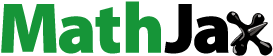
ABSTRACT
Limited follow-up data is available on the recovery of Omicron COVID-19 patients after acute illness. It is also critical to understand persistence of neutralizing antibody (NAb) and of T-cell mediated immunity and the role of hybrid immunity in preventing SARS-CoV-2 reinfection. This prospective cohort study included Omicron COVID-19 individuals from April to June 2022 in Shanghai, China, during a large epidemic caused by the Omicron BA.2 variant. A total of 8945 patients from three medical centres were included in the follow up programme from November 2022 to February 2023. Of 6412 individuals enrolled for the long COVID analysis, 605 (9.4%) individuals experienced at least one sequelae, mainly had fatigue and mental symptoms specific to Omicron BA.2 infection compared with other common respiratory tract infections. During the second-visit, 548 (12.1%) cases of Omicron reinfection were identified. Hybrid immunity with full and booster vaccination had reduced risk of SARS-CoV-2 reinfection by 0.29-fold (95% CI: 0.63–0.81) and 0.23-fold (95% CI: 0.68–0.87), respectively. For 469 participants willing to the hospital during the first visit, those who received full (72 [IQR, 36–156]) or booster (64 [IQR, 28–132]) vaccination had significantly higher neutralizing antibody titers than those with incomplete vaccination (36 [IQR, 16–79]). Moreover, non-reinfection cases had higher neutralizing antibody titers (64 [IQR, 28–152]) compared to reinfection cases (32 [IQR, 20–69]).
Introduction
With the continuous global spread of the COVID-19 pandemic, an increasing number of patients infected with the severe acute respiratory syndrome coronavirus 2 (SARS-CoV-2) have reported persistent and prolonged symptoms beyond the typical duration of SARS-CoV-2 infection [Citation1]. In October 2021, the World Health Organization (WHO) introduced a clinical definition for the post-COVID-19 condition, commonly known as long COVID, which includes symptoms that persist for at least 2 months and cannot be attributed to an alternative diagnosis within 3 months after SARS-CoV-2 infection [Citation2].
Long COVID has been linked to a range of symptoms that persist for several months after the acute phase [Citation2,Citation3]. Commonly reported symptoms include fatigue, brain fog, and shortness of breath, et al. [Citation2,Citation4–6], mainly for the SARS-CoV-2 original and Delta variant. The Omicron variant appears to cause less severe acute illness than previous variants, particularly in vaccinated populations [Citation7]. However, the potential for a significant number of individuals to develop persistent symptoms of the Omicron variant in the long term remains a major concern. It is crucial for public health to gain a comprehensive understanding of the long-term health effects and potential risk factors of Omicron infection. Additionally, it has been observed that other respiratory viruses, such as influenza, can also have long-term consequences [Citation8,Citation9], it needs to identify the specific long-term health effects of COVID-19 compared with other pathogens of respiratory tract infection.
Currently, there are increasing cases of SARS-CoV-2 repeat infections (reinfection) around the world, especially with the emergence of the Omicron variant, which has demonstrated the ability to evade immunity from previous infections [Citation1, Citation2]. There is also a need to gain a better understanding of the direct effect of hybrid immunity, i.e. immunity achieved by prior SARS-CoV-2 infection plus vaccination or vice versa in preventing reinfection [Citation10]. Addressing these issues will inform the implementation of strategies to prevent or reduce the risk of reinfection. In specific, former findings demonstrated that the duration of antibody response raised by COVID-19 vaccines could be prolonged for the uninfected population [Citation11–13]. However, the long-term duration of NAb and T-cell mediated immunity induced by hybrid immunity is largely unclear, as well as their effectiveness in protecting against reinfection.
China provided the most pristine environment to study the health and immunity outcomes, and the characteristics of the Omicron variant reinfection due to the zero-COVID policy that was abandoned in December 2022. Therefore, the primary objective of this study is to systematically characterize the health outcomes of Omicron COVID-19 survivors up to half a year after acute infection, and to determine the health impact and potential risk factors of long COVID. The secondary objective of this study is to assess the NAb titres and T-cell responses following primary Omicron infection with different doses of inactivated vaccination. Additionally, we further investigate the incidence, clinical symptoms of Omicron reinfection and its association with hybrid immunity.
Materials and methods
Study design and population
The study population included Shanghai residents with laboratory-confirmed SARS-CoV-2 infection by real-time polymerase reaction chain (RT–PCR) tests between April 2022 and June 2022. During hospitalization, inpatients received daily pharyngeal swab SARS-CoV-2 PCR tests, and the viral RNA shedding was confirmed when two consecutive negative tests with an interval of over 24 h were reported, with a cycle threshold value of at least 35 in both ORF1ab and N genes. Inclusion criteria were patients who had confirmed SARS-CoV-2 infection. Exclusion criteria were: (1) age under 18 years, (2) unclear SARS-CoV-2 vaccination history, (3) receipt of other types of vaccines besides inactivated vaccines, (4) unclear or wrong contact information. A total of 8945 patients were included in this follow-up study, which contained the discharged patients from three COVID-19 designated hospitals between April 2022 and June 2022.
