), Zeta (hCoV-19/Korea/KDCA72731/2021), Iota (hCoV-19/Korea/KDCA82438/2021), Eta (hCoV-19/Korea/KDCA79765/2021), and Omicron subvariants BA.1 (hCoV-19/South Korea/KDCA18126/2021), BA.2 (hCoV-19/South Korea/KDCA61368/2022), BA.2.12.1 (hCoV-19/South Korea/KDCA58217/2022) were obtained from the National Culture Collection for Pathogens (Chungcheongbuk-do, Republic of Korea). All variant pseudoviruses expressing SARS-CoV-2 spike proteins were produced by K-Bio Health (Chungcheongbuk-do, Republic of Korea).
Plaque reduction neutralization test (PRNT)
PRNT was performed as previously described [Citation23]. Briefly, CT-P63 was pre-incubated with virus and the complexes added to VeroE6 cells (ATCC, CRL-1586). Following incubation, neutralization activity was determined by plaque counting. Data were fitted to a dose–response inhibition model and the concentration required to neutralize 50% of viral infectivity (half-maximal inhibitory concentration [IC50]) was determined by four-parameter curve fitting using GraphPad Prism6 (GraphPad Prism Software, Inc., San Diego, CA, USA).
Pseudovirus assay
The pseudovirus neutralization assay was conducted as previously described [Citation24]. In brief, luciferase-based pseudoviruses were mixed with serial dilutions of CT-P63 and added to ACE2-expressing HEK293T cells (ATCC, CRL-3216 transduced with Addgene ACE2 plasmid [145839]). IC50 values were calculated after 72 h, using GraphPad Prism9.
Biolayer interferometry (BLI)
ACE2 binding inhibition by CT-P63 and binding specificity of CT-P63 to RBDs of SARS-CoV-2, severe acute respiratory syndrome coronavirus (SARS-CoV), human coronavirus HKU1 (HCoV-HKU1), and Middle East Respiratory Syndrome coronavirus (MERS-CoV) were evaluated by BLI using the Octet QKe system (FortéBio, Fremont, CA, USA) as previously described [Citation23]. Binding affinity of CT-P63 to wild-type and mutant SARS-CoV-2 RBDs (Sino Biological, Beijing, China) was measured as follows. Each RBD (50 nM) was loaded onto Anti-Penta-HIS Biosensors (Sartorius, Göttingen, Germany) for 7.5 min, and CT-P63 at a concentration of 1.25, 2.5, 5, and 10 nM was flowed for 10 and 15 min to generate association and dissociation curves, respectively. Octet Data Acquisition v11.0 software, FortéBio Data Analysis version 11.0 and Data Analysis HT version 11.0 (FortéBio) were used to collect and analyse the data, respectively.
Surface plasmon resonance (SPR)
The SPR assay for assessment of binding affinity of CT-P63 to SARS-CoV-2 RBD was conducted as previously described [Citation23].
In vivo experiments
Eight-week-old female human angiotensin-converting enzyme 2 (hACE2) transgenic mice, tg(K18-ACE2)2Prlmn (The Jackson Laboratory, Bar Harbor, ME, USA), were housed in a certified animal biosafety level 3 (A/BSL-3) facility at International Vaccine Institute (IVI; Seoul, Republic of Korea). All procedures were approved by the Institutional Animal Care and Use Committee at IVI (IACUC Approval No. 2020-021).
