ABSTRACT
Borna disease virus 1 (BoDV-1) was just recently shown to cause predominantly fatal encephalitis in humans. Despite its rarity, bornavirus encephalitis (BVE) can be considered a model disease for encephalitic infections caused by neurotropic viruses and understanding its pathomechanism is of utmost relevance. Aim of this study was to compare the extent and distribution pattern of cerebral inflammation with the clinical course of disease, and individual therapeutic procedures. For this, autoptic brain material from seven patients with fatal BVE was included in this study. Tissue was stained immunohistochemically for pan-lymphocytic marker CD45, the nucleoprotein of BoDV-1, as well as glial marker GFAP and microglial marker Iba1. Sections were digitalized and counted for CD45-positive and BoDV-1-positive cells. For GFAP and Iba1, a semiquantitative score was determined. Furthermore, detailed information about the individual clinical course and therapy were retrieved and summarized in a standardized way. Analysis of the distribution of lymphocytes shows interindividual patterns. In contrast, when looking at the BoDV-1-positive glial cells and neurons, a massive viral involvement in the brain stem was noticeable. Three of the seven patients received early high-dose steroids, which led to a significantly lower lymphocytic infiltration of the central nervous tissue and a longer survival compared to the patients who were treated with steroids later in the course of disease. This study highlights the potential importance of early high-dose immunosuppressive therapy in BVE. Our findings hint at a promising treatment option which should be corroborated in future observational or prospective therapy studies.
ABBREVIATIONS: BoDV-1: Borna disease virus 1; BVE: bornavirus encephalitis; Cb: cerebellum; CNS: central nervous system; FL: frontal lobe; GFAP: glial fibrillary acid protein; Hc: hippocampus; Iba1: ionized calcium-binding adapter molecule 1; Iba1act: general activation of microglial cells; Iba1nod: formation of microglial nodules; IL: insula; Me: mesencephalon; Mo: medulla oblongata; OL: occipital lobe; pASS: per average of 10 screenshots; patearly: patients treated with early high dose steroid shot; patlate: patients treated with late or none high dose steroid shot; Po: pons; So: stria olfactoria; Str: striatum
Introduction
Encephalitis caused by infection with Borna disease virus 1 (BoDV-1) is usually fatal in a wide range of mammals and as shown in 2018, also in humans [Citation1,Citation2]. Retrospective studies showed that some formerly etiologically unclear cases of encephalitis could be attributed to an infection with BoDV-1 resulting in bornavirus encephalitis (BVE) [Citation3,Citation4]. It can be assumed that BoDV-1 could account for a large proportion of the severe to fatal encephalitis cases of unknown cause in the virus-endemic areas, which includes eastern and southern parts of Germany [Citation4]. Nevertheless, the disease is extremely rare with up to date around 50 confirmed cases [Citation5]. Despite this rarity, understanding the pathomechanisms of BVE is of utmost relevance, as it can be considered a model disease for other encephalitic infections caused by neurotropic viruses.
Studies on the virus reservoir have shown that at least the bicoloured white-toothed shrew (Crocidura leucodon) forms the natural reservoir of BoDV-1 [Citation6–9]. Transmission to animal dead-end hosts, such as horses and sheep, is thought to occur from the environment via the olfactory pathway into the central nervous system (CNS) [Citation9–11]. In humans, the only known risk factor so far is living at the edge of a settlement or as a stand-alone position close to nature in virus-endemic areas [Citation12]. The transmission event itself is still unknown, but the olfactory pathway may play a role [Citation13].
Due to an insufficient awareness of the disease, the diagnosis is often significantly delayed [Citation14]. In addition, given the rareness of the disease, there are no evidence-based, specific therapy options, yet. The virus itself is non-cytolytic and tissue destruction is mediated by an immunopathogenic host response, at least by a CD8-T cell response [Citation15, Citation16]. Experimental studies in rodents successfully aimed to show that immunosuppressive therapy can inhibit or prevent the T cell-mediated immunopathology [Citation17–20]. In addition to T cells, local astrocytes might also play a role in promoting a pro-inflammatory state in the human brain in BVE [Citation21]. Immunosuppression was therefore seen as a possible key element in any successful treatment attempt [Citation21]. Circumstantial initial evidence exists showing that individuals with BoDV-1 encephalitis and iatrogenic immunosuppression had a tendency to live longer than non-immunosuppressed patients [Citation2, Citation4]. More recent observations in a young boy with BVE and very early immunosuppressive therapy in addition to virostatic treatment underscored this hypothesis as he achieved significant clinical improvement and prolonged survival [Citation13].
The aim of our study was to corroborate these findings through a thorough histomorphological analysis of BVE brains focusing on the degree of inflammatory infiltration, as well as glial and microglial activation in correlation to the clinical course and therapy regime.
