ABSTRACT
The Orthopoxvirus (OPXV) genus of the Poxviridae includes human pathogens variola virus (VARV), monkeypox virus (MPXV), vaccinia virus (VACV), and a number of zoonotic viruses. A number of Bcl-2-like proteins of VACV are involved in escaping the host innate immunity. However, little work has been devoted to the evolution and function of their orthologues in other OPXVs. Here, we found that MPXV protein P2, encoded by the P2L gene, and P2 orthologues from other OPXVs, such as VACV protein N2, localize to the nucleus and antagonize interferon (IFN) production. Exceptions to this were the truncated P2 orthologues in camelpox virus (CMLV) and taterapox virus (TATV) that lacked the nuclear localization signal (NLS). Mechanistically, the NLS of MPXV P2 interacted with karyopherin α-2 (KPNA2) to facilitate P2 nuclear translocation, and competitively inhibited KPNA2-mediated IRF3 nuclear translocation and downstream IFN production. Deletion of the NLS in P2 or orthologues significantly enhanced IRF3 nuclear translocation and innate immune responses, thereby reducing viral replication. Moreover, deletion of NLS from N2 in VACV attenuated viral replication and virulence in mice. These data demonstrate that the NLS-mediated translocation of P2 is critical for P2-induced inhibition of innate immunity. Our findings contribute to an in-depth understanding of the mechanisms of OPXV P2 orthologue in innate immune evasion.
Introduction
The Orthopoxvirus (OPXV) genus has more than 10 member species, including variola virus (VARV) the cause of smallpox, vaccinia virus (VACV), cowpox virus (CPXV), camelpox virus (CMLV), taterapox virus (TATV), monkeypox virus (MPXV), and several others [Citation1]. VACV is the vaccine that was used to prevent smallpox, a disease declared eradicated by the World Health Organization (WHO) in 1979 [Citation2]. From then until 2022, OPXVs caused only sporadic, limited disease in humans. But in 2022, MPXV, the cause of the zoonotic disease mpox, spread widely in humans, with sustained person-to-person transmission mediated by sexual contact, to cause a global outbreak that is ongoing today. MPXV remains a serious threat to global health and has emerged as the primary OPXV of public health concern. Although the WHO declared the end of public health emergency of international concern for MPXV, MPXV may continue to spill over from animal reservoirs into humans [Citation3]. Analysis of MPXV genomes from the 2022 to 2023 outbreak and comparison with similar viruses from West Africa has shown that MPXV has been acquiring single nucleotide polymorphisms [Citation4,Citation5]. Use of the anti-poxvirus drug tecovirimat for severe cases has resulted in the emergence of tecrovirimat-resistant MPXV strains [Citation6], and so additional anti-poxvirus drugs are needed.
The type I interferon (IFN) system is activated as a first response of the host’s immune system to viral infection. To replicate successfully in the host, OPXVs encodes numerous immunomodulatory proteins to evade and disrupt the production or action of IFNs or IFN-stimulated genes (ISGs) [Citation7]. One group of these immunomodulatory proteins that function inside the infected cell share a Bcl-2-like fold. However, despite structural similarity to Bcl-2, most of these VACV proteins do not regulate apoptosis, and instead inhibit one or more innate immune signalling pathway [Citation1,Citation8]. Members of this family include proteins A46, A49, A52, B14, C1, C6, C16 (VACV strain Copenhagen, VACV-Cop), F1, K7, N1, and N2. Protein A46 targets multiple TIR adaptors, including MyD88, MAL (TIRAP), TRIF, and TRAM, resulting in the inhibition of MAP kinase activation and TRIF-mediated IRF3 activation [Citation9,Citation10]. Protein A52 targets IRAK2 and TRAF6, and exhibits a more potent inhibitory effect on NF-κB activation than A46. Notably, A52 can activate p38 MAPK and enhance LPS-induced IL-10 production [Citation9,Citation11]. Protein A49 blocks NF-κB activation by molecular mimicry and prevents β-TrCP-mediated ubiquitylation of p-IκBα, thereby stabilizing p-IκBα so that p65 is retained in the cytoplasm [Citation12-14]. Protein B14 blocks NF-κB activation by binding to IKKβ and thereby prevents phosphorylation of IκBα [Citation15]. Protein C1 is not anti-apoptotic [Citation16] but promotes inflammasome activation [Citation17]. Protein C6 is a multifunctional IFN antagonist that inhibits IRF3 activation in 2 ways. First, it binds to the TBK-1 adaptor proteins TANK, SINTBAD and NAP1 [Citation18] and second, it induces proteasomal degradation of HDAC5, which promotes TRIF-dependent IRF3 activation [Citation19]. C6 also inhibits type I IFN-induced JAK-STAT signalling [Citation20] by inducing the proteasomal degradation of HDAC4 [Citation21]. Protein C16 (from VACV-Cop) widens VACV host range by antagonizing zinc-finger anti-viral protein (ZAP) [Citation22]. Protein F1 is an inhibitor of pro-apoptotic Bcl-2 proteins and also an inhibitor of caspase-9 [Citation23]. Similarly, N1 is both anti-apoptotic and also inhibits NF-κB activation [Citation24]. Protein K7 inhibits IRF3 activation by binding to DEAD box RNA helicase DDX3 [Citation25] and to Spir-1, an actin-binding protein [Citation26]. Lastly, protein N2 was described as a nuclear IRF3 inhibitor [Citation27] but its precise mechanism of action was not determined.
Orthologues of these VACV proteins are conserved in many OPXVs but most of these orthologues have not been studied. To investigate the relative importance of the OPVX Bcl-2 proteins, we studied the ability of Bcl-2-like proteins of VACV and MPXV to inhibit the expression of luciferase driven by the IFNB1 promoter after stimulation by HSV-1 infection. We found that VACV N2 (orthologue of MPXV P2) and MPXV P2 had the strongest inhibitory effect. Mechanistically, we demonstrate that MPXV P2 regulated antiviral innate immunity by interacting with KPNA2 and inhibiting the nuclear translocation of IRF3. Interestingly, a NLS in MPXV P2 that is conserved in most of OPXVs, but not CMLV and TATV, was critical for P2-mediated inhibition of IRF3 translocation and thereby crucial for suppression of innate immunity.
Material and methods
Reagents and antibodies
Rabbit monoclonal antibodies against TBK1 (E8I3G) (Cat # 38066), phospho-TBK1/NAK (Ser172) (D52C2) XP® (Cat # 5483), IRF3 (D6I4C) XP® (Cat # 11904), phospho-IRF3 (Ser396) (4D4G) (Cat # 4947), Flag (Cat # 14793), GST-Tag (91G1) (Cat # 2625), KPNA2 (Cat # 14372), His-Tag (D3I1O) XP® (Cat # 12698) and mouse monoclonal antibodies against Myc-Tag (9B11) (Cat # 2276), HA-Tag (6E2) (Cat # 2367), GFP (4B10) (Cat # 2955), GAPDH (D4C6R) (Cat # 97166) were from Cell Signalling Technology (USA). GST-KPNA2 and His-P2 fusion proteins were prepared in Escherichia coli strain BL21 and purified according to standard procedures. Anti-P2 and anti-A35 antibodies were generated by immunizing mice with Escherichia coli-expressed recombinant proteins. HRP-conjugated anti-mouse secondary antibody (Cat # A0216), HRP-conjugated anti-rabbit secondary antibody (Cat # A0208) were from Beyotime. Fluorescein (FITC) donkey anti-mouse IgG (Cat # 715-096-151), Rhodamine (TRITC) donkey anti-mouse IgG (Cat# 715-025-151), and Fluorescein (FITC) donkey anti-rabbit IgG (Cat # 711-095-152) were from the Jackson Laboratory. The plasmid prep purification Kit was from Genemark (China). Transcriptase kit was from Tiangen Biotech (Beijing, China). ANTI-FLAG M2 Affinity Agarose Gel (Cat # A4596), ivermectin (Cat # 1354309), and importazole (Cat # 401105) were from Sigma (USA). GST Magnetic Beads (MG-103) were from Biomed (Beijing, China). Primary antibodies were used at a dilution of 1:1000 and HRP-coupled anti-rabbit secondary antibodies were used at a dilution of 1:10,000 for immunoblot. Primary antibodies were used at a dilution of 1:200 for immunofluorescence.