The aim of the mouse studies was to determine the minimal in vivo effective dose of CT-P63. The doses evaluated were based on previous prophylactic and therapeutic studies with CT-P59 and the different SARS-CoV-2 variants [Citation24] and consideration of clinical doses of CT-P63. In the prophylaxis study, mice (n = 6/group) were administered CT-P63 (1 or 10 mg/kg) or vehicle control (placebo group) via a single intraperitoneal injection 24 h prior to intranasal inoculation (under anaesthesia) with 30 μL (1 × 105 plaque-forming units [PFU]) of wild-type SARS-CoV-2 (BetaCoV/Korea/KCD03/2020). Three mice from each group were scheduled for necropsy at Days 3 and 6 post-inoculation. For variant efficacy studies, mice (n = 10/group for Beta study; n = 11/group for Delta study; n = 8/group for Omicron study) were intranasally inoculated (under anaesthesia) with Beta (B.1.351; hCoV-19/Korea/KDCA55905/2021), Delta (B.1.617.2; hCoV-19/Korea/KDCA119861/2021), or Omicron (BA.1; hCoV19/Korea/KDCA447321/2021) variants (1 × 104 PFU for Beta and Delta and 1 × 105 PFU for Omicron), respectively. CT-P63 (20 or 40 mg/kg in the Beta study; 10 mg/kg in the Delta study; 5.33 mg/kg in the Omicron study) or formulation buffer (placebo groups) was administered via a single intraperitoneal injection at 8 h post-inoculation. Five mice from the Beta groups (CT-P63 and placebo) and four from the Delta groups were scheduled for necropsy at Days 3 and 6 post-inoculation. Four mice from the Omicron groups were scheduled for necropsy at Day 3 post-inoculation. Lung tissues and nasal washes were collected at necropsy and viral titres determined as previously described [Citation24]. Body weight and mortality were monitored daily until 6 days post-infection for the wild-type and Beta studies, and 10 days post-infection for the Delta and Omicron studies. Animals with 30% loss of body weight were euthanized according to the facility’s standard and excluded from statistics. Statistical significance was determined by one-way analysis of variance with Dunnett’s post hoc test.
CT-P63 1.1 study methods
Study design and procedures
A randomized, double-blind, placebo-controlled, parallel-group, single ascending dose, Phase I study in healthy adults (NCT05017168) was conducted at the Biokinetica S.A. clinical centre (Józefow, Poland). Participants were screened between Day −21 and Day −2 and admitted on Day −1 where they remained until completion of 48-h (Day 3) assessments. End-of-study (EOS) assessments were performed on Day 90.
Enrolled participants were assigned by permutated block randomization to three dose cohorts in cohort-dependent ratios to receive a single dose of CT-P63 or placebo on Day 1 (Figure S1). Procedure details and criteria for dosing discontinuations are outlined in the Supplementary Methods. A prespecified, study-independent, unblinded pharmacist who had no other participant contact dispensed drugs for administration. CT-P63 doses of 600, 900, and 1800 mg were administered as 960 mg/16 mL intravenous (IV) infusions over 60 ± 15 min; placebo IV infusions were matched to volume and packaging for each CT-P63 cohort. The study was unblinded to a predefined team at the sponsor for reporting key safety data up to Day 14 for each participant after database lock. Investigators, participants, predefined study centre, and Contract Research Organization staff remained blinded until all participants had completed the study and the database was finalized for study termination.
The study was conducted in compliance with the International Council for Harmonisation Good Clinical Practice and was approved by an independent ethics committee at the study centre prior to commencement. All participants provided written informed consent.
Participants
Full eligibility criteria are in the Supplementary Methods. Briefly, eligible participants were healthy adults aged 18–60 years (inclusive) with a body mass index (BMI) of 18.0–29.9 kg/m2 (inclusive). Negative SARS-CoV-2 reverse transcription polymerase chain reaction (RT–PCR) and antibody tests were required. Participants must not have received any investigational or approved SARS-CoV-2 vaccine.
Study endpoints
The primary objective was to evaluate safety and tolerability of CT-P63 up to Day 14 for last enrolled participant. Primary endpoints were incidence of treatment-emergent adverse events (TEAEs), treatment-emergent serious adverse events (TESAEs), and TEAEs of special interest (TEAESIs), comprising infusion-related reactions (IRRs) including hypersensitivity/anaphylactic reaction. Secondary endpoints were analyzed for the whole study period and were as for primary endpoints plus vital signs, hypersensitivity, 12-lead electrocardiogram (ECG), physical examination findings, and clinical laboratory tests and immunogenicity (incidence of anti-drug antibodies [ADAs] and neutralizing antibodies [NAbs]). PK endpoints were analyzed for the whole study period and comprised area under the concentration–time curve from time zero to infinity (AUC0–inf) and dose-normalized AUC0–inf (AUC0–inf/Dose), area under the concentration–time curve from time zero to the last quantifiable concentration (AUC0–last) and dose-normalized AUC0–last (AUC0–last/Dose), maximum serum concentration (Cmax) and dose-normalized Cmax (Cmax/Dose), time to Cmax (Tmax), terminal elimination half-life (t½), percentage of AUC0–inf obtained by extrapolation (%AUCext), terminal elimination rate constant (λz), total body clearance (CL), and volume of distribution at steady state (Vss).