Material and methods
Material
Autopsy material of seven patients (P1–P7) with confirmed BVE during 2013 and 2022 were included in this study. Their mean age of onset was 43 years (median: 43 years; range: 12–74 years). Reports of P1, P2 and P6 were already included in earlier studies [Citation22, Citation23] dealing with a general description of human BVE and an analysis of the magnet resonance imaging of BVE, respectively. For the purpose of comparability, ten regions (frontal lobe (FL), occipital lobe (OL), striatum (Str), hippocampus (Hc), insula (IL), mesencephalon (Me), pons (Po), medulla oblongata (Mo), cerebellum (Cb) and stria olfactoria (So)) were chosen (). The study was approved by the local ethics committees of the Technical University of Munich (number 577/19 S) and the Ludwig-Maximilians-University as the responsible ethics committee for the Augsburg University Hospital (number 23-0267).
Table 1. Regions available for histomorphological analysis.
Clinical data on the clinical course and therapy was retrieved from the patient records. The focus of our correlation with histology was on the outcome as a result of the disease and corresponding therapy. The time of death was defined as the end point and demonstrated in the histopathological correlate at this point.
Immunohistochemistry
For immunohistochemistry, 2 μm thick slides were dried at room temperature for 30 min. After epitope uncovering in pH 6.0 citrate buffer at 95°C for 30 min and H2O2 incubation, the slides were incubated with the primary antibody (anti-CD45: monoclonal, mouse, dilution 1:250; clone 2B11 & PD7/26; DCS, Germany) overnight at 4°C. Biotinylated secondary anti-mouse IgG (Vector Laboratories, USA) in a dilution of 1:400, followed by ABC reagent (Vector Laboratories, USA) were incubated for 30 min each. Subsequently, DAB reagent was added. BoDV-1 nucleoprotein was detected by immunohistochemistry using the monoclonal Bo18 antibody for an established avidin–biotin complex technique, as described earlier [Citation3, Citation24-26]. The immunohistochemistry for the glial fibrillary acid protein (GFAP) and the ionized calcium-binding adapter molecule 1 (Iba1) was performed using a fully automated staining system (BOND-III; Leica Biosystems, Wetzlar, Germany). The slides were incubated with primary antibodies anti-GFAP (monoclonal, rabbit, dilution 1:500; Cell Marque, USA) and anti-Iba1 (polyclonal, mouse, dilution 1:1000; Fujifilm Wako, Japan). The BOND Polymer Refine Detection Kit (Leica Biosystems, Germany) was used for antibody detection and counterstaining. For all immunostainings, counterstaining with haematoxylin was conducted. Positive controls served as quality assurance.
Image analysis
The immunohistochemically Bo18- and CD45-stained slides were digitalized using the Leica Aperio AT2 scanner. The images were recorded at a magnification of up to 200× and uploaded to the Aperio eSlides Manager. For each slide, 10 screenshots of 924 × 638 pixels equivalent with 925 × 640 µm and therefore 0.6 mm2 each were taken at 200× magnification. The screenshots of CD45 immunohistochemistry were analysed using the image processing program ImageJ (National Institutes of Health and University of Wisconsin). Various parameters, including brightness, saturation and hue as well as cell size and circularity were adjusted for optimal automatic detection of CD45-positive inflammatory cells. For quality assurance, regular manual recounts were carried out on a random basis. For each slide, the average of the 10 screenshots was calculated. The information number of lymphocytes per average of 10 screenshots (pASS) was used to evaluate the lymphocyte count. Due to interindividual variations in colour contrast of BoDV-1 immunohistochemistry, which were presumably caused by different degree of cell process infection, automated analysis was not possible. Therefore, both, positive and negative glial cells and neurons were counted manually. Again, the mean of the 10 screenshots was calculated.
For the immunohistochemistry of GFAP and Iba1, a semiquantitative score ranging from 0 to 5 adapted from previous publications, was determined using a standard light microscope (BX51, Olympus, Japan; with 0 = no glial or microglial, respectively, activation; 1 = very mild activation; 2 = mild activation; 3 = moderate activation; 4 = strong activation; 5 = extensive activation) [Citation3, Citation27–29]. For Iba1, two scores were estimated, one assessing the general activation (Iba1act) and the other assessing the formation of microglial nodules (Iba1nod).
Examples for all immunohistochemical stains can be seen in .
Figure 1. Examples for the immunohistochemical stains. (a) shows Bo18 immunohistochemistry for BoDV-1 nucleoprotein with Bo18-positive astrocytes (scale bar: 50 μm). In (b), an example of CD45 immunohistochemistry for lymphocytes is given (scale bar: 50 μm). (c) shows GFAP-positive reactive astrocytes (scale bar: 50 μm). In (d), microglial activation is demonstrated by Iba1 immunohistochemistry. Note the formation of microglial nodules (white arrows; scale bar: 200 μm).