Cell culture and transfection
HEK293 T, HeLa (expressing endogenous cGAS and STING) and Vero-E6 cells were obtained from the American Type Culture Collection (ATCC). HEK293 T, HeLa, and Vero-E6 cells were cultured in Dulbecco’s modified Eagle’s medium (Gibco, c11965500BT) supplemented with 10% fetal bovine serum (FBS; Gibco) and 1% (w:v) antibiotics (penicillin and streptomycin) at 37°C in 5% CO2. Transient transfection with the indicated plasmids was performed using a transfection reagent (YEASEN, 40802) according to the manufacturer’s instructions at a ratio of 1:3 (1 µg plasmid to 3 µl transfection reagent).
Viruses and animals
A stock of VACV Western Reserve (VACV-WR) was obtained from Dr. Luis Sigal (Thomas Jefferson University) and amplified and quantified as described [Citation28]. Initial stocks of the wild-type (WT) ECTV Moscow were obtained from ATCC (number VR-1374), and was gift from Min Fang (School of Life Sciences, Henan University, Henan, China). The recombinant VACV-N2L-ΔNLS and ECTV-EVM020-ΔNLS were generated by homologous recombination using the parental VACV-WR and ECTV Moscow genome, respectively, and a recombination transfer vector, as described previously [Citation29]. Donor DNA for homologous recombination consisted of a 50 bp left homology arm, DNA sequence encoding EGFP-N2L-ΔNLS or EGFP – EVM020-ΔNLS fusion protein, and 50 bp right homology arm. The successful insertion of EGFP-N2L-ΔNLS or EGFP-EVM020-ΔNLS was confirmed by sequencing. Herpes simplex virus type 1 (HSV-1) was kindly provided by Hongyu Deng (Institute of Biophysics, Chinese Academy of Sciences). All experiments with infectious VACV-WR, ECTV and HSV-1 were performed inside biosafety cabinets in the biosafety level 2 facility at the Institute of Microbiology, Chinese Academy of Sciences. To infect cells, the culture medium was removed, and the cells were washed twice with PBS (Gibco, 10010072). Serum-free culture medium containing the virus (MOI [multiplicity of infection] = 0.01) was added for 2 h and then the medium was replaced with 2% FBS culture medium and incubated until full cytopathic effect was observed or for the specific times indicated in the text.
Female BALB/c mice (6-8 weeks old) were purchased from Vital River, China. Mice were randomly grouped and were infected intraperitoneally with 5 × 106 PFU VACV-WT or VACV-N2L-ΔNLS, ECTV or ECTV-EVM020-ΔNLS in an animal biosafety level 2 room at the Institute of Microbiology, Chinese Academy of Sciences. The weight of each mouse was measured immediately before the challenge, and the day of the challenge was considered to be day 0. After challenge, mice were monitored for weight changes and survival on a daily basis until day 20 postchallenge. Mice were euthanized with CO2 when they had reached the ethical endpoint of 25% body weight loss. This study was approved and conducted strictly following the recommendations stated in the Guidelines for the Care and Use of Laboratory Animals issued by the Ethics Committee of the Institute of Microbiology, Chinese Academy of Sciences.
Plasmids
The full-length P2 and its mutants (P2-ΔNLS and P2-Mut) were cloned into both pcDNA3.1-N-Myc, pcDNA3.1-N-HA and pcDNA3.1-GFP vectors. cGAS, TBK1, IRF3, and IRF3-5D were synthesized by TSINGKE (Tsingke Biotechnology Co., Ltd) and cloned into the pCAGGS-vector. The Myc-STING plasmid was provided by Prof. Yingli Shang (Shandong Agricultural University, Taian, China). KPNA1, KPNA2, KPNA3, KPNA4, KPNA5, KPNA6, and KPNB1 were synthesized by TSINGKE (Tsingke Biotechnology Co., Ltd) and cloned into the pcDNA3-N-Flag vector. GST-tagged KPNA2 and His-P2 were synthesized by TSINGKE (Tsingke Biotechnology Co., Ltd) and cloned into PGEX-4T-1 and PET30a-His vectors, respectively. VACV-WR – C6L, C1L, N1L, N2L, K7R, F1L, A46R, A49R, A52R, and B14R were synthesized by TSINGKE (Tsingke Biotechnology Co., Ltd) and cloned into pcDNA3.1-Flag vector. MPXV-CB-C4L, D11L, D19L, P1L, P2L, C6R, C7L, A47R, and B12R were synthesized by TSINGKE (Tsingke Biotechnology Co., Ltd) and cloned into pcDNA3.1-Flag vector. VACV-WR-N2L, VACV-VTT-TN2L, VACV-VTT-TN2L + NLS (the 80–120 amino acid region containing NLS of VACV-WR-N2L was added to VACV-VTT-TN2L), and ECTV-EVM020 were synthesized by TSINGKE (Tsingke Biotechnology Co., Ltd) and cloned into both pcDNA3.1-N-HA and pcDNA3.1-GFP vectors. HSPV-OPG036, RPXV-021, OPVA-031, MPXV-P2L, CPXV-Q2L, VARV-P2L, TATV-031, CMLV-027, AKMV-034, and AKPV-034 were synthesized by TSINGKE (Tsingke Biotechnology Co., Ltd) and cloned into pcDNA3.1-GFP vector.
siRNA interference assay
All siRNA fragments were purchased from Tsingke (Tsingke Biotechnology Co., Ltd). The siRNA sequences were as follows: KPNA2 siRNA-1 sense: 5′-GCAUCAUGAUGAUCCAGAA-3′ and KPNA2 siRNA-1 antisense: 5′- UUCUGGAUCAUCAUGAUGC-3′. Scrambled siRNA (Invitrogen) was used as a negative control. The overexpression plasmid or siRNA was transfected into HeLa cells using Lipofectamine 2000 (Invitrogen, 11668019), according to the manufacturer's instructions. The total protein was isolated 24 h after transfection. Protein expression levels were assessed by immunoblotting.
Reverse transcription and qPCR
Total RNA was extracted from the cells using TRIzol reagent (Invitrogen, 15596026CN) according to the manufacturer's instructions. Each sample (2 μg) was digested with DNase I and reverse-transcribed into cDNA by using oligonucleotide (dT) primers. Mock reactions were performed in the absence of reverse transcriptase. Relative real-time PCR was performed to detect gene expression in HEK293 T and HeLa cells. SYBR Green (Takara, RR420A)-based real-time PCR was performed using an ABI 7500 instrument. The reaction was initiated at 95°C for 30 s, followed by 40 cycles of 95°C for 5 s and 60°C for 31 s. Each sample was amplified thrice. GAPDH was used as an internal control. Primer sequences used in this study were listed in Supplementary Table S3.