Study assessments
The study assessment schedule is shown in Table S1. TEAEs were coded using the Medical Dictionary for Regulatory Activities version 24.1 and graded using the Common Terminology Criteria for Adverse Events version 5.0. ADA positivity was determined through screening and confirmatory assays; NAbs were assessed for confirmed ADA-positive samples only. PK parameters were analysed by noncompartmental methods based on actual sampling time points, using Phoenix WinNonlin version 8.3.4 (Certara, Inc., Princeton, NJ, USA). Dose proportionality for AUC0–inf, AUC0–last, and Cmax was assessed using the power model with log-transformed PK parameters as a dependent variable and log-transformed dose as an independent variable. 90% confidence intervals (CIs) and standard error were estimated using least-squares regression.
Statistical analysis
The sample size of eight participants per cohort (six for CT-P63 and two for placebo) was determined empirically based on sample sizes in other Phase I studies investigating drug safety and tolerability; the total sample size was not based on a formal statistical hypothesis. Analysis sets are described in the Supplementary Methods. Safety analyses were performed on the Safety Set and summarized by CT-P63 cohort and a placebo group pooled from the three cohorts. PK analyses were performed on the PK Set and were summarized by CT-P63 cohort. Statistical analyses used Statistical Analysis System (SAS) software version 9.4 (SAS Institute Inc., Cary, NC, USA).
Results
In vitro neutralization potency of CT-P63
CT-P63 inhibited SARS-CoV-2 viral replication with an IC50 of 50.5 ng/mL against wild-type SARS-CoV-2 bearing the D614G mutation (B.1 lineage) (). CT-P63 retained neutralizing activity against SARS-CoV-2 variants including Omicron variants (BA.1, B.2, BA.2.12.1 and BA.3; and Figure S2). Assays with pseudoviruses expressing mutant SARS-CoV-2 spike proteins confirmed the broad neutralization potency of CT-P63 (). SPR analysis demonstrated the high affinity of CT-P63 for SARS-CoV-2 RBD with a KD value of 0.17 nM (data not shown). CT-P63 completely inhibited binding between the SARS-CoV-2 RBD and ACE2 (Figure S3A). The specificity of CT-P63 binding to the SARS-CoV-2 RBD was confirmed by comparison with RBD proteins from SARS-CoV, HCoV-HKU1, and MERS-CoV (Figure S3B). Binding affinity of CT-P63 for wild-type RBD, as determined by BLI (0.129–0.208 nM; Table S2), demonstrated good correlation with that observed by SPR (0.17 nM). Further BLI binding assays demonstrated that CT-P63 retained affinity for all SARS-CoV-2 RBD mutants tested with KD values ranging from 0.086 nM (S494P mutant) to 0.915 nM (BA.2.12.1 Omicron mutant; Table S2).
Table 1. In vitro neutralization potency of CT-P63 against live viruses and pseudoviruses.
Prophylactic efficacy of CT-P63 against wild-type SARS-CoV-2 in vivo
The efficacy of CT-P63, administered prophylactically, against wild-type SARS-CoV-2 in an hACE2 transgenic mouse model was assessed. All animals in placebo and CT-P63 groups survived during the study. Placebo-treated animals experienced mean body weight loss up to 18.7% at 6 days post-infection; body weight loss was significantly attenuated in CT-P63-treated animals from 3 to 6 days post-infection (A). CT-P63 significantly reduced viral titres in the respiratory tract. Mean lung viral titre was 5.5 log10 PFU/mL at 3 days post-infection, declining to 4.5 log10 PFU/mL at 6 days post-infection in the placebo group (B). With CT-P63, viral titres were significantly reduced at 3 days post-infection in a dose-proportional manner. No virus was detectable in the CT-P63 groups at 6 days post-infection, other than in one animal in the CT-P63 10 mg/kg group. The mean viral titre in nasal washes was 2.0 log10 PFU/mL at 3 days post-infection and 3.2 log10 PFU/mL at 6 days post-infection in the control group (C). Viral titres were lower in the CT-P63 groups at 6 days post-infection.
Figure 1. Prophylactic efficacy of CT-P63 against wild-type SARS-CoV-2 in an hACE2 transgenic mouse model. (A) Mean (SD) change in body weight. (B) Lung viral titres. (C) Nasal wash viral titres.
Notes: In panel A, a denotes p < 0.05 between placebo and CT-P63 1 mg/kg group and b denotes p < 0.05 between placebo and CT-P63 1 mg/kg and 10 mg/kg groups. In panels B and C, *, **, ***, and **** denote p < 0.05, p < 0.01, p < 0.001, and p < 0.0001 relative to placebo, respectively. hACE2, human angiotensin-converting enzyme 2; SARS-CoV-2, severe acute respiratory syndrome coronavirus 2; SD, standard deviation.