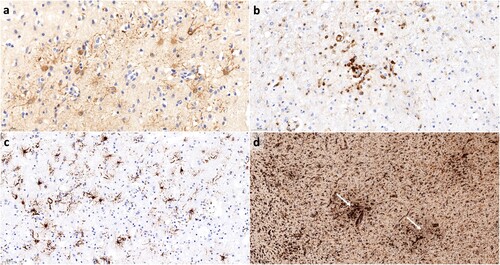
Statistical analysis
Statistical analysis was carried out using IBM SPSS Statistics (version 27.0). Since the data from the patient collective is not normally distributed, the nonparametric Mann–Whitney U test was used when analysing two variables. For the comparison of more than two variables, the Kruskal–Wallis-H test was performed. Correlations were analysed using the Spearman’s rho test. For all tests, statistical significance was defined as p < 0.05.
Results
Descriptive analysis of clinical courses of bornavirus encephalitis and individual therapy regimen
For this descriptive analysis, the onset of symptoms was chosen as the uniform starting point of the disease. Initially, all patients showed non-specific flu-like symptoms with high fever. Symptoms varied individually, but most complained of fatigue, cough, headache, dizziness, apathy and confusion. Later on, patients showed neurological symptoms, including a significant reduction in vigilance, as well as disorientation, ataxia, hallucinations, seizures and progressive loss of brain stem function. On average, patients were hospitalized on day 9 (range: day 5–14) after symptom onset. The patients developed their first seizure on average on day 12 (range: day 6–20). On day 13 (range: day 6– 19), most patients required protective intubation. Finally, all patients showed a persistent deep coma with electroencephalographic signs of severe encephalopathy, and in some cases, symptoms of increased intracranial pressure resulting in tonsillar herniation with central regulation failure. On average, the patients died 30 days (range 23–40; 95% CI [25.6, 35.1]; median: 30 days) after onset of symptoms.
Regarding the individual therapy regimen, P1 was excluded from the analysis, because of a whole different setting of infection. This patient was infected through an organ transplant, as reported before [Citation2, Citation23], and to avoid transplant rejection, treated with immunosuppressive therapy from the beginning. For the following analysis, timing and dose of immunosuppressive medications were taken into account. The most frequently used immunosuppression during the clinical course was a steroid shot for suspected autoimmune encephalitis with high doses of methylprednisolone, which was given in five of the six patients (P2, P3, P5, P6 and P7) over 5 days followed by low dose steroid therapy over several days (). In two patients (P2 and P3), the dose was 1 g per day, in one patient (P7), 0.5 g per day. For P5 and P6, the dose was not documented.
Figure 2. Overview of the correlation between timepoint of immunosuppression and extent of inflammation in the brain. Of note, P1 represents an interesting, but completely different setting of infection (infected organ transplant) and also dissimilar treatment situation with immunosuppression from the start to avoid a rejection reaction. Day 13 is marked as threshold for the grouping into patearly and patlate.
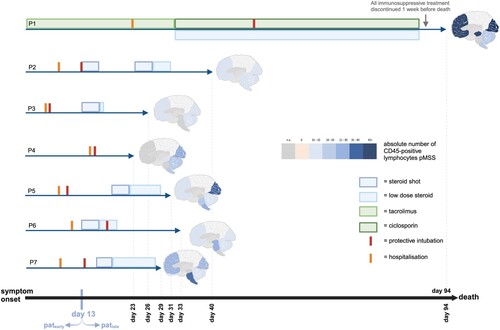
Regarding antiviral therapy, six patients (P2-P7) were treated with acyclovir due to clinically suspected encephalitis caused by herpes simplex virus in the meantime. It was administered on average 9.67 days (median = 9) after symptom onset for an average duration of 6.17 days (median = 6).
Therapy with antivirals ribavirin or favipiravir, for which antiviral activity against BoDV-1 was shown in experimental studies [Citation16,Citation30], were not administered in P1-P6 due to an only retrospective diagnosis of BVE. In one case (P7), favipiravir was administered at a very late stage on day 23–27 after symptom onset.
Spatial quantitative assessment of lymphocytes and BoDV-1-infected cells
The distribution pattern of lymphocytic infiltration of the CNS, as well as of the BoDV-1-infected neuronal and glial cells was evaluated in a quantitatively matter; GFAP and Iba1 positivity for glial and microglial, respectively, activation was assessed semiquantitatively. The results show interindividual patterns, especially regarding the CD45-positive cell distribution. In patients P2 and P3, the hippocampus showed the most significant inflammation (6.6 and 16.2 lymphocytes pASS). In P3, P4 and P5, the occipital cortex was particularly affected (9.6, 28.9 and 40.0 lymphocytes pASS). P1 shows the focus of inflammation in the frontal cortex (71.7 lymphocytes pASS), and in P6 and P7, this is in the brain stem (on average 25.5 and 34.1 lymphocytes pASS). The remaining regions appear more as a diffuse pattern. The different lymphocytic distribution patterns can be seen graphically in .
