ABSTRACT
Pigeon paramyxovirus 1 (PPMV-1) is an antigenic host variant of avian paramyxovirus 1. Sporadic outbreaks of PPMV-1 infection have occurred in pigeons in China; however, few cases of human PPMV-1 infection have been reported. The purpose of this article is to report a case of severe human PPMV-1 infection in an individual with probable post-COVID-19 syndrome (long COVID) who presented with rapidly progressing pulmonary infection. The patient was a 66-year-old man who was admitted to the intensive care unit 11 days after onset of pneumonia and recovered 64 days after onset. PPMV-1 was isolated from the patient’s sputum and in cloacal smear samples from domesticated pigeons belonging to the patient’s neighbour. Residual severe acute respiratory syndrome coronavirus 2 was detected in respiratory and anal swab samples from the patient. Sequencing analyses revealed that the PPMV-1 genome belonged to genotype VI.2.1.1.2.2 and had the 112RRQKRF117 motif in the cleavage site of the fusion protein, which is indicative of high virulence. This case of cross-species transmission of PPMV-1 from a pigeon to a human highlights the risk of severe PPMV-1 infection in immunocompromised patients, especially those with long COVID. Enhanced surveillance for increased risk of severe viral infection is warranted in this population.
Introduction
Newcastle disease (ND) is a common infectious condition found in a wide range of avian species and has caused significant economic losses in the poultry industry [Citation1–3]. The causative agent of ND is virulent Newcastle disease virus (NDV), also known as avian paramyxovirus 1 (APMV-1) [Citation4]. It belongs to the genus Avulavirus, subfamily Paramyxovirinae within the family Paramyxoviridae [Citation5]. NDV is an enveloped virus with a negative-sense, single-stranded, non-segmented RNA genome of 15.2 kb that encodes six structural proteins, including nucleocapsid protein, phosphoprotein, matrix protein, fusion protein, haemagglutinin-neuraminidase, and RNA-dependent RNA polymerase protein [Citation5–7]. Based on phylogenetic analysis of the F gene sequence, NDV is divided into class I and class II [Citation8–10].
Pigeon paramyxovirus type 1 (PPMV-1) is an antigenic host variant of NDV that causes infection in pigeons [Citation11]. Pigeons are the natural hosts of PPMV-1 [Citation12]. PPMV-1 was first identified in the Middle East, specifically in Iraq, in the late 1970s. It then spread rapidly across Europe, causing the third epizootic of the 1980s [Citation13, Citation14]. The first PPMV-1 outbreak in pigeons in China was confirmed in 1985, and since then PPMV-1 has been reported in pigeons in many provinces in China, with genotype VI being dominant [Citation15–17]. PPMV-1 infection in humans is usually mild and self-limiting. Therefore, it is rarely reported. However, several immunocompromised cases with pneumonia have died as a result of respiratory failure [Citation18–20].
In February 2023, a 66-year-old man was identified to have PPMV-1 infection by metagenomics next-generation sequencing (mNGS). Epidemiological investigation showed that he had been exposed to domesticated pigeons before he became ill. The patient had previously had coronavirus disease 2019 (COVID-19) and had since experienced symptoms of post-COVID-19 syndrome (commonly known as long COVID). PPMV-1 was identified and isolated in a sputum sample from the patient and from cloacal smear samples taken from the pigeons. Severe acute respiratory syndrome coronavirus 2 (SARS-CoV-2) was also detected in samples from the patient. In this report, we present the epidemiological features and viral characteristics of human PPMV-1 infection and offer insights into the significance of surveillance for increased risk of severe viral infection in the population with long COVID.
Methods
Collection of clinical and epidemiological data
Our patient was confirmed to have APMV-1 infection based on mNGS sequencing on February 17, 2023. The patient’s clinical information was collected from the hospital in Beijing, China, which the patient first visited on February 6, 2023. A standardized surveillance reporting form was used by field investigators and clinicians to gather epidemiological and clinical data. These data included demographics, housing environment, underlying medical comorbidities, exposure history, clinical symptoms, clinical laboratory test results, treatment, clinical complications, and outcomes.