Immunofluorescence microscopy
HEK293 T or Vero-E6 cells were plated one day before transfection in 24-well plates at 5.0 × 104 cells/well. When the cells reached 70% confluency, 1 μg of plasmids was transfected per well of a 24-well plate. Before transfection, medium was changed to warmed Opti-MEM without phenol red (Life Technologies). Plasmid transfection was performed using transfection reagent (Cat# 40802 YEASEN, China) according to the manufacturer's instructions. Twenty-four hours after transfection, cells were washed 3 times with PBS, fixed in 4% paraformaldehyde for 30 min at room temperature, permeabilized with 0.5% Triton X-100 in PBS (PBST) for 20 min and stained with appropriate antibodies. Nuclei were stained with 5 μg/mL DAPI (Sigma). After staining, coverslips were analyzed using a Leica SP8 confocal microscope.
Coimmunoprecipitation and immunoblotting
Cells were grown in a 10-cm plate or 6-well plate and transfected with transfection reagent (Cat# 40802 YEASEN, China) for 24 h. Then the cells were harvested by lysis buffer (0.5% NP40, 150 mM NaCl, 20 mM HEPES (pH 7.4), 10% glycerol and 1 mM EDTA), and centrifuged at 12,000 rpm or 14,000 g for 10 min to remove the cell debris. The supernatants were incubated with ANTI-FLAG® M2 affinity gel (Sigma-Aldrich) at 4°C for 6 h. After five washes with wash buffer (0.5% NP40, 300 mM NaCl, 20 mM HEPES (pH 7.4), 10% glycerol and 1 mM EDTA), the bound proteins were eluted by boiling for 10 min in SDS protein loading buffer and analyzed by immunoblot analysis. Representative immunoblot images of at least three independent replicates were shown in the figures.
Glutathione S-transferase (GST) pull-down assay
GST pull-down assays were performed using a GST Magnetic Beads kit (Beijing Biomed Gen Technology Co., Ltd., Beijing, China). GST-KPNA2 protein and His-P2 protein were expressed in Escherichia coli strains Rosetta (DE3) for 6 h at 37 °C after expression was induced with IPTG (0.5 mmol/L). Finally, protein complexes were visualized by immunoblotting with the appropriate antibody.
Protein modelling and molecular docking
The P2 protein model was constructed by the Robetta prediction server (http://robetta.bakerlab.org) and AlphaFold2 with default parameters (use_amber = False, use_templates = False, msa_mode = MMseqs2 (UniRef + Environmental), model_type = auto, pair_mode = unpaired + paired, num_recycles = 3), while KPNA2 (4WV6) and IRF3 (2PI0) protein crystal structures were obtained from PDB database (https://www.rcsb.org/pdb) in pdb format. Protein docking analysis for KPNA2-P2 and KPNA2-IRF3 were done using ZDOCK tool (https://zdock.umassmed.edu/). Two-dimensional and three-dimensional structure interaction diagram for KPNA2-P2 and KPNA2-IRF3 were viewed using LigPlot (https://www.ebi.ac.uk/thornton-srv/software/LIGPLOT/) and PyMol software (http://www.pymol.org), display the details of the interacting amino acids, bridging water molecules and hydrogen bond distances.
Statistical analysis
All the results were presented as the mean ± SD. Student’s t test one-way ANOVA, and one-way repeated measurement analysis of variance (RMANOVA) were used to determine statistical significance, with P value < 0.05 being considered significant (* P < 0.05, **P < 0.01, ***P < 0.001). Statistical analyses are completed using Graphpad Prism 7.
Results
VACV N2 and MPXV P2 have the strongest ability to antagonize IFNβ-driven luciferase expression among Bcl-2-like proteins
It is well documented that many VACV Bcl-2-like proteins, such as A46, A49, A52, B14, C1, C6, F1, K7, N1, and N2, are involved in antagonizing host innate immune responses [Citation1,Citation8,Citation27], but the evolution and roles of most of these proteins in other OPXVs remains unknown. We analyzed the amino acid sequences of the Bcl-2-like protein orthologues of VACV-WR, and found that these proteins were relatively conserved among OPXVs. In addition, compared with VACV-WR, most of Bcl-2-like proteins of other OPXVs had N – and C-terminal deletions or insertions ((a) and Table S1), but the biological significance is still unclear. To compare the relative ability of VACV Bcl-2-like proteins to antagonize activation of the IFN-β promoter, the IFNB1 luciferase assay were performed in HSV-1-infected HeLa cells. Immunoblotting showed that each protein was expressed at a similar level enabling direct comparisons to be made. The results showed that VACV N2 exhibited the strongest ability to inhibit HSV-1-triggered activation of the IFN-β promoter among ten Bcl-2-like family proteins of VACV ((b)). SARS-CoV-2 non-structural protein 2(NSP2)was used as a negative control because it does not inhibit the IFN-β promoter activation [Citation30,Citation31]. Then the MPXV orthologues of eight VACV Bcl-2-like proteins, including D11 (orthologue of VACV-Cop C6), D19 (orthologue of VACV-Cop C1), P1 (orthologue of VACV-Cop N1), P2 (orthologue of VACV-Cop N2), C6 (orthologue of VACV-Cop K7), C7 (orthologue of VACV-Cop F1), A47 (orthologue of VACV-Cop A46), and B12 (orthologue of VACV-Cop B14) were constructed and tested. A49 and A52 of VACV-Cop have no orthologues in MPXV. Again, immunoblotting confirmed similar levels of protein expression and consistently, MPXV P2 (VACV-Cop N2 orthologue) had the most significant inhibition of pathway activation among these MPXV Bcl-2-like proteins ((c)). Moreover, HeLa cells expressing P2 were stimulated with HSV-1 infection and the levels of specific mRNAs were measured by RT-qPCR. We observed that P2 inhibited the expression of IFNB1, ISG15, and ISG20 (Figure S1). These data demonstrate that the MPXV P2 orthologue is the key OPXV Bcl-2-like protein for antagonizing transcription from the IFNβ promoter.
Figure 1. VACV N2 and MPXV P2 have the strongest ability to antagonize IFNB1 expression among Bcl-2-like proteins. (A) Amino acid analysis of Bcl-2-like protein orthologues of VACV-WR strain. Neighbour joining phylogenetic trees for poxvirus full-length coding sequences were constructed using Neighbour-joining (NJ) method in MEGA 6.0 with bootstrap value set to 1000. Asterisks (∗) indicate insertions and Asterisks (†) indicate codon deletions. N and C represent the N and C terminus, respectively. The number 1 indicates that the protein is present, while the number 0 indicates the absence of the protein. (B) Luciferase activity of lysates in HeLa cells transfected with IFN-β luciferase reporter (IFN-β-Luc) and Bcl-2-like proteins of VACV-WR or vector plasmid for 24 h, then infected with HSV-1 at MOI = 1.0 for 6 h. (C) Luciferase activity of lysates of HeLa cells that had been transfected with IFN-β luciferase reporter (IFN-β-Luc) and Bcl-2-like proteins of MPXV or vector plasmid for 24 h, then infected with HSV-1 at MOI = 1.0 for 6 h. Immunoblot analysis of the expression of each Bcl-2-like protein were shown under each bar chart. Data are shown as mean ± SD. ***P < 0.001 (One-way ANOVA followed by Dunnett’s test, vector and virus treatments as a control for multiple comparisons).