Figure 3. Distribution of BoDV-1-positive cells and lymphocytes. Demonstrated is the amount of BoDV-1-positive cells (left) and absolute number of CD45-positive lymphocytes pMSS (right) for all seven patients. Shaded in yellow is P1 as exeption regarding the setting of infection. Below, patearly are shaded in green, followed by patlate, shaded in red.
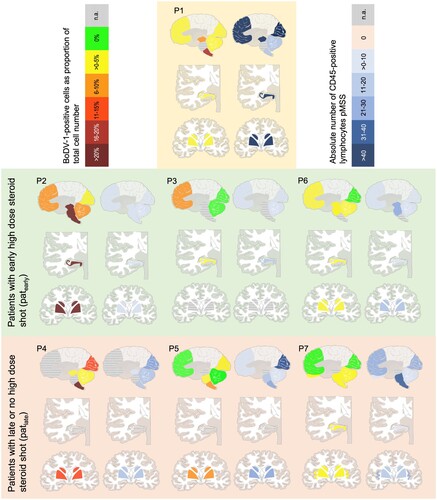
In contrast, when looking at the BoDV-1-positive glial cells and neurons, it is noticeable that in the human cases in this study there is massive viral involvement in the brain stem. As a limitation, some of these regions have not been preserved in P3 and P7. Of note, regarding P1 and P4, the more caudal, the more involved an anatomical region is. The medulla oblongata in particular stands out. In P2 and P4, over 20% of the total cell number (median 23%) in the medulla oblongata is infected and therefore significantly affected. In addition, P2 has to be particularly emphasized, showing the heaviest infected brain. P2 is the patient who survived the longest (40 days after the onset of symptoms), when excluding the iatrogenically infected P1. A strong involvement of the hippocampus with 37% and the striatum with 34% of BoDV-1-positive cells can be seen. Furthermore, the brain stem shows also many BoDV-1-positive cells (medulla oblongata 23%, pons 25%, mesencephalon 21% BoDV-1-positive cells). The least infected region on average is the cerebellum with 1.4% BoDV-1-positive cells (median 0.4%).
The strongest glial activation represented by GFAP positivity is seen in the insula (mean score 4.00). In contrast, the stria olfactoria and the striatum are the least affected regions (mean scores 0.00 and 1.86, respectively). Of note, again, an interindividual variation of the region with the strongest glial activation can be seen. For P2, for instance, an extensive glial activation (according to a score 5) is seen in the occipital lobe. In P1 and P3, the cerebellum is the region with the most glial activation; in P6 the insula.
A similar interindividual variety is demonstrated for the microglial activation. In average, the mesencephalon shows the strongest microglial activation (Iba1gen, mean score 4.00), whereas the most microglial nodules can be seen in the frontal lobe (Iba1nod, mean score 2.83). For both scores, the stria olfactoria is the region of least microglial activation (mean score 0 each), but is of limited informative value as the location was available for only one patient. Further regions of least microglial activation are the striatum, the hippocampus, the medulla oblongata and the insula (mean score 3,00 each) and for microglial nodules the pons (mean score 2.00).
Furthermore, we performed correlation analyses for each parameters (). A significant negative correlation between the amount of lymphocytes and the scores for GFAP and Iba1act can be seen (r = −0.314 and p = 0.038 for GFAP; r = −0.358 and p = 0.017 for GFAP). Additionally, GFAP and Ib1act show a highly significant positive correlation (r = 0.413 and p = 0.005). Hardly surprising, a highly significant positive correlation between Iba1act and Iba1nod is seen (r = 0.580 and p = <0.001). Interestingly, no correlation between BoDV-1-positive cells and lymphocytic count or glial/microglial activation is observed.
Table 2. Summary of the results of the correlation analyses.
Early high dose immunosuppression shows significant effects on the degree of inflammation
Interindividual differences in the neuropathological correlate were particularly evident when comparing patients treated early with high dose immunosuppression (patearly) to patients without immunosuppressive therapy or with immunosuppressive therapy applied late in the course of the disease (patlate). Again, P1 was excluded from this analysis due to a completely different infection mechanism. In patearly (P2, P3, and P6), the high dose steroid shots were given early (on day 13 after symptom onset; and (a)). For equal group size of patearly and patlate, a high dose steroid shot on day 13 was chosen as cut-off point. Furthermore, this is an obvious cut-off due to the fact that three patients which equals the half of the study population received their high-dose steroid on this point. In the three patients, an average of 6.9 lymphocytes pASS (median 5.1 lymphocytes pASS) was found across all regions. In contrast, P4 ((b)), who had not received any immunosuppressive therapy, as well as P5 and P7, who had received a late steroid shot on day 20 and 16, respectively, the latter with a lower dose of 500 mg intravenously per day, a clearly higher infiltration with lymphocytes was found (mean 19.1 lymphocytes pASS; median 16.1 lymphocytes pASS). This effect is highly significant when all data points for both groups (ergo all locations; n = 20 for both, patearly and patlate) are taken into account (p < 0.001). Even if this is a balanced distribution between the two groups, for some patients within a group, more locations are available compared to others, so a distortion cannot be excluded. When using the means for lymphocytic counts per patient, also a clear difference between the two groups is seen, which is not significant, though (p = 0.100).