Collection of clinical samples and detection
Clinical samples were collected from the patient’s bronchoalveolar lavage fluid, sputum, throat swab, anal swab, and blood and from the pigeons (cloacal swab and environmental smear specimen) and were processed for detection of pathogens. RNA was extracted from the samples using the QIAamp Viral RNA Mini Kit (Qiagen, Hilden, Germany) and tested for PPMV-1 virus and SARS-CoV-2 by real-time reverse transcription polymerase chain reaction (RT-PCR) using commercial kits from NBGen and DAAN Biological Technology Co., Ltd. (Beijing, China), respectively. The above examinations were performed in strict accordance with the manufacturers’ instructions.
Staining and detection of immune-related cells
Blood was drawn from the patient via sterile venipuncture into EDTA vacutainers (Becton Dickinson, BD, Franklin Lakes, NJ, USA). Fresh blood was incubated with a 6-colour T-cell, B-cell, and natural killer (NK) cell panel of monoclonal antibodies specific for CD3 (BV510), CD56 (APC), CD45 (APC-Cy7), CD4 (PE), CD19 (BV421), and CD8 (FITC) in Trucount tubes. After cell surface staining for 15 min at room temperature in the dark, erythrocytes were lysed by incubation with lysis buffer (BD) for 10 min. Flow cytometry was performed on a BD FACSymphony™A5 system (BD) and analysed using FlowJo 10 software. BD CS&T beads were used for the flow cytometer set-up and control samples. Standardized fluorescence compensation for the FACSymphony flow cytometer was established using BD FC beads for all fluorochromes in conjunction with FlowJo 10 software and CS&T beads.
Next-generation sequencing and phylogenetic analysis
PPMV-1 genes were amplified by RT-PCR and library preparation using a Nextera XT DNA library preparation kit (Illumina, San Diego, CA, USA) for sequencing on the Illumina MiniSeq platform. Raw FastQ data for PPMV-1 were assembled using a CLC Genomics Workbench, version 21.0, and the BLAST sequence was searched against the NCBI database. MEGA 6.0 was used for sequence alignment and construction of a phylogenetic tree based on the F gene with PPMV-1 reference sequences downloaded from the NCBI. In the same way, SARS-CoV-2 RNA was amplified by RT-PCR using upstream and downstream primer combinations covering the entire genome and NEBNext Q5 hot start HiFi PCR master mix (New England Biolabs, Hitchin, UK). After library preparation, the genome was sequenced on the Illumina MiniSeq platform. Raw FastQ data for SARS-CoV-2 were assembled using the CLC Genomics Workbench, version 21.0, and the censuses sequence was uploaded to https://pangolin.cog-uk.io/ for type identification.
Isolation of PPMV-1 and SARS-CoV-2
To isolate PPMV-1, Madin-Darby bovine kidney (MDBK) cells were inoculated with samples and the virus was detected by an immunofluorescence assay. Confluent MDBK cells were inoculated with approximately 200 μL of the patient’s sputum sample or 200 μL of a pigeon cloaca sample and grown in 6-well plates containing 800 μL of maintenance medium consisting of Dulbecco’s modified Eagle’s medium (Invitrogen, Carlsbad, CA, USA) and antibiotics (100 U/mL penicillin and 100 g/mL streptomycin, Invitrogen). After viral absorption for 2 h, the infection medium was replaced with maintenance medium and incubated at 37°C. Cytopathic effects (CPEs) were monitored after 1–2 days. The MDBK cells were fixed with 4% paraformaldehyde for 10 min, permeabilized with Triton X-100 for another 20 min, and incubated in blocking buffer for 10 min. The cells were then incubated with a mouse antibody against PPMV-1 nucleocapsid protein (1:1000, generated and kindly provided by Professor Honglei Sun, China Agricultural University) and then with an FITC-labelled secondary antibody (1:400, KPL Inc., Gaithersburg, MD, USA), both at 37°C for 60 min. The cells were then stained with 1 mg/mL DAPI. All images were acquired with a fluorescence microscope (Olympus, Tokyo, Japan).