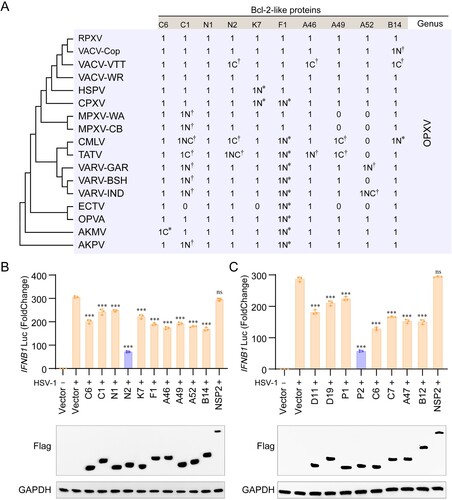
The nuclear localization signal (NLS) of monkeypox virus P2 is critical for its ability to localize to the nucleus and to antagonize IFN-β promoter activation
To investigate further whether P2 orthologues of other OPXVs had the similar functions, we studied the conservation of these proteins, including the N2 protein of VACV Tian Tan (VACV-VTT-TN2), the N2 protein of VACV-WR (VACV-WR-N2), protein OPG036 of HSPV (HSPV-OPG036), protein 021 of RPXV (RPXV-021), protein 031 of OPVA (OPVA-031), protein EVM020 of ECTV (ECTV-EVM020), protein P2 of MPXV West African strain (MPXV-WA-P2), protein P2 of MPXV Congo Basin strain (MPXV-CB-P2), protein Q2 of CPXV (CPXV-Q2), protein P2 of VARV India strain (VARV-P2), protein 031 of TATV (TATV-031), protein 027 of CMLV M96 strain (CMLV-027), protein 034 of Akhmetapox virus (AKMV-034), and protein 034 of Alaskapox virus (AKPV-034). Notably, the C-terminal regions of P2 orthologues are missing in VACV-VTT-TN2 (102-120 aa), TATV-031 (117-120 aa), and CMLV-027 (102-120 aa) ((a)) and in P2 this region is arginine-rich (102-119 aa) and contains a candidate NLS, identified using NLS Mapper (https://nls-mapper.iab.keio.ac.jp/cgi-bin/NLS_Mapper_form.cgi). We then examined whether the partial or complete loss of NLS in VACV-VTT-TN2, TATV-031, and CMLV-027 affected their cellular localization and antagonism of IFN-β transcriptional activation. As expected, OPXV P2 orthologues with the complete NLS all localized to the nucleus and significantly inhibited cGAMP-induced transcription of IFNB1 and the ISGs, ISG20 and MX1. In contrast, VACV-VTT-TN2, TATV-031 and CMLV-036 lost the ability to localize to the nucleus ((b)) and to suppress IFN-β and ISG transcription ((c)). Additionally, the cellular localization of MPXV P2 and VACV N2 was confirmed using confocal microscopy (Figure S2A, B) and nucleoplasmic separation experiments (Figure S2C, D) in P2-transfected-Vero-E6, HEK293 T, and HeLa cells, or in VACV-WR-infected Vero-E6 cells. P2 and N2 were distributed in both the nucleus and cytoplasm at 12 and 18 h post-transfection or infection, and thereafter accumulated in the nucleus at 24 and 48 h post-transfection or infection.
Figure 2. OPXV P2 protein orthologues with partial or complete loss of NLS lose the ability to translocate into the nucleus and to inhibit host innate immunity. (A) Neighbour-joining phylogenetic tree based on the MPXV P2 full length aa sequence and constructed using MEGA 6.0 with bootstrap value set to 1000 (left). The NLS aa sequence alignments of P2 orthologues in OPXVs by DNAMAN software (middle). The aa sequence homology of OPXVs compared with MPXV-CB-P2 (right). (B) The localization of P2 orthologues were determined by immunofluorescence microscopy in HEK293 T cells transfected with GFP-tagged P2 orthologue or GFP-Vector plasmid for 24 h. The nuclei were stained with DAPI. Scale bar: 3 µm. (C) mRNA levels of IFNB1 and ISG20 were determined by RT-q-PCR in HeLa cells transfected with GFP-tagged P2 orthologue or GFP-Vector plasmid for 24 h, and then stimulated with cGAMP for 6 h. Immunoblot analysis of the expression of P2 orthologues were shown under the bar chart. Data are shown as mean ± SD. ***P < 0.001 (One-way ANOVA followed by Dunnett’s test).
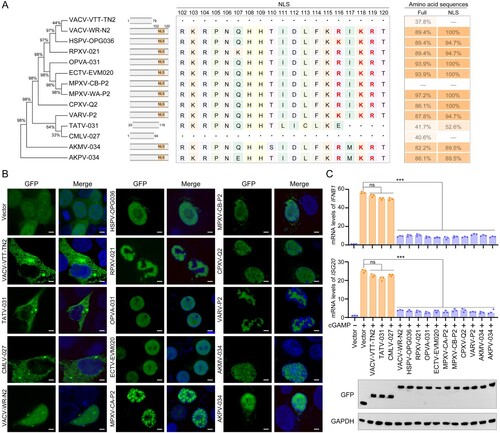
MPXV P2 antagonizes IRF3 nuclear translocation
To investigate how P2 might inhibit the cGAS-STING-IRF3 signalling pathway, HeLa cells were stimulated by addition or expression of cGAMP, cGAS, STING, TBK1, or IRF3-5D (a constitutively active form of IRF3) and mRNA levels of selected genes were analyzed by RT-qPCR. We found that the mRNA levels of IFNB1, ISG15 and ISG20 were significantly suppressed by P2 in cGAS-, STING1-, TBK1-, or IRF3-5D-transfected HEK293 T cells, indicating that P2 inhibited the pathway at or downstream of IRF3 phosphorylation ((a–c)). Further analysis showed that P2 overexpression did not affect the expression of cGAS, STING, TBK1, IRF3, p-TBK1, and p-IRF3, or the dimerization of IRF3 ((d–f)). The nuclear translocation of IRF3 is a prerequisite for IRF3-mediated gene transcription [Citation32]. Therefore, we examined the effect of P2 on IRF3 nuclear translocation in HEK293 T cells co-transfected with stimulator plasmids Myc-IRF3-5D, along with Flag-P2-expressing plasmid and found that P2 overexpression substantially suppressed nuclear accumulation of IRF3 ((g)). However, overexpression of P2 did not affect nuclear import proteins karyopherin-α3 (KPNA3) – and KPNA4-mediated [Citation33-35] p65 nuclear translocation and KPNA1-mediated [Citation36,Citation37] STAT1 nuclear translocation in TNF-α – or IFNα-treated HEK293 T cells (Figure S3), suggesting that P2 inhibited IFNβ transcription by specifically blocking IRF3 nuclear translocation. Taken together, these results reveal that P2 restricts IRF3 nuclear translocation, but not IRF3 production, phosphorylation and dimerization, thereby repressing IRF3-mediated transcription.
Figure 3. MPXV P2 antagonizes IRF3 nuclear translocation and innate immunity. (A–C) The mRNA levels of IFNB1 (a), ISG15 (b), and ISG20 (c) were determined by RT-q-PCR in HeLa cells transfected with Myc-P2 or Myc-vector plasmid, along with cGAMP, cGAS, STING1, TBK1, or IRF3-5D plasmid for 24 h. (D) The levels of cGAS, STING, TBK1, and IRF3 were detected by immunoblotting in HeLa cells co-transfected with Myc-P2 or Myc-Vector (top). Band intensities were determined by ImageJ software (bottom). (E) The phosphorylation levels of TBK1 and IRF3 were determined by immunoblotting in HEK293 T cells co-transfected with stimulator plasmids Flag-cGAS and His-STING, along with Myc-P2-expressing plasmid or Myc-vector for 24 h (left). Band intensities were determined by ImageJ software (right). (F) The dimerization of IRF3 was determined by immunoblotting in HEK293 T cells co-transfected with stimulator plasmids Myc-IRF3-5D, along with Flag-P2-expressing plasmid or Myc-vector for 24 h. (G) The localization of IRF3 was determined by immunofluorescence microscopy in HEK293 T cells co-transfected with stimulator plasmids Myc-IRF3-5D, along with Flag-P2-expressing plasmid or Flag-vector for 24 h (left), and the translocation percentage was calculated by dividing the number of cells with nuclear Myc-IRF3 by the total number of Myc-positive cells (right). The nuclei were stained with DAPI. Scale bar: 5 µm. Data are shown as mean ± SD. ***P < 0.001 (Multiple t-tests).