Figure 4. Exemplary comparison of the clinical course and treatment between a patient of group patearly (P2; a) and a patient of group patlate (P4; b).
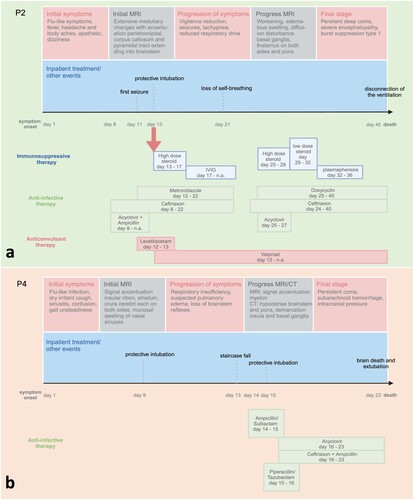
In P4, over 15 lymphocytes pASS per region were detected in almost all regions, with 28.9 lymphocytes pASS in the occipital cortex. In P5, an average of 21.9 pASS lymphocytes were also counted in almost all regions. Also in this case, the occipital cortex was significantly affected with 40,0 lymphocytes pASS. In P7, an average of 19.5 pASS lymphocytes was counted; the pons and medulla oblongata were particularly affected with 32.2 and 35.9, respectively, pASS lymphocytes.
P1, a case of BoDV-1 infection through an infected kidney transplant [Citation2], represents a special situation. As mentioned above, this patient was not included in our cohort analysis. The patient received tacrolimus up to 3 months before the onset of symptoms and was switched to ciclosporin and prednisolone from day 34 after the onset of symptoms, due to the assumption of tacrolimus-induced Guillain-Barré syndrome to prednisolone. Five days ante mortem, all therapeutic agents except the analgetic medication were discontinued due to a change to a palliative therapy concept. Immunohistochemical staining for CD45 revealed massive lymphocyte infestation in almost all brain regions. A possible explanation for this is the reactivation of the immune system. On average, 50.3 pASS lymphocytes were counted. The frontal cortex was the most affected region with 71.7 pASS lymphocytes.
The illustration of the courses of disease of all patients can be found in Supplementary Figure 1.
Early high dose immunosuppression shows clear effects regarding length of survival
Interestingly, the survival of patearly was extended in contrast to patlate (). Accordingly, patearly survived 33 days after the onset of symptoms on average (95% confidence interval (CI) [25.1, 40.9]; median 33 days). In contrast, patlate survived 6 days shorter with a mean of 27.7 days after onset of symptoms (95% CI [23.0, 32.4]; median 29 days). This difference in survival duration is not significant, though (p = 0.197).
Figure 5. Survival analysis showing an evident, though no significant, shorter survival of patlate compared to patearly.
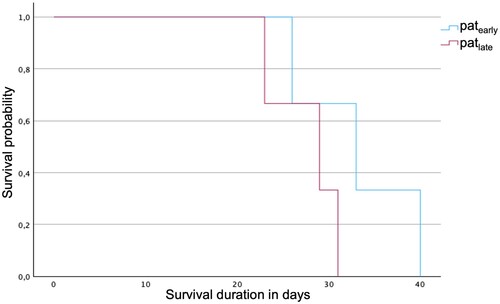
Substantial clinical improvement after early steroid shot was described for one of the patients of group patearly (P6). The 13-year-old patient received high doses of methylprednisolone from day 13 to day 17 after the onset of symptoms, followed by tapering therapy. From day 14, the patient’s condition worsened with the need for non-invasive ventilation. On day 17, the fifth day of high-dose steroid therapy, the patient was breathing spontaneously again and could be supported with an oxygen mask until day 19. Clinically, there was minimal improvement in findings with eye opening and a hint of a handshake. Nevertheless, protective intubation was carried out on day 19 due to the increasing lack of protective reflexes and substantial secretion. On day 23, the patient was extubated due to the onset of a cough. The cough reflex and cough were still inadequate, so the patient had to be reintubated two days later. This case shows that clinical improvement can be possible through early high-dose steroid therapy.