The study scheduled two follow-up visits: the first visit is to evaluate the recovery status and immune response of Omicron infected patients after half a year later of the primary infection through telephone and hospital surveys. The second visit was only conducted by telephone to investigate the reinfection at the end of the first Omicron wave in China. According to the chest imaging and clinical characteristics, the disease severity in our study was classified into two types: non-pneumonia and pneumonia.
In addition, COVID-19 survivors with chronic respiratory disease (including asthma, chronic bronchitis, COPD, and lung carcinoma) were excluded in the first visit. Those COVID-19 participants who completed the telephone survey in the first visit were then matched by sex, age (3 years) and follow-up time (
3 weeks) to select common respiratory tract infection groups. Specifically, the pneumonia cases were matched at a ratio of 1:1, while the non-pneumonia participants were matched at a ratio of 3:1 with their respective controls (). The non-pneumonia group was stratified by sampling by age to select their controls. The appointment for the follow-up visit was set by trained medical staff via telephone. Forty percent of telephone records were quality controlled by random sampling. Venous blood samples were collected from all participants who were willing to attend follow-up appointments for measuring the blood routine, systematic inflammation, chest CT and SARS-CoV-2 neutralizing antibody and T cell response.
Figure 1. The flowchart of this study. An overview of the study population is provided for both the telephone cohort and the sample cohort.
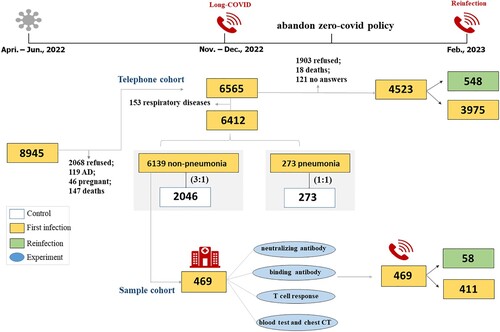
This population-based cohort study received approval from the ethics committee of Zhongshan Hospital affiliated to Fudan University (B2022-244R), Renji Hospital affiliated to Shanghai Jiaotong University, School of Medicine (RA-2022-601), and Pudong Hospital affiliated to Fudan University (WZ-22). The study was registered on www.chictr.org.cn (ChiCTR2200060003). And the Strengthening the Reporting of Observational Studies in Epidemiology (STROBE) checklists were used to guide transparent reporting (Table S1).
Procedures
Baseline characteristics and clinical information were acquired from the hospital’s electronic medical records. Definitions for the comorbidities are listed in Table S2. And vaccination history was obtained from the Shanghai Group Immunization System, which included comprehensive vaccination information of all vaccine recipients, such as the type, manufacturer, date, and doses of vaccines (Table S3). Verbal and written informed consent were obtained from the telephone visit and hospital visit at Zhongshan Hospital, respectively. For the first visit, a control group of 2319 adults with the common respiratory tract infection was created and followed up by telephone at the same time. The COVID-19 and control groups shared the same telephone questionnaires (Table S4).
We then followed 6565 patients again at the end of the first Omicron wave in China to investigate the reinfection by asking whether reinfection, the reinfection prof, and the clinical symptoms (Table S5). The results were collected by telephone.
The detailed follow-up schedule and quality control were in Tables S6-8. The definitions and measurements of persistent symptoms were in Table S9.
Experimental methods
SARS-CoV-2 binding antibodies in our study were measured by enzyme linked immunosorbent assay (ELISA) to quantify plasma IgG binding to Omicron BA.2 RBD. ELISA endpoint titres were defined as the highest dilution that yields an absorbance of 2-fold greater than the background value. The NAb response was quantified by pseudovirus (Omicron BA.2) based on the neutralization assay. Neutralizing ID50 (50% inhibitory dose) titers were calculated from the highest dilution resulting in a 50% reduction in relative light units (RLUs) to virus control wells after background (RLUs of no virus wells) subtraction.
T-cell response was analysed using a human ELISpot kit (BD Bioscience, #551849, USA) according to the manufacturer's protocol. Plate images were captured by Biospot Tablet Reader (ChampSpot III, Beijing Shengsheng Technology Co., LTD, China) and analysed for spot-forming cell (SFCs) counts.
IL-6 and PCT were analysed by the electrochemical luminescence method through IMMULITE/IMMULITE 1000 IL-6 (Siemens Healthcare Diagnostics Products Limited, United Kingdom) and Elecsys BRAHMS PCT (Roche Diagnostics GmbH, Germany), respectively. CRP was tested by immunoturbidimetry method through Tina-quant C-Reactive Protein IV (CRP4) (Roche Diagnostics GmbH, Germany).
The details of experimental methods were in the Supplement.