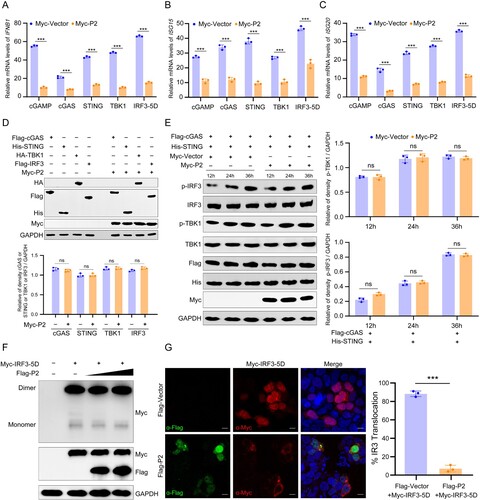
KPNA2 mediates the translocation of MPXV P2 into the nucleus
Classical nuclear import depends on a nuclear localization signal (NLS) in a cargo protein that is bound by a heterodimeric receptor consisting of a classical nuclear import receptor karyopherin alpha (KPNA) and a pore-targeting subunit karyopherin beta1 (KPNB1) [Citation38,Citation39]. Previous studies have shown that karyopherin α-2 (KPNA2) interacts with the NLS of IRF3 and mediates its nuclear import [Citation40]. To investigate which karyopherin mediated MPXV P2 nuclear import, ivermectin was used to block KPNA and KPNB interaction and so inhibit the nuclear transport facilitated by the prototypical NLS-mediated mechanism [Citation41]. KPNB1 can also recognize cargo directly and facilitate nuclear transport independent of KPNA [Citation42,Citation43], and such transport can be inhibited by the KPNB1 inhibitor importazole, a small molecule that specifically inhibits the KPNB family [Citation44]. Therefore, ivermectin and importazole were used to block the function of KPNAs and KPNB1, respectively. Confocal imaging showed that ivermectin, but not importazole, reduced nuclear localization of P2, suggesting that the nuclear import of P2 was dependent on KPNAs ((a)). Further, immunoprecipitation/mass spectrometry (IP/MS) was performed to screen P2-binding proteins. Numerous proteins can be visualized with silver staining ((b)). LC-MS/MS analysis showed that KPNA2 and KPNB1 were two of the nuclear transport-associated proteins that co-immunoprecipitated with P2 (Table S2). Consistently, the result of co-IP assay showed that P2 was able to co-precipitate with KPNA2 and KPNB1, but not KPNA1, KPNA3, KPNA4, KPNA5, and KPNA6 ((c)). Considering that the KPNB1 inhibitor importazole had no effect on the nuclear localization of P2, KPNB1 might be co-precipitated by binding to P2-precipitated KPNA2, rather than directly interacting with P2. Data from immunoprecipitation experiments corroborated the co-precipitation of VACV N2 and KPNA2 during VACV-WR infection ((d)). Furthermore, the result of pull-down assay showed that there was a direct interaction between GST-KPNA2 and His-P2 ((e)). To investigate the structural basis underlying the interaction between P2 and KPNA2, AlphaFold2 was used to predict the protein structure of P2 ((f)) and molecular docking analysis ((g)) was used to confirm the P2-KPNA2 interaction. The P2 region (102-119 aa) is a helix–loop–helix domain (silver area), which exactly covers the whole projected NLS area. To further confirm the role of KPNA2 on P2 nuclear import, siRNAs were selected to knockdown KPNA2 in HEK293 T cells. Nucleoplasmic separation experiments ((h)) and confocal imaging ((i)) showed that P2 nuclear localization was greatly reduced when KPNA2 was knocked down. Ivermectin also suppressed the nuclear accumulation of P2 and KPNA2 in HEK293 T cells ((j)). All these results demonstrate that KPNA2 mediates the nuclear import of MPXV P2.
Figure 4. KPNA2 mediates the translocation of MPXV P2 into the nucleus. (A) The localization of P2 was determined by immunofluorescence microscopy in HEK293 T cells treated with DMSO, ivermectin, or importazole for 6 h, then transfected with pEGFP-P2 plasmids for another 24 h. The nuclei were stained with DAPI. Scale bar: 3 µm. (B) Images of silver-stained gel of immunoprecipitated proteins using P2 as bait. HEK293 T cells were transfected with a plasmid expressing GFP-P2 fusion protein for 24 h, following by immunoprecipitation (IP) using antibodies against GFP. Samples were subjected to SDS-PAGE and visualized by silver staining. (C) Immunoblot analysis of lysates from HEK293 T cells co-transfected with Myc-P2 and Flag-KPNAs (KPNA1-6) or KPNB1 for 24 h, followed by immunoprecipitation with indicated antibodies. (D) Immunoblot analysis of lysates from BSC-1 cells infected by VACV-WR (MOI = 0.1) for 48 h, followed by immunoprecipitation with indicated antibodies. (E) The interaction between P2 and KPNA2 was determined by GST pull-down assay. Escherichia coli (BL21) expressed His-tagged P2 and GST-tagged KPNA2 recombinant proteins were purified, and protein-protein interactions were examined by GST-mediated pull-down assay, followed by immunoblotting with anti-His and GST antibodies. (F) Structure prediction of P2 viewed from the front and back view (rotated 180 degrees) using AlphaFold2. The NLS region is shown in silver. (G) Molecular docking of the interaction between P2 (silver) and KPNA2 (brown, PDB code: 4WV6). (H) The cellular localization of P2 was detected by nuclear-cytoplasmic separation assay in HEK293 T cells co-transfected with Myc-P2 and siRNA-KPNA2 or siRNA-NC plasmids. (I) The localization of P2 was determined by immunofluorescence microscopy in HEK293 T cells transfected with control siRNA or KPNA2 siRNA for 24 h, then transfected with pEGFP-P2 plasmids for another 24 h. The nuclei were stained with DAPI. Scale bar: 3 µm. (J) The localization of P2 was determined by immunofluorescence microscopy in HEK293 T cells treated with ivermectin for 6 h, then transfected with GFP-P2 plasmids and Flag-KPNA2 plasmids for another 24 h. The nuclei were stained with DAPI. Scale bar: 3 µm. Data are shown as mean ± SD.