For isolation of virus, Vero cells in six-well plates containing Dulbecco’s modified Eagle’s medium supplemented with 2% foetal bovine serum (Invitrogen) were inoculated with 1 mL of viral transport medium from the patient’s throat swab that was identified to be positive for SARS-CoV-2 and incubated in a CO2 incubator at 37°C. The plates were observed daily for CPEs using an inverted microscope (Eclipse Ti; Nikon, Tokyo, Japan). After cultivation for 5 days, serial passages were carried out. After three passages, the virus was identified by real-time RT-PCR.
Neutralizing antibody assay
Microneutralization assays were performed. Starting at a 1:10 dilution, 50 μL of two-fold serial dilutions of antisera were pre-incubated 1:1 with 100 median tissue culture infective doses (TCID50) of the virus stocks at 37°C for 2 h. The mixture was then transferred to 96-well plates containing MDBK cells and incubated for a further 18–20 h at 37°C in a 5% CO2 incubator. The end-point dilutions were assessed using an immunofluorescence assay.
Scanning and transmission electron microscopy
Scanning electron microscopy (SEM) was used to detect changes in the morphology of normal human bronchial epithelial (NHBE) cells following PPMV-1 inoculation to gain an understanding of the invasiveness of PPMV-1 in human respiratory epithelial cells. Virus isolated from the patient sputum specimen produced CPEs in monolayers of NHBE cells 48 h after inoculation. Briefly, NHBE cell cultures were inoculated with the virus at a multiplicity of infection (MOI) of 1. Forty-eight hours post-infection, infected and mock-infected NHBE cells were fixed with 2.5% glutaraldehyde (Soliabio, Beijing, China) at 4°C overnight, washed and dehydrated in an ethanol gradient, subjected to critical point drying, and vacuum-evaporated with platinum. The membrane supporting the NHBE cells was then cut from the Transwell rack and glued to a sample holder, and the samples were examined by SEM using a Hitachi SU8000 (Tokyo, Japan).
NHBE cells were infected with PPMV-1 at an MOI of 1, and cell culture supernatants were collected at 48, 72, and 96 h post-inoculation for virus titration. The titres of PPMV-1 in the cell supernatants at different time points were determined by TCID50 assays as described elsewhere [Citation21]. MDBK cells were seeded in 96-well plates at a density of 2 × 104 cells/well. After 24 h, the culture supernatants were serially diluted with Dulbecco’s modified Eagle’s medium, and MDBK cells were infected with 100 μL of 10-fold serial dilutions of harvested culture supernatants. The cells were incubated at 37°C for 96 h. The CPEs on cells in each well were observed using a light microscope (IX70; Olympus). TCID50 values were calculated using the method devised by Reed and Muench [Citation22].
Transmission electron microscopy (TEM) was used to observe the morphology of the virus particles with the aim of identifying the virus. Twenty hours after infection, MDBK cells infected with PPMV-1 at an MOI of 10 were fixed with 2.5% glutaraldehyde (Soliabio). After washing with phosphate-buffered saline, the samples were dehydrated with an alcohol gradient and embedded in Epon812 for 72 h at 60°C. Ultra-thin sections were cut, stained with uranium acetate and lead citrate, and then observed using a Hitachi HT7800 transmission electron microscope.
Results
Clinical information and pathogen detection
The patient first visited our hospital with a fever (38.5°C) on February 6, 2023 (day 1 of onset). Before this illness, the patient had experienced persistent physical weakness since being infected with SARS-CoV-2 on December 30, 2022. The patient also had a history of hypertension, coronary heart disease, and dyslipidaemia, for which he was prescribed aspirin and a statin. He complained of shivering, cough, palpitations, weakness, nausea, and continuous fever and had taken antipyretic agents at home. The patient was treated with moxifloxacin as an outpatient on February 6–9, 2023. On February 10 (day 5), his temperature increased to 39.7°C and was accompanied by cough, abdominal pain, and diarrhoea. Therefore, he was hospitalized for treatment. On February 16, 2023 (day 11), there had been no improvement and the patient was transferred to the intensive care unit. A computed tomography scan of the chest indicated bronchitis with thickening of the walls of the bronchial tubes in both lungs.