Discussion
BVE, only recently discovered in humans, is a devastating lymphocytic sclerosing panencephalitis that is fatal in most cases [Citation3, Citation4]. Up to date, essential information of risk factors, transmission setting, portal of virus entry and viral distribution mechanisms are lacking. In our analysis, a particularly intense presence of BoDV-1-positive cells was noticeable in the brain stem, mainly in the medulla oblongata. This observation had also been made in a magnet resonance imaging – histology correlation study [Citation22] and might indicate further portals of viral entry, besides the assumed olfactory pathway [Citation10, Citation11, Citation13, Citation31]. The gustatory tract with its afferent fibres from three cranial nerves (facial, glossopharyngeal and vagus nerve) and its connection via the nucleus tracti solitarii in the brain stem, would be of particular interest, for instance. For patient 1, who was iatrogenically infected via an infectious organ transplant [Citation2], an ascending infection via peripheral nerves and spinal cord to the brain is assumed. Whether a peripheral entry portal is possible in a natural setting, e.g. by contamination of wounds with infectious material, is a subject of debate.
Interestingly, no correlation between the lymphocytic count and amount of BoDV-1-positive cells was observed in our study. This could be explained through different local cell compositions in distinctive stages of inflammation. In regions where the virus has only recently entered, the strong lymphocytic reaction might still be lacking in contrast to regions which have been infected longer. In contrast to reports in animals, where a circumscribed virus presence has been shown [Citation32, Citation33], there is a broad virus distribution covering almost every region of the human brain. An obvious explanation for this constellation could be the significantly longer survival time of human patients due to intensive care and immunosuppressive treatment. Studies characterizing the lymphocytic population and immunological processes by, e.g. transcriptomic analyses in correlation with the viral load would help to evaluate this hypothesis. Also, the lacking correlation of BoDV-1-positive signal and lymphocytic count could be purely artificial due to the immunosuppressive therapy. Another explanation for this aspect could lie in the usage of the antibody Bo18 for the assessment of BoDV-1. Bo18 is a broadly applied and well established antibody. It is directed against the nucleoprotein of BoDV-1 [Citation24]. Even unlikely, it cannot be fully excluded that in some cells, only the epitopes of the viral phosphoprotein, but not the nucleoprotein are expressed. In this case, Bo18 would not mark these cells as BoDV-1-positive.
The cytotoxicity of BoDV-1 infection is mediated at least by virus-induced CD8-positive T lymphocytes [Citation34–36]. However, also brain astrocytes as additional source of pro-inflammatory mediators might likely play a role in human infections [Citation13, Citation21]. In the present study, we could show that patients treated with early high-dose steroids had a longer survival and a significantly reduced number of lymphocytes in their brains. Even if there is a balanced distribution of included numbers of regions between patearly and patlate, for some patients, more locations were available compared to others, so a distortion cannot be excluded. When using the means for lymphocytic counts per patient, also a clear, but not significant, difference between the two groups was seen.
Clinically, mild symptom improvement after steroid therapy was only described for P6. In a recently published report, it was shown in a 7-year-old patient that early (seven days after onset of symptoms) and strong immunosuppressive and virostatic therapy could temporarily lead to significant clinical improvement [Citation13]. The clinical and neuropathological findings of that study suggested that early immunosuppressive therapy could improve symptoms and influence survival in humans, and thus, appeared to be an important key factor in its treatment. Nevertheless, a specific antiviral treatment is also needed, as the virus establishes a persistent infection. As for that, individual experimental therapy with the synthetic guanidine nucleobase favipiravir and guanosine analogue ribavirin has been attempted [Citation16,Citation19]. However, it is currently still questionable to what extent antiviral therapy can really help to achieve an actual effect in vivo on such a neurotropic virus as BoDV-1, which also has a high affinity for intranuclear residence within the neurons. One possibility is that some kind of latency of the virus could be triggered and thus, the disease could be contained.
Nevertheless, the prognosis of BVE is still extremely poor and there is an urgent need for an effective therapy or prophylaxis such as vaccination. A life-long therapy with the mentioned antivirals in combination with extensive immunosuppressive, however, is not feasible due to the high risk of complications, including opportunistic infections for immunosuppression, and e.g. haemolytic anaemia for ribavirin [Citation13], besides other adverse effects. Furthermore, permanent immunosuppression could lead to a broader distribution of BoDV-1, also including non-nervous tissue with the possibility of virus shedding [Citation13], which would be very difficult to deal with at this point. In fact, there is a first report of virus shedding in a human in the form of viral RNA detection in tear fluid and saliva by PCR [Citation13]. The potential loss of viral neurotropism as result of immunosuppression was also shown in an experimental study analysing BoDV-1-infected rats treated with cyclosporin A. In the twentieth century, vaccination of horses and sheep was common in endemic areas. Due to concerns regarding adverse effects because of insufficient attenuation of the BoDV-1 live vaccines and the simultaneous decrease in horse population, the vaccines were abolished [Citation37]. Recent experimental studies showed that vaccination against BoDV-1 is possible with sufficient doses and schemes that include at least three vaccinations. A high antigen dose stimulated strong T cell responses which eliminate the virus and induce neutralizing antibodies. In an experimental setting, intracerebral injection of BoDV-1 was the most effective way to protect from Borna disease [Citation37]. With the fatal infections in humans, vaccination studies might rise up again, especially considering the current lack of potent therapeutics for this fatal disease.