Statistical analysis
Demographic and clinical variables were expressed as n (%) for categorical variables and median (interquartile range, IQR) or mean (standard deviation, SD) for continuous variables after evaluation for normality. Characteristics of participants were stratified according to whether they had long COVID and the severity of acute illness. Categorical data were compared with the χ² test. Continuous variables were analysed with a t-test or a Mann–Whitney U test, as appropriate. The McNemar test was used to compare the difference in acute symptoms between the primary infection and reinfection. The lung CT images were calculated by a learning framework, named Uncertainty Vertex-weighted Hypergraph Learning (UVHL) [Citation14] method to assess the lung infection volume and infection ratio. The UVHL structure considers the uncertainty of each vertex individually and the learning process is conducted on an unequal space.
We employed multivariable Poisson regression models to investigate the fixed effects of risk factors associated with at least one symptom, fatigue, respiratory and mental symptoms. We adjusted for age, gender, disease severity, cigarette smoking, the numbers of comorbidity, the doses of vaccination, and the viral RNA shedding time. To estimate the hybrid immunity effectiveness against Omicron reinfection, besides multivariate model, propensity score based inverse probability of treatment weighting (IPTW) and propensity score adjustment methods were applied to reduce the effects of confounding. The propensity scores were estimated using a logistic regression model with included all characteristic covariates collected. The absolute standardized mean difference (SMD) values less than 0.1 were considered as covariates balanced (Figure S1). All tests were two-tailed and p-values of less than 0.05 were considered statistically significant. All analyses used R version 4.1.0.
Results
Cohort characteristics
A total of 8945 patients with Omicron COVID-19 were included from three designated hospitals during the Shanghai Spring epidemic of 2022. The first follow-up consisted of telephone and hospital surveys was conducted from November to December, 2022 (). For the first telephone survey, 6565 survivors were successfully followed, we finally compared the sequelae between 6412 COVID participants including 6139 non-pneumonia patients and 273 pneumonia patients and 2319 controls by excluding 153 patients with respiratory diseases (). The overall demographics of COVID-19 participants with different disease severity were shown in Table S10. The mean age of the enrolled participants was 49.2 (SD, 18.0) years, with 3100 (48.3%) females and 3312 (51.7%) males. 31.2% of patients had comorbidities (2003/6412), the most frequent being hypertension, diabetes, coronary heart disease, and carcinoma. Among 6412 patients, 2159 (33.7%), 1583 (24.7%), and 2670 (41.6%) patients had received incomplete, full, and booster COVID-19-inactivated vaccines, respectively. The median viral RNA shedding time was 12 (IQR 9–16) days (Table S10).
The demographics, comorbidities, and vaccination were similar across the followed and lost follow-up groups (Table S11), indicating 6412 COVID-19 cases were no distinct bias. Furthermore, we established an age-, sex-, and follow-up time-matched control group of individuals who had common respiratory tract infections to compare the proportions and severity of persistent symptoms (). Meanwhile, a hospital survey was conducted on 469 patients who were willing to participate in. There were statistically differences of baseline characteristics between hospital-visit population (469 patients) and lost to hospital-visit population (5943 patients), which includes younger age (46.3 (15.7) vs. 49.6 (18.0), p < 0.001), the less number of comorbidities and the proportion of incomplete vaccination (89 (18.6%) vs. 2070 (34.8%), p < 0.001) in hospital-visit population (Table S11).
Table 1. Characteristics of COVID-19 and control groups.
For the second telephone visit conducted at the end of the first Omicron wave in China from February to March 2023, patient characteristics were similar between successfully follow-up (4523 patients) and lost to follow-up (2042 patients) groups (Table S11). The 548 self-reported reinfection cases were identified, which had a higher proportion of female, young patients and incomplete vaccination compared with the non-reinfection individuals (Table S10).
The incidence, symptoms and risk factors of Omicron long COVID for the first visit
605 (9.4%) participants reported long COVID, which have a higher proportion of older individuals (52.4 (SD, 18.5) vs 49.0 (SD, 18.4), p < .001), current smokers (129 (21.3%) vs 972 (16.7%), p = .004), had more comorbidities (p < .001), more incomplete vaccination (240 (39.7%) vs 1919 (33.0%), p < .001) and a longer viral RNA shedding time (13 [IQR, 9-17] vs 12 [IQR, 8-15], p < .001) compared with non-long-COVID participants. Detailed demographics of participants are shown in Table S10. The generalized symptoms were reported with the highest proportion (7.5%, 479/6412), followed by the mental symptoms (7.0%, 446/6412), the respiratory symptoms (3.8%, 242/6412), and digestive symptoms (0.9%, 55/6412) (). The commonly self-reported symptomatic sequelae at the first visit were fatigue (6.5%, 418/6412), memory decline (5.2%, 333/6412), somnipathy (3.1%, 199/6412), and cough (2.3%, 146/6412) regardless of disease severity (). Pneumonia and the number of comorbidities with ≥2 had higher proportions of at least one persistent symptom compared with the non-pneumonia and non-comorbidity groups (Figure S2). Moreover, 8.0% (185/2319) of the matched control population felt not recovered, significantly lower than the COVID-19 population (13.9%, 892/6412) (). The proportions and severity of most recorded prevalent symptoms were significantly higher in the COVID-19 group than that in the control group ( and Table S12).