After the patient was admitted to hospital, relevant pathogens were sought. On February 17 (day 12), APMV-1 was suggested in bronchoalveolar lavage fluid by mNGS. Subsequently, sputum, throat swabs, and anal swabs were collected from the patient for detection of APMV-1, SARS-CoV-2, and other pathogens. The specific detection results are shown in .
Epidemiological investigations
Epidemiological investigation showed that the patient lived with his wife in a single-family cottage in an alley in an urban district of Beijing. The property’s floor area was approximately 70 square metres and the building had poor ventilation. A neighbour opposite the residence had raised pigeons in their attic for a long time. The residence of the patient was close to that of this neighbour. When the patient left his home, he needed to pass the house where the pigeons lived. The patient had intensive contact with the pigeons kept by the neighbour before the onset of his illness. Pigeon cloacal swabs and environmental smear samples of the loft tested positive for PPMV-1. The sequences of PPMV-1 samples from the patient and pigeons were identical. Based on epidemiological and aetiological evidence, cross-species transmission from pigeons to a human was confirmed.
Serum samples from close contacts (the patient’s wife and neighbour) were tested for neutralizing antibodies using MDBK cells. The patient’s microneutralization antibody titres were 1:8 on day 18 and day 23 and 1:16 on day 28 and day 31 after onset of the illness, while the close contacts were negative for the serum antibody.
Immune-related cell count analysis
To better understand the immune status of the patient, 6-colour flow cytometric assays using the BD FACSymphony™A5 system were performed to determine immune-related cell counts. White blood cell, lymphocyte, monocyte, total T lymphocyte, total B lymphocyte, NK cell, helper/inducer T-cell, and suppressive/cytotoxic T-cell counts () were significantly decreased in comparison with normal levels (, ).
Figure 2. Flow cytometry gating strategy of T-cells, B-cells and natural killer (NK) cells. A representative flow cytometry plot from an infected individual showing: (A) gated lymphocytes, monocytes-granulocytes. (B) NK cells are divided into functionally distinct CD56+/CD3- and CD56+/CD3 + subsets. (C) CD19+ B-cells, and CD3 + lymphocytes. (D) T-cells (CD3+) are divided based on CD4 + and CD8 + expression.
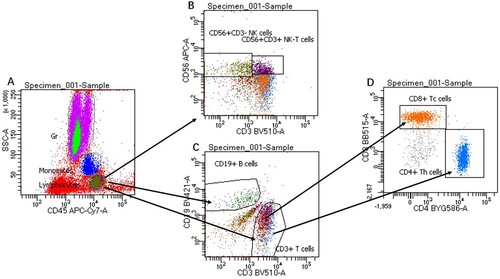
Table 1. Flow cytometry analysis of the patient’s peripheral blood.
Virus isolation and immunofluorescence assays
Virus was isolated from a sputum sample collected from the patient on February 18 (day 13 after illness onset) and from pigeon cloacal samples and was identified by immunofluorescence after inoculation with MDBK cells (). Virus isolated from the sputum specimen produced CPEs on monolayers of MDBK cells after 72 h. After 4 days, CPEs and detached cells were evident over the entire monolayer area. Multiple subsequent passages and isolation of plaques yielded culture suspension and infected cells from a single plaque of the virus. The culture suspension and cells were used for identification of the virus by fluorescence electron microscopy. After confirming the virus as PPMV-1 by real-time RT-PCR and immunofluorescence assays, the virus was named PPMV-1-202302. Vero cells were inoculated with clinical specimens for isolation of SARS-CoV-2, produced no typical CPEs, and were confirmed to be negative for SARS-CoV-2 by real-time RT-PCR.
Figure 3. Immunofluorescence identification of virus in MDBK cells. (A) Immunofluorescence assay of virus isolated from the patient’s sputum sample. (B) Immunofluorescence assay of virus isolated from pigeon cloacal smear samples. (C) Normal MDBK cells served as a mock control. MDBK, Madin-Darby bovine kidney.