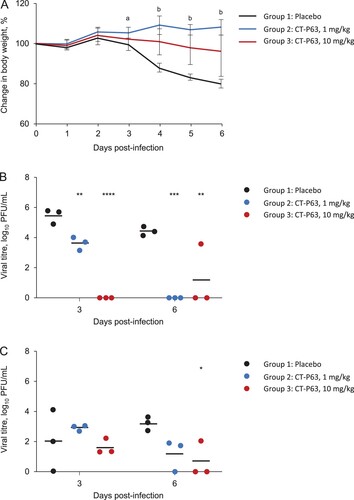
Efficacy of CT-P63 against SARS-CoV-2 variants in vivo
Beta variant
All untreated and CT-P63-treated animals (20 or 40 mg/kg) survived to 6 days post-infection. Mean body weight of the control group decreased up to 26.9%; body weight reductions were significantly reduced with CT-P63 (A). Mean lung viral titre was 5.30 and 2.19 log10 PFU/mL in the control group at 3 and 6 days post-infection, respectively; infectious virus was not detected in any CT-P63-treated animals (B). In control animals, the mean viral titre in nasal washes was 2.53 log10 PFU/mL at 3 days post-infection, declining to 0.77 log10 PFU/mL at 6 days post-infection (C). Viral titres were undetectable in the CT-P63 groups, other than in one animal in the 20 mg/kg group at 3 days post-infection.
Figure 2. Efficacy of CT-P63 against SARS-CoV-2 Beta, Delta, and Omicron variants in an hACE2 transgenic mouse model. (A) Mean (SD) change in body weight after Beta variant infection. (B) Lung viral titres after Beta variant infection. (C) Nasal wash viral titres after Beta variant infection. (D) Mean (SD) change in body weight after Delta variant infection. (E) Lung viral titres after Delta variant infection. (F) Nasal wash viral titres after Delta variant infection. (G) Mean (SD) change in body weight after Omicron variant infection. (H) Lung viral titres after Omicron variant infection at Day 3 post-infection. (I) Nasal wash viral titres after Omicron variant infection at Day 3 post-infection.
Notes: In panel A, a denotes p < 0.001 between placebo and CT-P63 20 mg/kg group and p < 0.0001 between placebo and CT-P63 40 mg/kg group and b denotes p < 0.001 between placebo and CT-P63 20 mg/kg and 40 mg/kg groups. In panels B, C, D, E, F, G, H, and I *, **, ***, and **** denote p < 0.05, p < 0.01, p < 0.001, and p < 0.0001 relative to placebo, respectively.hACE2, human angiotensin-converting enzyme 2; SARS-CoV-2, severe acute respiratory syndrome coronavirus 2; SD, standard deviation.
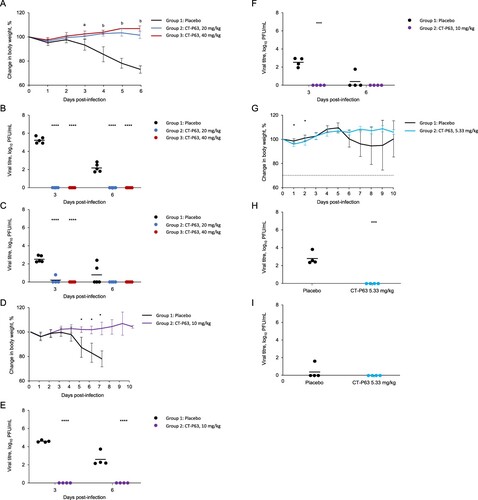
Delta variant
None of the untreated animals survived beyond 8 days post-infection; all CT-P63-treated animals (10 mg/kg) survived to 10 days post-infection. Mean body weight of the control group decreased up to 22.1%; reductions in body weight were reduced with CT-P63 (D). Mean lung viral titre was 4.62 and 2.61 log10 PFU/mL at 3 and 6 days post-infection, respectively, in the control group (E). No virus was detectable in the CT-P63 group. In nasal washes, the mean viral titre in the control group reached 2.57 log10 PFU/mL at 3 days post-infection, declining to 0.63 log10 PFU/mL at 6 days post-infection (F). No virus was detectable in the CT-P63 group.