Limitations of our study include the autopsy setting representing the final stage of disease after life-prolonging procedures. A correlation between clinical symptoms and the distribution pattern of neuropathological changes would be very desirable, but is unfortunately difficult to implement due to the limitations of the autopsy setting. Correlations with MRI or PET imaging at different stages of the disease would help for addressing this aspect. Furthermore, the cohort of seven patients is small, owing to the rareness of BVE. In addition, the role of antiviral treatment was collected, but not included in our analysis. Also, the documentation of the clinical courses and treatments varied due to the fact that patients were treated in different centres and in a time frame of nine years. Furthermore, the length of survival after intubation might not reflect the natural course of the disease, but is likely to be biased by time-varying decision and consulting processes with the patients’ relatives. This aspect must be taken into account when considering the result of our survival analysis.
Nevertheless, this study is a first correlation between clinical data and thorough histomorphological quantitative analysis with clear results that could assist for future decision making for the therapy of patients with BVE.
Concludingly, we corroborate recent clinical observations of prolonged patient survival under immunosuppressive therapy with a detailed histomorphological study assessing intensity of brain tissue inflammation. Immunosuppressive therapy might thus be an essential therapy pillar in the treatment of BVE. There is an urgent need for future studies evaluating viral suppression with a combination therapy of immunosuppression and specific antiviral drugs.
REV_SupFigure1
Download PNG Image (4.1 MB)Acknowledgements
The authors want to thank Sandra Baur, Juliane Stephan and Natalia Gerling for their excellent technical support. , and Supplementary Figure 1 were created with BioRender.com.
Disclosure statement
No potential conflict of interest was reported by the author(s).
Additional information
Funding
References
- Korn K, et al. Fatal encephalitis associated with Borna disease virus 1. N Engl J Med. 2018;379(14):1375–1377. doi:10.1056/NEJMc1800724
- Schlottau K, et al. Fatal encephalitic Borna disease virus 1 in solid-organ transplant recipients. N Engl J Med. 2018;379(14):1377–1379. doi:10.1056/NEJMc1803115
- Liesche F, et al. The neuropathology of fatal encephalomyelitis in human Borna virus infection. Acta Neuropathol. 2019;138(4):653–665.
- Niller HH, et al. Zoonotic spillover infections with Borna disease virus 1 leading to fatal human encephalitis, 1999–2019: an epidemiological investigation. Lancet Infect Dis. 2020;20(4):467–477.
- Bauswein M, et al. Human infections with Borna disease virus 1 (BoDV-1) primarily lead to severe encephalitis: further evidence from the seroepidemiological BoSOT study in an endemic region in southern Germany. Viruses. 2023;15(1):188. doi:10.3390/v15010188
- Bourg M, et al. Bicolored white-toothed shrews as reservoir for borna disease virus, Bavaria, Germany. Emerg Infect Dis. 2013;19(12):2064–2066.
- Durrwald R, et al. The bicolored white-toothed shrew Crocidura leucodon (HERMANN 1780) is an indigenous host of mammalian Borna disease virus. PLoS One. 2014;9(4):e93659. doi:10.1371/journal.pone.0093659
- Hilbe M, et al. Shrews as reservoir hosts of Borna disease virus. Emerg Infect Dis. 2006;12(4):675–677. doi:10.3201/eid1204.051418
- Nobach D, et al. Shedding of infectious borna disease virus-1 in living bicolored white-toothed shrews. PLoS One. 2015;10(8):e0137018.
- Gosztonyi G. Natural and experimental Borna disease virus infections–neuropathology and pathogenetic considerations. APMIS Suppl. 2008;116(124):53–57.
- Kupke A, et al. Intranasal borna disease virus (BoDV-1) infection: insights into initial steps and potential contagiosity. Int J Mol Sci. 2019;20:6.
- Pörtner K, et al. Risk factors for Borna disease virus 1 encephalitis in Germany – a case-control study. Emerg Microbes Infect. 2023;12(1):e2174778. doi:10.1080/22221751.2023.2174778
- Grosse L, et al. First detected geographical cluster of BoDV-1 encephalitis from same small village in two children: therapeutic considerations and epidemiological implications. Infection. 2023;51(5):1383–1398.
- Allartz P, et al. Detection of bornavirus-reactive antibodies and BoDV-1 RNA only in encephalitis patients from virus endemic areas: a comparative serological and molecular sensitivity, specificity, predictive value, and disease duration correlation study. Infection. 2024;52(1): 59–71.