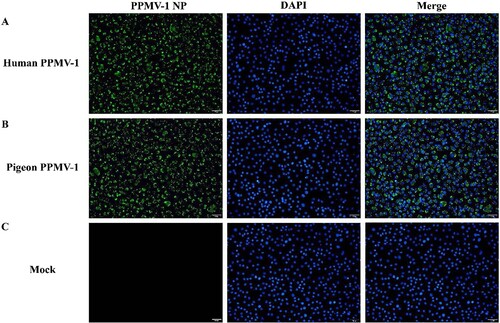
PPMV-1 and SARS-CoV-2 genome analysis
The sequences of the viruses isolated from the patient and the pigeons shared a nucleotide similarity of 100%. The full-length genome of the isolated virus displayed 99.45% nucleotide sequence identity with a PPMV-1 isolated from pigeons in eastern China (pigeon/Shandong/190610-2/2019, GenBank). Phylogenetic analysis clustered the virus within a group of pigeon viruses isolated in north China, including a strain isolated from the patient’s neighbour’s pigeons (). This indicates the possibility of transmission from pigeons to humans. The virus had the VI.2.1.1.2.2 genotype, which in China exists mainly in pigeons. Moreover, the 112RRQKRF117 motif was observed in the cleavage site of the fusion protein, which indicates high virulence in poultry. Furthermore, the SARS-CoV-2 genome was obtained from the sputum sample collected on February 19, 2023 with 96.1% coverage, and was subtyped as Omicron variant BF.7.14 through the Pangolin COVID-19 Lineage Assigner Web application (https://pangolin.cog-uk.io/).
Virus identification and infectivity analysis
The virus isolation culture supernatants were collected for virus purification using discontinuous sucrose gradient centrifugation, and the purified virus was examined by TEM. The virus particles were polymorphic with a diameter of 100–400 nm, and the spines on the surface were approximately 12–15 nm long (A).
Figure 5. Visualization of PPMV-1 isolated from the patient by transmission and scanning electron microscopy. (A) Virus particles were identified by transmission electron microscopy. Morphology of PPMV-1 viruses budding from infected MDBK cells. MDBK cells were infected with indicated viruses at an MOI of 10 and processed for transmission electron microscopy at 20 h after infection. (B) Cytopathic effects of PPMV-1 virus infection of NHBE cells. NHBE cells were infected with the indicated virus at an MOI of 0.1. Scanning electron microscopy was used to detect changes in the morphology of NHBE cells. Normal NHBE cells served as a mock control. Black arrows indicate PPMV-1 virus particles. MDBK, Madin-Darby bovine kidney; MOI, multiplicity of infection; NHBE, normal human bronchial epithelial.
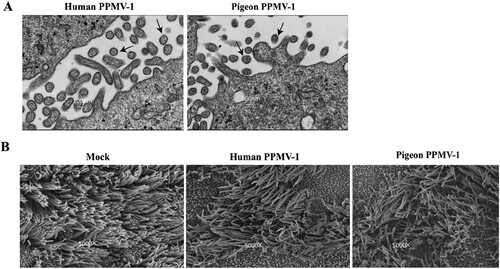
The replication ability of the virus from the patient and pigeons was examined in NHBE cells, which form a ciliated-pseudostratified columnar epithelium at an air-liquid interface. SEM was used to investigate the CPEs of PPMV-1-infected NHBE cells in detail (B). Shrinkage of cilia in the plaque region and beaded changes in the periphery of plaques were detected (B). The cilia were disordered in virus-infected cells in the far periphery of the plaques. Extracellular viral particles were observed in infected NHBE cells by TEM. The virus titres (TCID50/ml) at 48, 72, and 96 h post-inoculation were 6.3 × 102, 4.7 × 103, and 1 × 105, respectively. These findings indicate that epithelial cells in the human upper and lower respiratory tract are susceptible to PPMV-1 infection.