Table 2. Comparison of persistent symptoms among COVID-19 and common respiratory tract infection groups.
Then, we explored the risk factors that accounted for the long COVID after adjusting for the covariates that significantly differed between long-COVID and non-long-COVID groups (Table S10). Factors associated with at least one persistent symptom were female (RR, 1.39; 95% CI, 1.16-1.67), the disease severity during the acute illness (RR, 2.15; 95% CI, 1.66-2.76), current smoking (RR, 1.71; 95% CI, 1.26-2.28), the presence of comorbidities (2 comorbidities RR, 1.72,95% CI, 1.31–2.23; ≥ 3 comorbidities RR, 2.00, 95% CI, 1.48–2.66) and viral RNA shedding time (RR, 1.03; 95% CI, 1.02-1.05) (A). Similarly, the above risk factors were positively associated with fatigue, mental and respiratory symptoms (B-D). In addition, our results suggested that vaccination was not associated with a reduction in the incidence of long COVID ().
Figure 2. Factors associated with persistent symptoms. Risk of at least one sequelae (A), fatigue (B), mental (C), and respiratory symptoms (D) are plotted. Incident outcomes were assessed from viral RNA shedding to the end of the follow-up. RRs were calculated using multivariable Poisson regression adjusting for sex, age, BMI, disease severity during the infection, smoking status, doses of vaccination, the number of comorbidity, and viral RNA shedding time. Adjusted RRs (squares) and 95% CIs (error bars) are presented. RR = rate ratio.
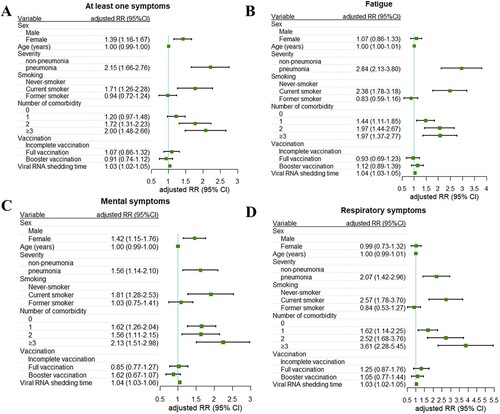
Results of the immune responses and hospital surveys from the first visit
A total of 469 patients came to the hospital for face-to-face health checks at the first visit. The NAb titres of full (72 [IQR, 36-156]) and booster vaccination (64 [IQR, 28-132]) groups were all significantly higher than those in the incomplete vaccination group (36 [IQR, 16-79]), the binding antibody titres exhibited the similar trends (1600 [IQR, 800-3200]) (A and S3A). However, there was no significant difference in T-cell responses among different vaccination doses (Figure S4A), which may due to limited temporal memory of T cells against SARS-CoV-2. No differences were found in terms of binding antibodies, and NAb titres between long COVID group and non-long-COVID group (B and S3B), as well as the laboratory tests (blood routine, C-reaction protein (CRP), Interleukin-6 (IL-6), procalcitonin (PCT)) and chest CT (Table S13).
Figure 3. The Omicron BA.2 NAb titres of 469 participants who came to the hospital. The NAb titres among participants with different doses of vaccination (A) and between long-COVID and non-long-COVID (B). A comparison of NAb between reinfection and non-reinfection (C) and stratified by doses of vaccination (D). Mann-Whitney U test was used to calculate P values. Nab = neutralizing antibody; NS. = no significance; *P < 0.05, **P < 0.01, ***P < 0.001.
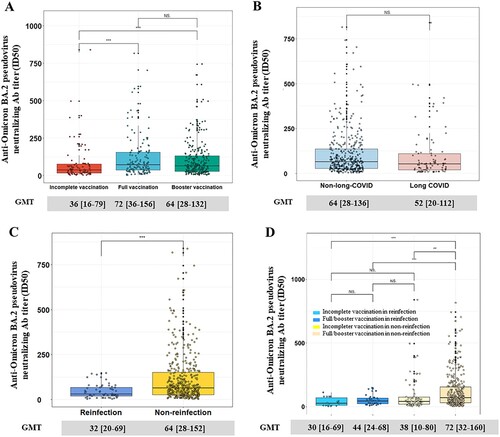
The incidence, clinical symptoms of the reinfection according to the second visit
Among 6565 completed the first follow-up, 4523 received reinfection investigation through the Internet and telephone at the end of the first Omicron wave in China. In which, 548 participants (12.1%, 548/4523) report Omicron reinfection that as at least two positive SARS-CoV-2 tests occurring in the same individual and separated by a period of at least 90 days (Tables S9-10). Their mean (SD) age was 47.3 (SD, 18.2) years, and 318 (57.8%) were females (Table S10). Overall, 80.7% of the reinfection patients reported alleviative or similar clinical symptoms compared with their primary infection (Figure S5). The greatest significant differences between the primary infection and reinfection groups were observed in respiratory system symptoms such as sputum (33.8% vs 26.3%), sore throat (38.9% vs 31.8%), runny nose (31.9% vs 21.5%), cough (56.8% vs 45.3%) and muscular soreness (33.8% vs 25.7%). However, the risk of progressing to pneumonia in the reinfection was not different from the primary infection (6.6% vs 5.3%) (Figure S5).