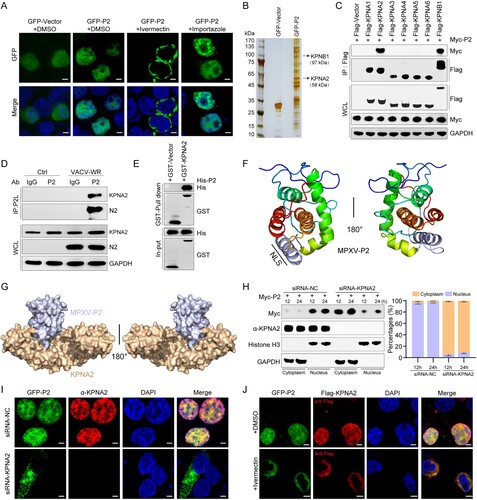
The NLS of MPXV P2 hijacks KPNA2 to inhibit the nuclear import of IRF3
Given that KPNA2 is required for the nuclear import of both P2 and IRF3, the NLS of those two proteins might competitively interact with KPNA2. To explore which amino acids of P2 and IRF3 interact with KPNA2, P2-KPNA2 and IRF3-KPNA2 interaction was analyzed by molecular docking. The results show that the amino acid R116, K118, and R119 of P2 might interact with E266, S105, and D270 of KPNA2 by hydrogen bonding ((a, b)), and amino acids R81 and K87 of IRF3 might interact with E266, D270, and S105 of KPNA2 by hydrogen bonding ((c, d)). This suggests that R116, K118, and R119 of P2 are critical for P2-KPNA2 interaction and P2 and IRF3 may competitively interact with the same region of KPNA2.
Figure 5. Two-dimensional and three-dimensional structure interaction diagram for P2-KPNA2 (PDB code: 4WV6) (A, B) and IRF3 (PDB code: 2PI0)-KPNA2 (PDB code: 4WV6) (C, D) were created using LigPlot (https://www.ebi.ac.uk/thornton-srv/software/LIGPLOT/) and PyMol software (http://www.pymol.org), displaying the details of the interacting aa, bridging water molecules and hydrogen bond distances. (E) A nucleocytoplasmic fractionation experiment was performed in HEK293 T cells transfected with GFP-P2-WT, GFP-P2-ΔNLS, GFP-P2-Mut, or GFP-Vector for 24 h, followed by immunoblot analysis. Antibodies against GAPDH or histone H3 were used to mark cytosolic or nuclear fractions, respectively. (F) Immunoblot analysis of lysates from HEK293 T cells co-transfected with Flag-KPNA2 plasmid and GFP-P2-WT, GFP-P2-ΔNLS, GFP-P2-Mut, or GFP-Vector plasmid for 24 h, followed by immunoprecipitation with indicated antibodies. (G) The localization of IRF3 was determined by immunofluorescence microscopy in HEK293 T cells co-transfected with stimulator plasmids Myc-IRF3-5D, along with HA-P2-WT, HA-P2-ΔNLS, HA-P2-Mut expressing plasmid or HA-vector, for 24 h. The nuclei were stained with DAPI. Scale bar: 3 µm. (H) Immunoblot analysis of lysates from HEK293 T cells co-transfected with Flag-KPNA2 and Myc-IRF3-5D plasmids, along with HA-P2-WT, HA-P2-ΔNLS, HA-P2-Mut, or HA-Vector plasmid for 24 h, followed by immunoprecipitation with indicated antibodies. (I) Immunoblot analysis of lysates from HEK293 T cells co-transfected with Flag-KPNA2 and Myc-IRF3-5D plasmids, along with HA-VACV-WR-N2, HA-ECTV-EMV020, HA-VACV-VTT-TN2, HA-VACV-VTT-TN2+NLS, or HA-Vector plasmid for 24 h, followed by immunoprecipitation with indicated antibodies. (J) The mRNA levels of IFNB1 and ISG20 were determined by RT-qPCR in HEK293 T cells co-transfected with HA-P2-WT, different doses of Flag-KPNA2 plasmid (0.5, 1.0, or 2.0 ug) for 24 h, and then stimulated with cGAMP for 6 h. Scale bar: 5 µm. The experiments (h and i) were repeated three times and Image J software was used for the grey value analysis. Vector treatment as a control for multiple comparisons. Data are shown as mean or mean ± SD. ***P < 0.001 (One-way ANOVA followed by Dunnett’s test).
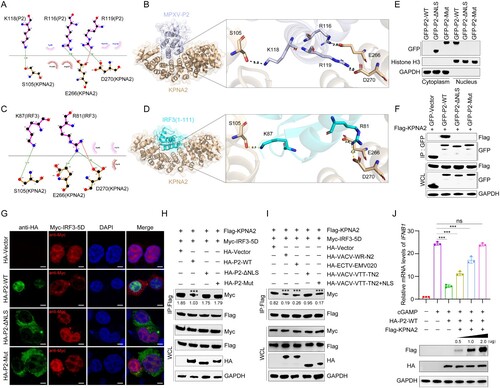
To determine whether this NLS (102-119 aa) is essential for mediating the nuclear localization of P2, P2 with NLS deletion (P2-ΔNLS) or NLS mutant (P2-mut, R116A, K118A, and R119A) were constructed. Nucleoplasmic separation experiment ((e)) and confocal imaging (Figure S4) showed that the deletion or mutation of P2 NLS altered its subcellular localization. Moreover, P2-ΔNLS and P2-mut no longer interacted with KPNA2 ((f)) and no longer inhibited the nuclear translocation of IRF3 in HEK293 T cells ((g)). P2 reduced the binding of IRF3 to KPNA2, but P2-ΔNLS and P2-mut did not ((h)). Similarly, N2 of VACV-WR and EMV020 of ECTV inhibited IRF3-KPNA2 interaction, but TN2 of VACV-VTT did not. Furthermore, the addition of NLS to VACV-VTT TN2 (VACV-VTT-TN2 + NLS) restored its ability to inhibit IRF3-KPNA2 interaction ((i)). At the same time, overexpression of KPNA2 remedied the inhibitory function of P2 on the IFN1B1 transcription in a dose-dependent manner ((j)). Taken together these results show that the NLS of P2 hijacks KPNA2 to inhibit KPNA2-mediated IRF3 nuclear import, thereby antagonizing IRF3-dependent transcription.
MPXV P2 NLS mutation or deletion abrogates the ability to inhibit antiviral innate immunity and to promote viral replication
Next, we investigated the effect of P2 NLS on VACV-triggered antiviral innate immune responses and viral replication. HeLa cells were transfected with HA-P2-WT, HA-P2-ΔNLS, HA-P2-Mut, or HA-vector plasmid, and then stimulated by infection with VACV-WR. The mRNA levels of IFNB1 ((a)), ISG20 ((b)) and ISG56 ((c)) were significantly increased after VACV infection, which was antagonized by overexpressing P2-WT, but not P2-ΔNLS or P2-Mut. Similarly, overexpression of P2 promoted VACV-WR expression of protein A35 in a dose-dependent manner ((d)), whereas P2-ΔNLS and P2-Mut did not ((e)). Similarly, P2, but not P2-ΔNLS and P2-Mut, enhanced A35 mRNA level ((af), and yield of infectious virus ((g)). Together, compared with P2-WT, P2-ΔNLS and P2-Mut lose the ability to inhibit antiviral innate immunity and to promote viral replication.
Figure 6. Protein P2 inhibits innate immune activation to facilitate viral replication. (A–C) The mRNA levels of IFNB1 (a), ISG15 (b), and ISG20 (c) were determined by RT-qPCR in HeLa cells transfected with HA-P2-WT, HA-P2-ΔNLS, HA-P2-Mut, or HA-vector plasmid for 24 h, and then stimulated with VACV-WR (MOI = 0.1) for 12 h. (D) Immunoblot analysis of VACV-WR-A35 in HeLa cells transfected with different dose of HA-P2 WT or HA-Vector plasmid for 24 h, followed by infection with VACV-WR (MOI = 0.1) for 24 h (top). Band intensities were determined by ImageJ software (bottom). (E–G) HeLa cells were transfected with HA-P2-WT, HA-P2-ΔNLS, HA-P2-Mut, or HA-vector plasmid for 24 h, followed by infection with VACV-WR (MOI = 0.1) for 24 h. The protein levels of VACV-WR-A35 were measured by immunoblotting (e, top). Band intensities were determined by ImageJ software (e, bottom). The mRNA levels of VACV-WR-A35R were measured by RT-qPCR (F). Infectious viral titers were obtained by plaque assays from the collected supernatant (G). Data are shown as mean ± SD. **P < 0.01 and ***P < 0.001 (One-way ANOVA followed by Dunnett’s test).