Discussion
PPMV-1 has long been prevalent among pigeons and leads to significant economic losses in the Chinese pigeon industry nationwide [Citation23–25]. Human infection with PPMV-1 is rarely reported. This report describes a case of PPMV-1 infection and isolation of the virus in a sputum sample from a human patient and a pigeon cloaca sample. The patient was also SARS-CoV-2-positive, having been diagnosed with COVID-19 on December 30, 2022. Whole genome sequences of PPMV-1 from the case and the pigeons were obtained by next-generation sequencing. With the timely detection of pathogens that in part directed clinical treatment, the patient had recovered by April 8, 2023.
In this study, mNGS was used to identify the causative pathogen in the patient’s samples. The interval between onset of the patient’s illness and identification of the pathogen was 12 days. Compared with a previous report in which NDV was identified by mNGS, which took 23 days [Citation18], this short detection time provided a valuable time window for effective clinical treatment. Ultimately, because of the early diagnosis by mNGS, the patient was successfully discharged from hospital 64 days after PPMV-1 infection. This report highlights the clinical benefit of early diagnosis by mNGS for patients with infections of unknown origin. The patient’s serum was positive for neutralizing antibody against PPMV-1, with a slight upward trend between days 18 and 31, indicating a recent infection. However, pathogen and serum antibody tests were negative for the patient’s wife and neighbour, indicating that the virus had limited transmission ability and that this was a case of cross-species infection rather than human-to-human transmission.
PPMV-1 is a common zoonotic pathogen that circulates in the pigeon reservoir and can occasionally spread to humans. Although the risk of infectious diseases originating from the human-animal interface is usually focused on the wild environment, close attention to interaction between domestic pets and humans in urbanized regions is also warranted. The comprehensive One Health strategy targeting both the wild environment and the human built environment is intended to prevent and control zoonotic pathogens [Citation26–27]. Action can be taken to control the risk of spillover or transmission to humans by reduced contact with animals. Meanwhile, intensive surveillance to track the activity of pathogenic microorganisms is supposed to be in place in both wild and domesticated species. Furthermore, universal or targeted vaccination is an optional strategy that can be used to contain infection and transmission in animals as well as between animals and humans.
On May 5, 2023, the World Health Organization stated that COVID-19 is now an established and ongoing health issue but no longer constitutes a public health emergency of international concern [Citation28]. However, it should be noted that the impact of long COVID is open-ended and involves extensive and diverse populations. During the period when COVID-19 was prevalent, the risk of severe infection caused by usually mild pathogens may have increased in individuals who had contracted COVID-19 to give rise to a population with underlying medical comorbidities, particularly in immunocompromised individuals. In late 2022, the BF.7.14 lineage was dominant in Beijing, and our patient was initially infected with SARS-CoV-2 during the same period. On February 18, 2023, residual SARS-CoV-2 was detected in respiratory samples from the patient and were also identified as BF.7.14 lineage. It seemed unlikely that the same variant was contracted in a such short period of time. Furthermore, on February 13, 2023 (week 7), the positive SARS-CoV-2 rate in virological surveillance was only 2.8% in Beijing, indicating that the virus activity was at a low level and the risk of infection with COVID-19 was minimal. The above-mentioned evidence indicates that the patient had since experienced symptoms of long COVID rather than reinfection. The case reported here, which met the above criteria, reflects this situation. Strengthening surveillance among at-risk groups with post-COVID-19 syndrome is important, especially for individuals having frequent contact with animals, including pets.
The patient described in this report had chronic underlying diseases with hypertension and coronary heart disease. He had experienced persistent physical weakness since being diagnosed with COVID-19 on December 30, 2022. The synergistic effect of the chronic underlying diseases and a previous infection with SARS-CoV-2 may have led to compromised immunity, which might have increased the severity of the PPMV-1 infection. It has not been reported and might be controversial whether a previous infection with SARS-CoV-2 increases the severity of the disease caused by PPMV-1 infection. However, it was previously reported that patients who were infected with COVID-19 and influenza had more severe symptoms and a higher risk of death than those who were infected with COVID-19 alone [Citation29]. The patient has lived in the same region for many years but has never been infected with PPMV-1. Nevertheless, after contracting COVID-19 in late 2022, he did not recover fully and was diagnosed with long COVID. In this physiological context, he was subsequently infected with PPMV-1 in February 2023. This infection pattern indicates that there may be a potentially causal relationship between PPMV-1 and COVID-19 rather than occasional co-infection, but the mechanism affecting the change of susceptibility is still unknown.