Omicron variant
Three out of four untreated animals survived beyond 8 days post-infection and all CT-P63-treated animals (5.33 mg/kg) survived to 10 days post-infection. Mean body weight of the control group decreased up to 5.4%; reductions in body weight were reduced with CT-P63 (G). Mean lung viral titre was 2.88 log10 PFU/mL at 3 days post-infection in the control group (H). No virus was detected in the CT-P63 group. In nasal washes, a viral titre was detectable in only one animal in the control group and reached 1.61 log10 PFU/mL at 3 days post-infection, and no virus was detectable in the CT-P63 group (I).
CT-P63 1.1 study findings
Participant disposition and characteristics
The first participant was randomized on 11 October 2021, and the last participant completed the study on 25 January 2022. There were no major protocol deviations throughout the study. All 24 participants met the inclusion and exclusion criteria and were randomized to receive one of three doses of CT-P63, or placebo (intent-to-treat population) (). Twenty-three (95.8%) participants completed the study; one participant in the CT-P63 1800 mg group discontinued at EOS (lost to follow-up). Overall, demographic and baseline characteristics were comparable between groups ().
Figure 3. Participant flow diagram.
*Four back-up participants were included, in case enrolment was not met; these participants discontinued the study before randomization.
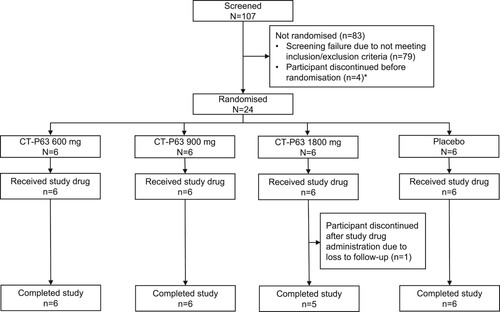
Table 2. Participant demographics and baseline characteristics (ITT Set).
Safety and immunogenicity
All participants received the planned dose of CT-P63. Overall, 15 TEAEs were reported for eight (33.3%) participants throughout the study period; most were of grade 1–2 in intensity (). The most frequently reported TEAE was COVID-19, experienced by four (66.7%) participants in the placebo group. The only other TEAE reported by at least two participants was headache, occurring in one (16.7%) participant in each of the CT-P63 1800 mg and placebo groups. There were no deaths, TESAEs, TEAESIs of IRR, or TEAEs leading to study drug discontinuation. Overall, mean clinical laboratory parameters were within normal ranges at each visit; there were no trends noted between CT-P63 and placebo groups. One clinical laboratory parameter, creatine phosphokinase, shifted from normal at baseline to an abnormal value considered clinically significant by the investigator; this occurred at Day 7 in one (16.7%) participant in the CT-P63 1800 mg group, had normalized at next visit (Day 14), and was considered unrelated to study drug. There were no clinically notable abnormalities reported for vital signs, hypersensitivity monitoring, 12-lead ECG, or physical examination. All seven female participants were potentially able to bear children, but no cases of pregnancy were reported during the study. No participants recorded positive ADA results after study drug administration in any treatment group up to EOS.
Table 3. Summary of TEAEs (Safety Set).
Pharmacokinetics
Serum CT-P63 concentrations rapidly peaked following the single IV infusion, before declining in a biphasic manner (). Mean serum concentrations were greater across time points for the higher CT-P63 dose groups. CT-P63 was detectable in serum up to 2136 h post-dose for all participants who received study drug. A summary of the PK parameters for CT-P63 are shown in . Median values of Tmax ranged from 1.56 to 2.08 h across the CT-P63 groups. Geometric mean values of t½ and CL were comparable across the treatment groups. Geometric mean values for dose-normalized AUC0–inf, AUC0–last, and Cmax were similar between the CT-P63 600 and 900 mg groups, and slightly lower in the 1800 mg group. Systemic exposure (AUC) increased dose proportionally over the studied range of doses, as 90% CI for the slope estimates included 1 (Figure S4A and S4B). Increases in maximal exposure (Cmax) were slightly less than dose proportional (Figure S4C).
Figure 4. Mean (SD) serum CT-P63 concentrations over time* (A) linear scale and (B) semi-logarithmic scale (PK Set).
*The lower limit of quantification was 1.00 µg/mL; concentrations below this were treated as 0. PK, pharmacokinetic; SD, standard deviation.