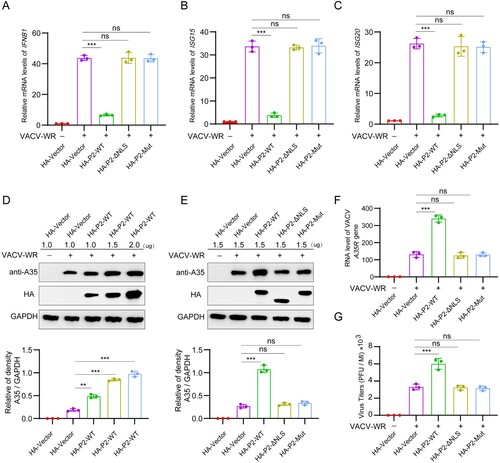
Deletion of NLS from the P2 orthologue (N2) in VACV-WR attenuates viral replication and virulence
To further investigate the function of the NLS in P2 and orthologues, we constructed a recombinant VACV-WR that had N2 fused to EGFP and lacking the N2 NLS (VACV-N2L-ΔNLS) ((a) and S5A). This virus was then used together with wild-type VACV-WR (VACV-WT) to infect HeLa cells and the levels of mRNA for IFNB1 and several ISGs were measured. Compared with VACV-WT, the VACV-N2L-ΔNLS induced higher levels of IFNB1 and ISGs ((b)), and reduced virus protein expression and yield of infectious virus ((c, e)). To investigate whether the attenuation completely dependent on type I IFN, we determined the replication of virus in Vero-E6 cells, which lack IFN-alpha and IFN-beta genes, and found that NLS deletion no longer affected virus replication ((d)). Moreover, overexpression of N2 protein restored the replication ability of VACV-N2L-ΔNLS ((f)). Meanwhile, the virulence of VACV-WT and VACV-N2L-ΔNLS in mice infected intranasally was examined ((g)). VACV-N2L-ΔNLS infection resulted in lower weight loss ((h)), lower mortality rates ((i)) and higher levels of mRNA for Ifnb1 and Isgs than VACV-WT ((j)). Moreover, the level of A35 mRNA ((k)), titers of infectious virus in lung, spleen and liver ((l)), and amount of virus protein measured by immunohistochemistry ((m)) were all reduced after infection by VACV-N2L-ΔNLS-infected compared to VACV-WR. H&E staining showed that the lung injury in the VACV-N2L-ΔNLS-infected mice was significantly less than that in the VACV-WT-infected mice ((n)). In addition, a recombinant ectromelia virus (ECTV) with EVM020 NLS deletion was constructed by homologous recombination (ECTV-EVM020-ΔNLS) by homologous recombination (Figures S5B and S6A). Similar to VACV-N2L-ΔNLS, the ECTV-EVM020-ΔNLS induced higher levels of IFNB1 and ISGs (Figure S6B), and exhibited weaker replicative capacity than ECTV-WT (Figure S6C and S6E). NLS deletion no longer affected virus replication in Vero-E6 cells (Figure S6D). In addition, overexpression of EVM020 rescued the attenuation of ECTV-EVM020-ΔNLS (Figure S6F). These data demonstrate that NLS of P2 orthologues enables OPXVs to escape innate immunity and induce higher virus titers. We further analyzed the genomes of attenuated VACV vaccine strains and their respective deleted genes (Figure S7). The results show that P2L orthologue truncation or loss occurred in Tian Tan vaccine strain (VACV-VTT), VACV Ankara (VACV-MVA), VACV New York Vaccinia Virus strain (VACV-NYVAC), and JYNNEOS, suggesting that P2L orthologue may be one of the key virulence factors in OPXV.
Figure 7. Deletion of NLS from the P2 orthologue (N2) in VACV-WR attenuates viral replication and virulence. (A) The NLS deletion strategy of the N2L gene in VACV-WR: the N2L gene in the VACV-WR genome was replaced with EGFP-N2L-ΔNLS via homologous recombination. (B) The mRNA levels of IFNB1, ISG15, ISG20, MX1, MX2, and IFIT1 were determined by RT-qPCR in HeLa cells infected with VACV-WT or VACV-N2L-ΔNLS (MOI = 0.1) for 12 h. (C) Virus titers were determined in HeLa cells infected with VACV-WT or VACV-N2L-ΔNLS (MOI = 0.1) for the indicated times by plaque assay. (D) Virus titers were determined in Vero-E6 cells infected with VACV-WT or VACV-N2L-ΔNLS (MOI = 0.1) for the indicated times by plaque assay. (E) Immunoblotting analysis of VACV-WR-A35 in HeLa cells infected with VACV-WT or VACV-N2L-ΔNLS (MOI = 0.1) for 24 or 48 h (left). Band intensities were determined by ImageJ software (right). (F) Virus titers were determined in HeLa cells transfected with Myc-N2 or Myc-vector plasmid for 24 h, followed by infection with VACV-WT (MOI = 0.1) or VACV-N2L-ΔNLS for the indicated times by plaque assay. (G) Schematic illustration of the experiment to measure the virulence of VACV-WT and VACV-N2L-ΔNLS in mice. Briefly, groups of mice were infected with VACV-WT or VACV-N2L-ΔNLS (5 × 106 PFU) by intraperitoneal infection, and the body weight changes and clinical observations of the mice were monitored for 20 days (n = 10). On the d 6 post infection, mice were euthanized and the lungs, spleen, and liver were collected for further detection (n = 5). (H, I) Body weight changes (h) and survival rate (i) of mice after virus infection. (J) The mRNA levels of Ifnb1, Isg15, Isg20, Mx1, Mx2, and Ifit1 in lungs of virus-infected mice were detected by RT-qPCR at d 6 post infection. GAPDH expression was used as a normalizer. (K) RT-qPCR analysis of VACV A35R mRNA expression in lungs, spleens, and livers of virus-infected mice. GAPDH expression was used as a normalizer. (L) Infectious viral titers in lungs, spleens, and livers of virus-infected mice were determined by plaque assay. (M) Quantitative analysis of the number of VACV-WR-positive cells in spleen of virus-infected mice by immunohistochemistry staining with anti-A35 antibody. Scale bar: 30 µm. (N) H&E staining image of mouse lungs of virus-infected mice. The severity of pathological manifestations was scored from 0 to 4 with 0 for no damage and four for the maximum damage. Scale bar: 30 µm. Data are shown as mean ± SD. ***P < 0.001; Multiple t-tests for (e, k, and l), two-tailed Student’s t-test for (m and n), one-way repeated measurement analysis of variance (RMANOVA) for (c, f, and h), and log rank test for (i).