- Lee BJ, et al. Ribavirin inhibits Borna disease virus proliferation and fatal neurological diseases in neonatally infected gerbils. Antiviral Res. 2008;80(3):380–384. doi:10.1016/j.antiviral.2008.08.001
- Tokunaga T, et al. Antiviral activity of favipiravir (T-705) against mammalian and avian bornaviruses. Antiviral Res. 2017;143:237–245. doi:10.1016/j.antiviral.2017.04.018
- Hallensleben W, et al. Borna disease virus-induced neurological disorder in mice: infection of neonates results in immunopathology. J Virol. 1998;72(5):4379–4386. doi:10.1128/JVI.72.5.4379-4386.1998
- Noske K, et al. Virus-specific CD4+ T cells eliminate borna disease virus from the brain via induction of cytotoxic CD8+ T cells. J Virol. 1998;72(5):4387–4395. doi:10.1128/JVI.72.5.4387-4395.1998
- Stitz L, Bilzer T, Planz O. The immunopathogenesis of Borna disease virus infection. Front Biosci. 2002;7:541–555. doi:10.2741/A793
- Stitz L, Sobbe M, Bilzer T. Preventive effects of early anti-CD4 or anti-CD8 treatment on Borna disease in rats. J Virol. 1992;66(6):3316–3323. doi:10.1128/jvi.66.6.3316-3323.1992
- Rauch J, et al. Human Borna disease virus 1 encephalitis shows marked pro-inflammatory biomarker and tissue immunoactivation during the course of disease. Emerg Microbes Infect. 2022;11(1):1843–1856. doi:10.1080/22221751.2022.2098831
- Finck T, et al. Bornavirus encephalitis shows a characteristic magnetic resonance phenotype in humans. Ann Neurol. 2020;88(4):723–735.
- Liesche-Starnecker F, et al. Hemorrhagic lesion with detection of infected endothelial cells in human bornavirus encephalitis. Acta Neuropathol. 2022;144(2):377–379. doi:10.1007/s00401-022-02442-3
- Haas B, Becht H, Rott R. Purification and properties of an intranuclear virus-specific antigen from tissue infected with Borna disease virus. J Gen Virol. 1986;67 (Pt 2):235–241.
- Herden C, Schluesener HJ, Richt JA. Expression of allograft inflammatory factor-1 and haeme oxygenase-1 in brains of rats infected with the neurotropic Borna disease virus. Neuropathol Appl Neurobiol. 2005;31(5):512–521. doi:10.1111/j.1365-2990.2005.00668.x
- Werner-Keiss N, et al. Restricted expression of Borna disease virus glycoprotein in brains of experimentally infected Lewis rats. Neuropathol Appl Neurobiol. 2008;34(6):590–602. doi:10.1111/j.1365-2990.2008.00940.x
- Holzgreve A, et al. Psma expression in glioblastoma as a basis for theranostic approaches: a retrospective, correlational panel study including immunohistochemistry, clinical parameters and PET imaging. Front Oncol. 2021;11:646387. doi:10.3389/fonc.2021.646387
- Liesche-Starnecker F, et al. Visualizing cellularity and angiogenesis in newly-diagnosed glioblastoma with diffusion and perfusion MRI and FET-PET imaging. EJNMMI Res. 2021;11(1):72. doi:10.1186/s13550-021-00817-3
- Schon S, et al. Imaging glioma biology: spatial comparison of amino acid PET, amide proton transfer, and perfusion-weighted MRI in newly diagnosed gliomas. Eur J Nucl Med Mol Imaging. 2020;47(6):1468–1475. doi:10.1007/s00259-019-04677-x
- Stitz L, et al. A functional role for neutralizing antibodies in Borna disease: influence on virus tropism outside the central nervous system. J Virol. 1998;72(11):8884–8892. doi:10.1128/JVI.72.11.8884-8892.1998
- Ludwig H. The biology of bornavirus. APMIS Suppl. 2008;116(124):14–20.
- Furstenau J, et al. Borna disease virus 1 infection in alpacas: Comparison of pathological lesions and viral distribution to other dead-end hosts. Vet Pathol. 2024;61(1):62–73. doi:10.1177/03009858231185107
- Kinnunen PM, et al. Epidemiology and host spectrum of Borna disease virus infections. J Gen Virol. 2013;94(Pt 2):247–262.
- Chevalier G, et al. Neurons are MHC class I-dependent targets for CD8 T cells upon neurotropic viral infection. PLoS Pathog. 2011;7(11):e1002393. doi:10.1371/journal.ppat.1002393
- Hausmann J, et al. T cell ignorance in mice to Borna disease virus can be overcome by peripheral expression of the viral nucleoprotein. Proc Natl Acad Sci U S A. 1999;96(17):9769–9774. doi:10.1073/pnas.96.17.9769
- Stitz L, et al. Transforming growth factor-beta modulates T cell-mediated encephalitis caused by Borna disease virus. Pathogenic importance of CD8+ cells and suppression of antibody formation. J Immunol. 1991;147(10):3581–3586. doi:10.4049/jimmunol.147.10.3581
- Dürrwald R, et al. Vaccination against borna disease: overview, vaccine virus characterization and investigation of live and inactivated vaccines. Viruses. 2022;14:12.