This study has several limitations. The mechanism of pigeon-to-human PPMV-1 cross-species transmission warrants further study. It is crucial to study viral and host factors associated with cross-species transmission to help develop effective intervention measures. Moreover, PPMV-1 has not been rigorously investigated, and its epidemiology and variation in pigeons require further investigation.
In conclusion, cross-species transmission of PPMV-1 from pigeons to humans is a new public health concern. mNGS can play a significant role in discovery and early diagnosis of novel pathogens. Enhanced surveillance to detect an increased risk of severe disease after viral infection is warranted in the population with long COVID.
Acknowledgement
Health care workers who contributed to the epidemiological survey, sample collection, and transportation. We were grateful to Dr Xiaohui Zou, from China–Japan Friendship Hospital, for kindly providing support to our manuscript.
Disclosure statement
No potential conflict of interest was reported by the author(s).
Additional information
Funding
References
- Alexander DJ. Ecology and epidemiology of Newcastle disease. In: Capua I, Alexander DJ, editor. Avian influenza and Newcastle disease: A field and laboratory manual. Milano: Springer Milan; 2009. p. 19–26.
- Ganar K, Das M, Sinha S, et al. APMV-1: current status and our understanding. Virus Res. 2014;184:71–81. doi:10.1016/j.virusres.2014.02.016
- Dimitrov KM, Ramey AM, Qiu X, et al. Temporal, geographic, and host distribution of avian paramyxovirus 1 (Newcastle disease virus). Infect Genet Evol. 2016;39:3922–3934. doi:10.1016/j.meegid.2016.01.008
- Kuhn JH, Adkins S, Alioto D, et al. Taxonomic update for phylum Negarnaviricota (Riboviria: Orthornavirae), including the large orders Bunyavirales and Mononegavirales. Arch Virol. 2020;165:3023–3072. doi:10.1007/s00705-020-04731-2
- Czegledi A, Ujvari D, Somogyi E, et al. Third genome size category of avian paramyxovirus serotype 1 (Newcastle disease virus) and evolutionary implications. Virus Res. 2006;120:36–48. doi:10.1016/j.virusres.2005.11.009
- Steward M, Vipond IB, Millar NS, et al. RNA editing in Newcastle disease virus. J Gen Virol. 1993;74:2539–2547. doi:10.1099/0022-1317-74-12-2539
- Karsunke J, Heiden S, Murr M, et al. W protein expression by Newcastle disease virus. Virus Res. 2019;263:207–216. doi:10.1016/j.virusres.2019.02.003
- Diel DG, da Silva LH, Liu H, et al. Genetic diversity of avian paramyxovirus type 1: proposal for a unified nomenclature and classification system of Newcastle disease virus genotypes. Infect Genet Evol. 2012;12:1770–1779. doi:10.1016/j.meegid.2012.07.012
- Lu X, Wang X, Zhan T, et al. Surveillance of class I Newcastle disease virus at live bird markets and commercial poultry farms in eastern China reveals the epidemic characteristics. Virol Sin. 2021;36:818–22. doi:10.1007/s12250-021-00357-z
- Dimitrov KM, Abolnik C, Afonso CL, et al. Updated unified phylogenetic classification system and revised nomenclature for Newcastle disease virus. Infect Genet Evol. 2019;74:103917, doi:10.1016/j.meegid.2019.103917
- Chong YL, Lam TT-Y, Kim O, et al. Successful establishment and global dispersal of genotype VI avian paramyxovirus serotype 1 after cross species transmission. Infect Genet Evol. 2013;17:260–268. doi:10.1016/j.meegid.2013.04.025
- Pestka D, Stenzel T, Koncicki A. Occurrence, characteristics and control of pigeon paramyxovirus type 1 in pigeons. Polish J. Vet. Sci. 2014;17:379–384. doi:10.2478/pjvs-2014-0056
- Aldous EW, Fuller CM, Mynn JK, et al. A molecular epidemiological investigation of isolates of the variant avian paramyxovirus type 1 virus (PPMV-1) responsible for the 1978 to present panzootic in pigeons. Avian Pathol. 2004;33:258–269. doi:10.1080/0307945042000195768
- Kaleta EF, Alexander DJ, Russell PH. The first isolation of the avian pmv-1 virus responsible for the current panzootic in pigeons ? Avian Pathol. 1985;14:553–557. doi:10.1080/03079458508436258
- Wang J, Liu H, Liu W, et al. Genomic characterizations of six pigeon paramyxovirus type 1 viruses isolated from live bird markets in China during 2011 to 2013. PLoS One. 2015;10:e0124261.