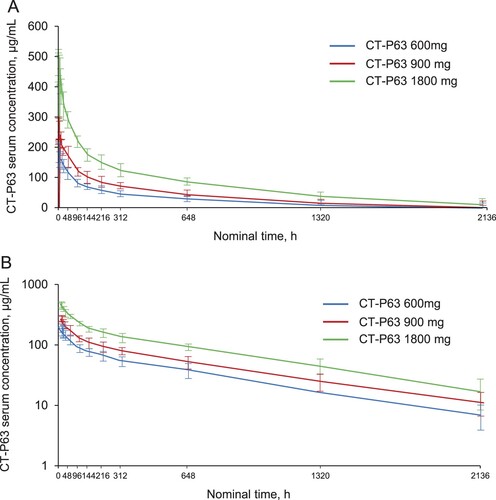
Table 4. Summary of serum PK parameters for CT-P63 (PK Set).
Discussion
The continued emergence of SARS-CoV-2 variants with the potential to escape existing immunity raises serious concerns and highlights the need for new therapies for COVID-19. We have conducted extensive in vitro and in vivo studies to characterize CT-P63, a novel recombinant human mAb. CT-P63 exhibited neutralizing activity against a broad spectrum of SARS-CoV-2 variants and pseudoviruses, including Omicron BA.1, BA.2, BA.2.12.1, and BA.3. In addition, the potential therapeutic efficacy of CT-P63 against wild-type SARS-CoV-2 and Beta and Delta variants was demonstrated in a mouse model. For the Omicron variant, in vitro neutralizing activity translated into in vivo efficacy as shown by the significantly reduced lung virus burden compared with control. The current mouse model demonstrated attenuated symptoms and viral burden in the respiratory tract following an Omicron variant infection compared to other variants as reported in recent studies [Citation25,Citation26].
The in vitro neutralization and BLI assays demonstrated specificity of CT-P63 for the SARS-CoV-2 RBD, and it retained broad coverage of SARS-CoV-2 RBD variants, including Beta, Delta, and sub-lineages of Omicron. Compared with wild-type, CT-P63 showed a <2-fold reduction in activity versus Omicron subvariants (BA.1, BA.2, and BA.2.12.1) in a live virus assay. In the pseudovirus assay, the IC50s for CT-P63 were in the range of 12–22.3 ng/mL versus Omicron subvariants (BA.1, BA.2, BA.2.12.1) compared with 4.8 ng/mL for wild-type. Many in vitro neutralization studies have been conducted to assess the activity of approved and advanced clinical development mAbs against these variants of concern [Citation10,Citation11,Citation21,Citation22,Citation24,Citation27–29]. Some mAbs retained activity versus Beta and Delta [Citation10,Citation11,Citation24,Citation29], but nearly all have shown a marked reduction in activity versus Omicron [Citation21,Citation22,Citation27,Citation28]. S309, the parent of sotrovimab, retained activity versus Omicron BA.1 with a ∼2-fold reduction in potency [Citation21,Citation30], but activity was lost against Omicron BA.2 (potency decreased by 27-fold) [Citation22]. Currently, only LY-CoV1404 (bebtelovimab) has demonstrated activity against all Omicron sub-lineages [Citation22]. Our results for CT-P63, and the additional in vivo findings that suggest a single dose of CT-P63 could effectively neutralize the Beta, Delta, and Omicron variants, indicate that CT-P63 potently neutralizes all currently known variants of SARS-CoV-2. Newly emerging Omicron variants BA.4 and BA.5 (L452R) in South Africa have the potential to spread worldwide [Citation12,Citation31]. Given our results from neutralization tests with variants that have L452R/Q, such as Delta, Lambda and Omicron BA.2.12.1, it is not unreasonable to expect that CT-P63 will be effective against these Omicron variants.
A Phase I study in healthy individuals demonstrated that a single IV infusion of CT-P63 at doses from 600 to 1800 mg was safe and well tolerated. There were no reported TEAEs related to study drug and no IRRs or other dose-dependent events in the CT-P63 treatment groups, confirming the safety profile observed previously [Citation32,Citation33].
PK analyses revealed that systemic exposure was dose-proportional and maximal exposure was slightly less than dose-proportional over the studied dose range (600 to 1800 mg). Geometric mean values (geometric CV%) of t½ were 578.4 h (21.0) for CT-P63 600 mg, 643.3 h (19.0) for CT-P63 900 mg, and 600.1 h (20.9) for CT-P63 1800 mg treatment groups, confirming a long t½. Of particular note, the geometric CV% value of Cmax for CT-P63 600 mg group was found to be 191.3 μg/mL (17.9), which greatly exceeds the IC50 of CT-P63 versus the Omicron live virus (96.48 ng/mL). The active moieties of CT-P63 will be largely out of the systemic circulation after five half-lives (∼126 days after administration). Given the clinical course of COVID-19, the PK profile of CT-P63 is suggestive of a single dose being sufficient in the treatment of the disease.