The effectiveness of hybrid immunity against reinfection
Specifically, the reinfection proportion of individuals who received the incomplete vaccination (14.8%) was higher than those who received full (10.3%) and booster vaccination (11.1%) (). In order to further evaluate the effectiveness of hybrid immunity in preventing reinfection, the results of inverse probability weighting (IPTW) method showed that full and booster vaccination had odd ratios (ORs) of 0.71 (95% CI, 0.63-0.81) and 0.77 (95% CI, 0.68-0.87) for reinfection, respectively, which were consistent in multivariate analysis and propensity score-adjusted analysis ().
Table 3. The efficacy of hybrid immunity in protecting from reinfection.
Out of the 469 participants who were admitted to the hospital, 58 were identified as reinfections, and 411 were non-reinfections based on the RT–PCR, IgG, IgM, and antigen test kit. The titres of NAb in non-reinfection individuals were significantly higher among those who received full or booster vaccination, compared to the reinfection individuals despite either incomplete (72 [32-160] vs. 30 [16-69], p < .001) or full/booster vaccination (72 [32-160] vs. 44 [24-68], p < .001) (C-D), verifying the above-mentioned population-based statistical analyses and strongly emphasizing that effective vaccination plus SARS-CoV-2 infection is associated with beneficial outcomes in NAb titres. While there was no significant difference in the binding antibody titres and T-cell responses between the reinfection and non-reinfection groups (Figures S4B-S4C).
Discussion
Our study, which includes a large general population in Shanghai, China, provides a more comprehensive understanding of the prevalence and impact of long-COVID of Omicron variant infection [Citation15–19]. Unlike the original SARS-CoV-2 variant with about 61% persistent symptoms at 6 months after initial COVID-19 illness [Citation20], our results showed a distinct decrease of only 9.4% of patients self-reported experiencing long COVID. Additionally, the high prevalence of persistent fatigue, mental symptoms, and dyspnea was specific to those with SARS-CoV-2 Omicron variant infection by comparing control groups with common respiratory tract infections. Women and those with pre-existing multi-morbidity were less likely to recover completely from COVID-19 in our study, which was consistent with previous studies [Citation21,Citation22]. Furthermore, our findings showed prolonged viral RNA shedding time is associated with long COVID, making it a valuable risk factor to consider. Additionally, the PHOSP-COVID collaborative group of the UK revealed IL-6 molecule was significantly increased in the moderate with cognitive impairment cluster at 5 months after SARS-CoV-2 original and Delta variants infection [Citation23], while the systemic inflammation biomarker CRP, PCT and IL-6 demonstrated no significant difference in the long COVID individuals in our study. Meanwhile, we found long COVID cases also have no distinct abnormal chest CT at half a year after Omicron infection. These clinical and imaging characteristics indicated the decreased long-term physical or cognitive impairment of Omicron variant infection. In our study, we pinpointed risk factors for long COVID, including being female, having multiple comorbidities, experiencing severe disease symptoms, and currently smoking. It seems that quitting smoking could serve as a proactive step against long COVID. Additionally, while inactivated vaccines might not directly lower the incidence of long COVID, they do provide defense against Omicron reinfections, thus indirectly decreasing the chances of contracting long COVID.
Several potential mechanisms behind long COVID have been proposed. For central and peripheral nervous system, an activation of microglia or auto-immune reactions may result in persistent neuroinflammation, which could account for neurocognitive impairment or mental health disorders [Citation24–26]. In addition, local microthrombosis caused by hypercoagulation or mitochondrial failure has also been suggested to contribute to symptoms [Citation27]. Abnormalities in the central nervous system such as brain hypometabolism might be involved in the onset of persistent fatigue [Citation28]. Lung vascular damage in micro-vessels may be engaged in long-term respiratory symptoms, possibly also lead to pulmonary hypertension [Citation29]. Furthermore, some long COVID patients may experience the damage of cellular energy production, possibly stemming from mitochondrial dysfunction [Citation30,Citation31]. Our study found no discernible differences in laboratory tests, antibody titers, or chest CT scans between the long COVID and non-long COVID groups. This might be attributed to our basic methodology. To delve deeper into the biological variations between these groups, more advanced techniques, such as nuclear magnetic resonance, single-cell transcriptomics and proteomics, may be necessary.