- Liu H, Wang Z, Son C, et al. Characterization of pigeon-origin Newcastle disease virus isolated in china. Avian Dis. 2006;50:636–640. doi:10.1637/7618-042606R1.1
- Guo H, Liu X, Han Z, et al. Phylogenetic analysis and comparison of eight strains of pigeon paramyxovirus type 1 (PPMV-1) isolated in China between 2010 and 2012. Arch Virol. 2013;158:1121–1131. doi:10.1007/s00705-012-1572-8
- Zou XH, Suo LJ, Wang YM, et al. Concurrent pigeon paramyxovirus-1 and Acinetobacter baumannii infection in a fatal case of pneumonia. Emerg Microbes Infect. 2022;11:968–977. doi:10.1080/22221751.2022.2054366
- Kuiken T, Breitbart M, Beer M, et al. Zoonotic infection with pigeon paramyxovirus type 1 linked to fatal pneumonia. J Infect Dis. 2018;218:1037–1044. doi:10.1093/infdis/jiy036
- Goebel SJ, Taylor J, Barr BC, et al. Isolation of avian paramyxovirus 1 from a patient with a lethal case of pneumonia. J Virol. 2007;81:12709–12714. doi:10.1128/JVI.01406-07
- Jang J, Hong SH, Kim IH. Validation of a real-time RT-PCR method to quantify Newcastle Disease Virus (NDV) titer and comparison with other quantifiable methods. J Microbiol Biotechnol. 2011;21:100–108. doi:10.4014/jmb.1006.06006
- Reed LJ, Skiadopoulos MH. A simple method of estimating fifty percent endpoints. Am J Hyg. 1938;27:493–497.
- Xie P, Chen L, Zhang Y, et al. Evolutionary dynamics and age-dependent pathogenesis of sub-genotype VI.2.1.1.2.2. Viruses. 2020;12:43.
- Tian Y, Xue R, Yang W, et al. Characterization of ten paramyxovirus type 1 viruses isolated from pigeons in China during 1996-2019. Vet Microbiol. 2020;244:108661, doi:10.1016/j.vetmic.2020.108661
- Mokili JL, Rohwer F, Dutilh BE. Metagenomics and future perspectives in virus discovery. Curr Opin Virol. 2020;2:63–77. doi:10.1016/j.coviro.2011.12.004
- Osterhaus ADME, Vanlangendonck C, Barbeschi M, et al. Make science evolve into a One Health approach to improve health and security: a white paper. One Health Outlook. 2020;2:6, doi:10.1186/s42522-019-0009-7
- Aarestrup FM, Bonten M, Koopmans M. Pandemics– one health preparedness for the next. Lancet Reg Health Eur. 2021;9:100210, doi:10.1016/j.lanepe.2021.100210
- WHO. Statement on the fifteenth meeting of the IHR (2005) Emergency Committee on the COVID-19 pandemic. https://www.who.int/news/item/05-05-2023-statement-on-the-fifteenth-meeting-of-the-international-health-regulations-(2005)-emergency-committee-regarding-the-coronavirus-disease-(covid-19)-pandemic.
- Swets MC, Russell CD, Harrison EM, et al. SARS-CoV-2 Co-infection with influenza viruses, respiratory syncytial virus, or adenoviruses. Lancet. 2022;399:1463–1464. doi:10.1016/S0140-6736(22)00383-X