Our report does have some limitations. This was a small, single ascending dose study. As such, participant numbers for each CT-P63 group were small, so caution in interpreting the data is needed. The healthy individuals enrolled were aged between 21 and 44 years and may not be representative of the individuals expected to receive CT-P63 in the clinic who will likely be older with comorbidities. The safety, tolerability, and PK findings need to be evaluated in large-scale trials enrolling patients infected with SARS-CoV-2.
In conclusion, the neutralizing ability of CT-P63 versus common variants of SARS-CoV-2, including Omicron, was confirmed in vitro. Efficacy was demonstrated in vivo, and results suggest that a single dose of CT-P63 could effectively neutralize the Beta, Delta and Omicron variants. Safety and PK were confirmed in a healthy human Phase I study.
Supplemental Material
Download MS Word (829.8 KB)Acknowledgements
We thank all participants and investigators involved in the study. Medical writing support, including development of a draft outline and subsequent drafts in consultation with the authors, collating author comments, copyediting, fact checking, and referencing, was provided by Beatrice Tyrrell, DPhil, at Aspire Scientific Limited (Bollington, UK). Funding for medical writing support for this article was provided by Celltrion, Inc. (Incheon, Republic of Korea).
Disclosure statement
J.M.S., R.S., B.K., H.N., C.K., J.I.K., M.K., D.K.R., S.K., K.A., N.J., and S.Y.L. are employees of Celltrion, Inc. M.T-K., A.W., A.K are employees of Biokinetica which was sponsored by Celltrion, Inc., for conduct of this study. M.H.L., J.S.Y., K.C.K., J.Y.L., H.L., H.M.W., J.W.K., J.A.C., M.S. report no potential conflict of interest.
Data availability statement
The authors confirm that the data supporting the findings of this study are available within the article and its supplementary materials.
Additional information
Funding
References
- World Health Organization. WHO coronavirus (COVID-19) dashboard [Internet]. 2022 [cited 2022 May 17]. Available from: https://covid19.who.int/.
- Bruzzesi E, Ranzenigo M, Castagna A, et al. Neutralizing monoclonal antibodies for the treatment and prophylaxis of SARS-CoV-2 infection. New Microbiol. 2021;44(3):135–144.
- Shang J, Ye G, Shi K, et al. Structural basis of receptor recognition by SARS-CoV-2. Nature. 2020;581(7807):221–224.
- Hoffmann M, Kleine-Weber H, Schroeder S, et al. SARS-CoV-2 cell entry depends on ACE2 and TMPRSS2 and is blocked by a clinically proven protease inhibitor. Cell. 2020;181(2):271–280.e278.
- Mornese Pinna S, Lupia T, Scabini S, et al. Monoclonal antibodies for the treatment of COVID-19 patients: an umbrella to overcome the storm? Int Immunopharmacol. 2021;101(Pt A):108200–108200.
- Gottlieb RL, Nirula A, Chen P, et al. Effect of bamlanivimab as monotherapy or in combination with etesevimab on viral load in patients with mild to moderate COVID-19: a randomized clinical trial. JAMA. 2021;325(7):632–644.
- Gupta A, Gonzalez-Rojas Y, Juarez E, et al. Early treatment for COVID-19 with SARS-CoV-2 neutralizing antibody sotrovimab. N Engl J Med. 2021;385(21):1941–1950.
- Streinu-Cercel A, Săndulescu O, Preotescu L-L, et al. Efficacy and safety of regdanvimab (CT-P59): a Phase 2/3 randomized, double-blind, placebo-controlled trial in outpatients with mild-to-moderate coronavirus disease 2019. Open Forum Infect Dis. 2022;9(4):ofac053.
- Weinreich DM, Sivapalasingam S, Norton T, et al. REGN-COV2, a neutralizing antibody cocktail, in outpatients with COVID-19. N Engl J Med. 2021;384(3):238–251.
- Planas D, Veyer D, Baidaliuk A, et al. Reduced sensitivity of SARS-CoV-2 variant Delta to antibody neutralization. Nature. 2021;596(7871):276–280.