Currently, the dominant Omicron variant is characterized by increased immune evasion, which has led to a higher rate of reinfection. Our study found there was about a 12.1% reinfection rate at the end of the second Omicron wave in Shanghai, China. While the reinfection rates of the Omicron variant vary significantly across different countries. There was an 11.5% reinfection rate during the Omicron period in Iceland [Citation32]. Matt J. Keeling et al. predicted that the Omicron wave (from 2021 to 2022) was associated with 18% (16–20%) of cases being reinfections in England [Citation33]. A study of over 20,000 Omicron-infected individuals in Qatar has shown that the rate of reinfection after an initial Omicron infection is approximately 2.1% [Citation34]. The significant difference in Omicron reinfection rate across countries may be due to the different vaccination coverage, national condition, and research bias. Our findings also indicated that most people who experienced reinfection reported milder symptoms compared to their primary infection, which can potentially be attributed to the long-term NAb resulting from the primary infection and vaccination. According to a Portuguese study, individuals who have hybrid immunity resulting from infection with either Omicron BA.1 or BA.2, in conjunction with mRNA vaccination, experience considerably stronger protection against reinfection with BA.5 for a period of up to 8 months than those with vaccine-only immunity [Citation35]. Likewise, our study revealed that the hybrid immunity resulting from the inactivated vaccine also protect at some extent from reinfection although inactivated vaccine has limited effectiveness in preventing the primary infection. In addition, T-cell responses showed no distinction between reinfection and non-reinfection, suggesting the short-temporal immunity memory did not work against reinfection.
Our findings also indicated that female sex has a higher reinfection rate with the Omicron variant of COVID-19. Studies on previous virus variants suggested that severity and mortality of COVID-19 is higher in men than in women. However, women may have a higher risk of COVID-19 reinfection and developing long COVID [Citation36,Citation37]. The disparities between the sexes in terms of susceptibility to reinfection and vaccine response to SARS-CoV-2 likely stem from their distinct immune reactions[Citation38]. Moreover, more frequent social interactions or different types of social gatherings suggest that younger individuals may be more susceptible to reinfection, resulting in higher reinfection rates.
Our study has several strengths. To our knowledge, this is the first study to characterize the long-term health risks of Omicron infection in China as well as the hybrid immunity of the primary infection combined with inactivated vaccination status. The inclusion of common respiratory tract infection groups enabled us to demonstrate that the outcomes were not due to confounding and to further estimate the specific long-term sequelae of the Omicron variant. Differed from other studies that used the negative control (non-COVID infection) [Citation39], Our analysis also identified differences in symptoms and their duration between common respiratory tract infections and COVID-19. On the other hand, this is the first study to investigate the incidence, clinical symptoms, and protective factors of Omicron reinfection in China, as well as the role of inactivated vaccine in preventing from Omicron reinfection.
There are several limitations to our study. Firstly, we relied on a self-reported symptom questionnaire administered via telephone, which may have introduced bias due to the subjectivity of participants [Citation40,Citation41], which may introduce bias in that patients are less likely to provide information not on the questionnaires. Secondly, the patients willing to coming to the hospital all were non-pneumonia and younger than the overall population, therefore introducing the selection bias. Third, the reinfection rate may be underestimated due to the asymptomatic infection that people easily ignore. Finally, we did not follow the trajectories of persistent symptoms.
In conclusion, this longitudinal cohort study that included 6565 Omicron COVID-19 survivors revealed the relatively severe symptoms compared with the common respiratory tract infection. The study also provided valuable information about the hybrid immunity of the primary infection combined with inactivated vaccination preventing reinfection.
Supplemental Material
Download MS Word (1.2 MB)Acknowledgments
We are grateful to all the patients and volunteers who participated in the study. We appreciate the outstanding support made by Shanghai Huidr Information Technology Co., Ltd.
Disclosure statement
No potential conflict of interest was reported by the author(s).
Additional information
Funding
References
- Evans RA, McAuley H, Harrison EM, et al. Physical, cognitive, and mental health impacts of COVID-19 after hospitalisation (PHOSP-COVID): a UK multicentre, prospective cohort study. The Lancet Respiratory Medicine. 2021 Nov;9(11):1275–1287. doi:10.1016/S2213-2600(21)00383-0
- Global Burden of Disease Long CC, Wulf Hanson S, Abbafati C, et al, et al. Estimated global proportions of individuals With persistent fatigue, cognitive, and respiratory symptom clusters following symptomatic COVID-19 in 2020 and 2021. Jama. 2022 Oct 25;328(16):1604–1615. doi:10.1001/jama.2022.18931
- Sigfrid L, Drake TM, Pauley E, et al. Long COVID in adults discharged from UK hospitals after COVID-19: a prospective, multicentre cohort study using the ISARIC WHO clinical characterisation protocol. The Lancet Regional Health Europe. 2021 Sep;8:100186. doi:10.1016/j.lanepe.2021.100186
- Subramanian A, Nirantharakumar K, Hughes S, et al. Symptoms and risk factors for long COVID in non-hospitalized adults. Nat Med. 2022 Aug;28(8):1706–1714. doi:10.1038/s41591-022-01909-w
- Jacobson KB, Rao M, Bonilla H, et al. Patients With uncomplicated coronavirus disease 2019 (COVID-19) have long-term persistent symptoms and functional impairment similar to patients with severe COVID-19: A cautionary tale during a global pandemic. Clinical Infectious Diseases: An Official Publication of the Infectious Diseases Society of America. 2021 Aug 2;73(3):e826–e829. doi:10.1093/cid/ciab103
- Ballering AV, van Zon SKR, Hartman O, et al. Persistence of somatic symptoms after COVID-19 in The Netherlands: an observational cohort study. Lancet. 2022 Aug 6;400(10350):452–461. doi:10.1016/S0140-6736(22)01214-4
- Antonelli M, Pujol JC, Spector TD, et al. Risk of long COVID associated with delta versus omicron variants of SARS-CoV-2. Lancet. 2022 Jun 18;399(10343):2263–2264. doi:10.1016/S0140-6736(22)00941-2
- Baskett WI, Qureshi AI, Shyu D, et al. COVID-Specific Long-term sequelae in comparison to common viral respiratory infections: An analysis of 17 487 infected adult patients. Open Forum Infect Dis. 2023 Jan;10(1). ofac683. doi:10.1093/ofid/ofac683
- Jiang WQ, Li XS, Zhong WH, et al. Comparison of clinical characteristics in patients with coronavirus disease and influenza A in Guangzhou, China. World J Emerg Med. 2021;12(4):287–292. doi:10.5847/wjem.j.1920-8642.2021.04.006
- Pilz S, Theiler-Schwetz V, Trummer C, et al. SARS-CoV-2 reinfections: overview of efficacy and duration of natural and hybrid immunity. Environ Res. 2022 Jun;209:112911. doi:10.1016/j.envres.2022.112911
- Fonseca MHG, de Souza TFG, de Carvalho Araujo FM, et al. Dynamics of antibody response to CoronaVac vaccine. J Med Virol. 2022 May;94(5):2139–2148. doi:10.1002/jmv.27604
- Saure D, O'Ryan M, Torres JP, et al. Dynamic IgG seropositivity after rollout of CoronaVac and BNT162b2 COVID-19 vaccines in Chile: a sentinel surveillance study. Lancet Infect Dis. 2022 Jan;22(1):56–63. doi:10.1016/S1473-3099(21)00479-5
- Singh AK, Phatak SR, Singh R, et al. Humoral antibody kinetics with ChAdOx1-nCOV (covishield) and BBV-152 (covaxin) vaccine among Indian healthcare workers: A 6-month longitudinal cross-sectional coronavirus vaccine-induced antibody titre (COVAT) study. Diabetes Metab Syndr. 2022 Feb;16(2):102424. doi:10.1016/j.dsx.2022.102424
- Di D, Shi F, Yan F, et al. Hypergraph learning for identification of COVID-19 with CT imaging. Med Image Anal. 2021 Feb;68:101910. doi:10.1016/j.media.2020.101910
- Maestre-Muniz MM, Arias A, Mata-Vazquez E, et al. Long-Term outcomes of patients with coronavirus disease 2019 at One year after hospital discharge. J Clin Med. 2021 Jun 30;10(13):2945. doi:10.3390/jcm10132945
- Huang L, Li X, Gu X, et al. Health outcomes in people 2 years after surviving hospitalisation with COVID-19: a longitudinal cohort study. The Lancet Respiratory Medicine. 2022 Sep;10(9):863–876. doi:10.1016/S2213-2600(22)00126-6
- Yang XY, Hou C, Shen Y, et al. Two-Year health outcomes in hospitalized COVID-19 survivors in China. Jama Netw Open. 2022 Sep 1;5(9):e2231790. doi:10.1001/jamanetworkopen.2022.31790
- Huang CL, Huang LX, Wang YM, et al. 6-month consequences of COVID-19 in patients discharged from hospital: a cohort study (publication with expression of concern. See vol. 401, pg. 90, 2023). Lancet. 2021 Jan 16;397(10270):220–232. doi:10.1016/S0140-6736(20)32656-8
- Bellan M, Soddu D, Balbo PE, et al. Respiratory and psychophysical sequelae Among patients With COVID-19 four months after hospital discharge. Jama Netw Open. 2021 Jan 27;4(1). doi:10.1001/jamanetworkopen.2020.36142