- Wang P, Nair MS, Liu L, et al. Antibody resistance of SARS-CoV-2 variants B.1.351 and B.1.1.7. Nature. 2021;593(7857):130–135.
- World Health Organization. Tracking SARS-CoV-2 variants [Internet]. 2022 [cited 2022 Apr 20]. Available from: https://www.who.int/en/activities/tracking-SARS-CoV-2-variants/.
- Callaway E. Heavily mutated Omicron variant puts scientists on alert. Nature. 2021;600(7887):21.
- Jung C, Kmiec D, Koepke L, et al. Omicron: what makes the latest SARS-CoV-2 variant of concern so concerning? J Virol. 2022;96(6):e0207721.
- Mahase E. COVID-19: what do we know about omicron sublineages? Br Med J. 2022;376:o358.
- Mahase E. Omicron sub-lineage BA.2 may have “substantial growth advantage,” UKHSA reports. Br Med J. 2022;376:o263.
- Yu J, Collier AY, Rowe M, et al. Neutralization of the SARS-CoV-2 Omicron BA.1 and BA.2 variants. N Engl J Med. 2022;386(16):1579–1580.
- Shah M, Woo HG. Omicron: a heavily mutated SARS-CoV-2 variant exhibits stronger binding to ACE2 and potently escapes approved COVID-19 therapeutic antibodies. Front Immunol. 2022;12:830527–830527.
- Takashita E, Kinoshita N, Yamayoshi S, et al. Efficacy of antibodies and antiviral drugs against COVID-19 omicron variant. N Engl J Med. 2022;386(10):995–998.
- Hu YF, Hu JC, Chu H, et al. In-silico analysis of monoclonal antibodies against SARS-CoV-2 Omicron. Viruses. 2022;14(2):390.
- VanBlargan LA, Errico JM, Halfmann PJ, et al. An infectious SARS-CoV-2 B.1.1.529 Omicron virus escapes neutralization by therapeutic monoclonal antibodies. Nat Med. 2022;28(3):490–495.
- Iketani S, Liu L, Guo Y, et al. Antibody evasion properties of SARS-CoV-2 Omicron sublineages. Nature. 2022;604(7906):553–556.
- Kim C, Ryu DK, Lee J, et al. A therapeutic neutralizing antibody targeting receptor binding domain of SARS-CoV-2 spike protein. Nat Commun. 2021;12(1):288.
- Ryu DK, Kang B, Noh H, et al. The in vitro and in vivo efficacy of CT-P59 against Gamma, Delta and its associated variants of SARS-CoV-2. Biochem Biophys Res Commun. 2021;578:91–96.
- Halfmann PJ, Iida S, Iwatsuki-Horimoto K, et al. SARS-CoV-2 Omicron virus causes attenuated disease in mice and hamsters. Nature. 2022;603(7902):687–692.
- Shuai H, Chan JF, Hu B, et al. Attenuated replication and pathogenicity of SARS-CoV-2 B.1.1.529 Omicron. Nature. 2022;603(7902):693–699.
- Cao Y, Wang J, Jian F, et al. Omicron escapes the majority of existing SARS-CoV-2 neutralizing antibodies. Nature. 2022;602(7898):657–663.
- Lusvarghi S, Pollett SD, Neerukonda SN, et al. SARS-CoV-2 BA.1 variant is neutralized by vaccine booster-elicited serum, but evades most convalescent serum and therapeutic antibodies. Sci Transl Med. 2022 [Apr 6];14(645):eabn8543.
- Ryu DK, Song R, Kim M, et al. Therapeutic effect of CT-P59 against SARS-CoV-2 South African variant. Biochem Biophys Res Commun. 2021;566:135–140.
- Sheward DJ, Kim C, Ehling RA, et al. Neutralisation sensitivity of the SARS-CoV-2 omicron (B.1.1.529) variant: a cross-sectional study. Lancet Infect Dis. 2022;22(6):813–820.
- Callaway E. Are COVID surges becoming more predictable? New Omicron variants offer a hint. Nature. 2022;605(7909):204–206.
- Goldin L, Elders T, Werhane L, et al. Reactions and COVID-19 disease progression following SARS-CoV-2 monoclonal antibody infusion. Int J Infect Dis. 2021;112:73–75.
- Taylor PC, Adams AC, Hufford MM, et al. Neutralizing monoclonal antibodies for treatment of COVID-19. Nat Rev Immunol. 2021;21(6):382–393.