- Blomberg B, Mohn KGI, Brokstad KA, et al. Long COVID in a prospective cohort of home-isolated patients. Nat Med. 2021 Sep;27(9):1607. doi:10.1038/s41591-021-01433-3
- Martimbianco ALC, Pacheco RL, Bagattini AM, et al. Frequency, signs and symptoms, and criteria adopted for long COVID-19: A systematic review. Int J Clin Pract. 2021 Oct;75(10):e14357. doi:10.1111/ijcp.14357
- Whitaker M, Elliott J, Chadeau-Hyam M, et al. Persistent COVID-19 symptoms in a community study of 606,434 people in England. Nat Commun. 2022 Apr 12;13(1):1957. doi:10.1038/s41467-022-29521-z
- Evans RA, Leavy OC, Richardson M, et al. Clinical characteristics with inflammation profiling of long COVID and association with 1-year recovery following hospitalisation in the UK: a prospective observational study. Lancet Resp Med. 2022 Aug;10(8):761–775. doi:10.1016/S2213-2600(22)00127-8
- de Erausquin GA, Snyder H, Carrillo M, et al. The chronic neuropsychiatric sequelae of COVID-19: The need for a prospective study of viral impact on brain functioning. Alzheimers Dement. 2021 Jun;17(6):1056–1065. doi:10.1002/alz.12255
- Song WJ, Hui CKM, Hull JH, et al. Confronting COVID-19-associated cough and the post-COVID syndrome: role of viral neurotropism, neuroinflammation, and neuroimmune responses. Lancet Resp Med. 2021 May;9(5):533–544. doi:10.1016/S2213-2600(21)00125-9
- Yong SJ. Persistent brainstem dysfunction in long-COVID: A hypothesis. Acs Chem Neurosci. 2021 Feb 17;12(4):573–580. doi:10.1021/acschemneuro.0c00793
- Ostergaard L. SARS CoV-2 related microvascular damage and symptoms during and after COVID-19: consequences of capillary transit-time changes, tissue hypoxia and inflammation. Physiol Rep. 2021 Feb;9(3. doi:10.14814/phy2.14726
- Guedj E, Campion JY, Dudouet P, et al. F-18-FDG brain PET hypometabolism in patients with long COVID. Eur J Nucl Med Mol I. 2021 Aug;48(9):2823–2833. doi:10.1007/s00259-021-05215-4
- Dhawan RT, Gopalan D, Howard L, et al. Beyond the clot: perfusion imaging of the pulmonary vasculature after COVID-19. Lancet Resp Med. 2021 Jan;9(1):107–116. doi:10.1016/S2213-2600(20)30407-0
- Clough E, Chean KT, Inigo J, et al. Mitochondrial dynamics in SARS-COV2 spike protein treated human microglia: implications for neuro-COVID. J Neuroimmune Pharm. 2021 Dec;16(4):770–784. doi:10.1007/s11481-021-10015-6
- Stefano GB, Ptacek R, Ptackova H, et al. Selective neuronal mitochondrial targeting in SARS- CoV-2 infection affects cognitive processes to induce ‘brain fog’ and results in behavioral changes that favor viral survival. Med Sci Monitor. 2021 Jan 25;27:e930886. doi:10.12659/MSM.930886
- Eythorsson E, Runolfsdottir HL, Ingvarsson RF, et al. Rate of SARS-CoV-2 reinfection during an omicron wave in Iceland. Jama Netw Open. 2022 Aug 3;5(8). doi:10.1001/jamanetworkopen.2022.25320
- Keeling MJ. Patterns of reported infection and reinfection of SARS-CoV-2 in England. J Theor Biol. 2023 Jan 7;556. doi:10.1016/j.jtbi.2022.111299
- Chemaitelly H, Ayoub HH, Tang PT, et al. Immune imprinting and protection against repeat reinfection with SARS-CoV-2. New Engl J Med. 2022 Nov 3;387(18):1716–1718. doi:10.1056/NEJMc2211055
- Malato J, Ribeiro RM, Fernandes E, et al. Stability of hybrid versus vaccine immunity against BA.5 infection over 8 months. Lancet Infect Dis. 2023 Feb;23(2):148–150. doi:10.1016/S1473-3099(22)00833-7
- dos Santos LA, de Gois PG, Silva AMF, et al. Recurrent COVID-19 including evidence of reinfection and enhanced severity in thirty Brazilian healthcare workers. J Infection. 2021 Mar;82(3):399–406. doi:10.1016/j.jinf.2021.01.020
- Abdelrahman MM, Abd-Elrahman NM, Bakheet TM. Persistence of symptoms after improvement of acute COVID19 infection, a longitudinal study. J Med Virol. 2021 Oct;93(10):5942–5946. doi:10.1002/jmv.27156
- Bechmann N, Barthel A, Schedl A, et al. Sexual dimorphism in COVID-19: potential clinical and public health implications. Lancet Diabetes Endo. 2022 Mar;10(3):221–230. doi:10.1016/S2213-8587(21)00346-6
- Hastie CE, Lowe DJ, McAuley A, et al. Outcomes among confirmed cases and a matched comparison group in the long-COVID in Scotland study (vol 13, 5663, 2022). Nat Commun. 2022 Oct 12;13(1):5663. doi:10.1038/s41467-022-33415-5.
- Mehandru S, Merad M. Pathological sequelae of long-haul COVID. Nat Immunol. 2022 Feb;23(2):194–202. doi:10.1038/s41590-021-01104-y
- Aiyegbusi OL, Hughes SE, Turner G, et al. Symptoms, complications and management of long COVID: a review. J Roy Soc Med. 2021 Sep;114(9):428–442. doi:10.1177/01410768211032850