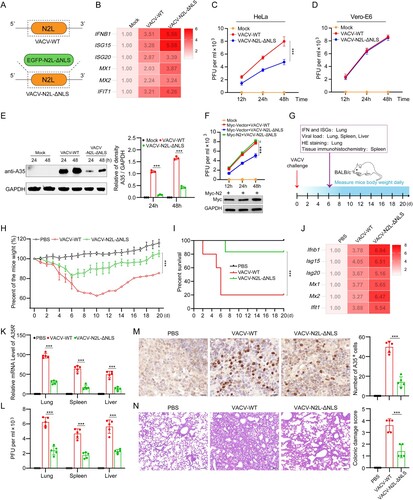
Discussion
The adaptive evolution of OPXV is accompanied by nucleotide insertions, deletions, and/or mutations in genomic DNA [Citation45-47]. MPXV P2 is a highly conserved Bcl-2-like family protein, and functional orthologues are present in the majority of viruses of the genus OPXV. In this study, we found that the NLS near the C terminus of P2 orthologue is lost in TATV-031, CMLV-027, and vaccine strain VACV-VTT-TN2. Therefore, these proteins could not localize to the nucleus and suppress IRF3-dependent gene expression, like other P2 orthologues with the NLS. Mechanistically, the NLS of MPXV P2 interacted with KPNA2 to facilitate P2 nuclear translocation, which competitively inhibited KPNA2-mediated IRF3 nuclear translocation and downstream IRF3-dependent gene expression (). These findings reveal an efficient innate immune evasion strategy of OPXV, indicating that the NLS of MPXV P2 orthologue is critical to hijack innate immunity and thus facilitate virus replication.
Figure 8. The NLS of MPXV P2 orthologue hijacks KPNA2 to inhibit the nuclear import of IRF3. Upon OPXV infection, the cGAS-STING signalling pathway is activated. The NLS of P2 or homologous protein (such as from VACV, VARV, MPXV and ECTV) interacts with KPNA2, which inhibits KPNA2-mediated IRF3 nuclear import, leading to reduced transcription of IRF3-dependent genes. However, the P2 proteins lacking an NLS (such as from VACV-VTT, TATV, and CMLV) do not block the nuclear localization of IRF3.
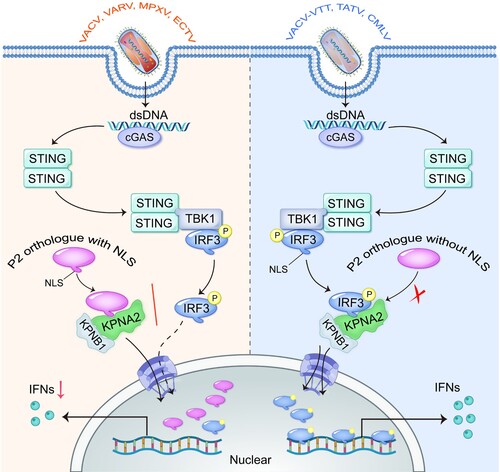
We found that the P2 orthologues of OPXVs (except TATV-031 and CMLV-027) were able to localize to the nucleus and inhibit IRF3-mediated gene expression. Consistent with our results, Ferguson, et. al. demonstrated that the P2 orthologue VACV N2 is located in the nucleus and inhibits IFN production [Citation27]. Another line of research indicated that VACV N2 could interact with KPNA2 by yeast two-hybrid assays, but this was not investigated further and the biological significance of this interaction was untested [Citation48]. In this study, we discovered that P2 interacted directly with KPNA2 to inhibit KPNA2-mediated IRF3 nuclear translocation and the downstream gene expression. In addition, KPNA2 is able to recognize the NLS of other viral proteins and cellular factors, thus regulating their movement. For example, the open reading frame 6 (ORF6) of the SARS-CoV-2 inhibits IFN-β production by binding to KPNA2 to block IRF3 nuclear translocation [Citation32]. KPNA2 mediates the nuclear import of Nijmegen breakage syndrome protein 1 [Citation49], mediator of DNA damage checkpoint protein 1 [Citation50], and RAS-related C3 botulinum toxin substrate 1 [Citation51], which is not related to IRF3-mediated gene expression.
Despite evidence that OPXV emerged thousands of years ago, the origin and evolution of OPXV are still blurred [Citation45,Citation52,Citation53]. The poxvirus genome has evolved through the acquisition and loss of genes, particularly gene duplication and horizontal gene transfer, in order to adapt to and counter the host's antiviral immune defences and thus establish successful infection [Citation54]. Analysis of the content and organization of the OPXV genome suggests that the acquisition and loss of genes is the primary mechanism of its evolution [Citation55,Citation56]. The host range of poxvirus is not limited by species-specific receptors, and the success of infection primarily relies on its ability to counteract the host immune response [Citation57]. High virulence typically occurs when viruses spread to other animal species, as observed in the current 2019 coronavirus disease (COVID-19) pandemic [Citation58]. In this study, we found that the P2 orthologue has partial or complete loss of NLS in TATV and CMLV compared to other OPXVs, rendering them unable to localize to the nucleus and inhibit the host's innate immunity, which might influence OPXV host range. However, we have not investigated whether add back the NLS to P2 orthologues in TATV and CMLV could restore the IRF3 inhibition, or whether deletion of the NLS from P2 in MPXV could inhibit IRF3 nuclear translocation and attenuated viral replication and virulence. These are limitations of this study.
In summary, NLS of OPXV P2 orthologue hijacks KPNA2 to prevent IRF3 translocation and facilitate virus replication. Although it is unclear whether P2 orthologue plays a dominant role in OPXV replication, our data indicate that deletion of P2 orthologue or its NLS might be a potential way to develop the attenuated OPXV vaccines. Screening or designing the small molecule inhibitors targeting P2 orthologue or its nuclear import (such as ivermectin) is also a promising strategy to treat OPXV infection. In addition, P2 orthologue might promote viral replication through other mechanisms after it enters the nucleus, for example inhibiting host mRNA nuclear export and the function of host nuclear proteins, which is interesting and worthy of further study.
Author contributions
L.S. initiated and supervised the project. L.S. and P.J. designed the experiments, analyzed the data, and wrote the first draft of manuscript the paper. P.J., J.M., and Y.Z. performed the experiments. H.Z., X.J., W.F., X.B., Y.Z., Y.L., H.Z., J.G., G.P., K.Z., M.F., and M.L. helped with some experiments. W.L., X.J., and G.S. analyzed the data and revised the manuscript.
Supplemental Material
Download MS Word (1.6 MB)Supplemental Material
Download MS Excel (13 KB)Disclosure statement
No potential conflict of interest was reported by the author(s).
Additional information
Funding
References
- Moss B, Smith GL. (2021). Poxviridae: the viruses and their replication. Fields virology DNA viruses, chapter 16.
- Fenner FHD, Arita I, Jezek Z, et al. Smallpox and its eradication. Geneva: World Health Organization; 1988. p. 1460.
- Roper RL, Garzino-Demo A, Del Rio C, et al. Monkeypox (Mpox) requires continued surveillance, vaccines, therapeutics and mitigating strategies. Vaccine. 2023 May 11;41(20):3171–3177. doi:10.1016/j.vaccine.2023.04.010
- Isidro J, Borges V, Pinto M, et al. Phylogenomic characterization and signs of microevolution in the 2022 multi-country outbreak of monkeypox virus. Nat Med. 2022 Aug;28(8):1569–1572. doi:10.1038/s41591-022-01907-y
- Ndodo N, Ashcroft J, Lewandowski K, et al. Distinct monkeypox virus lineages co-circulating in humans before 2022. Nat Med. 2023 Sep;29(9):2317–2324. doi:10.1038/s41591-023-02456-8
- Todd G, Smith CMG, Wynn NT, et al. Resistance to anti-orthopoxviral drug tecovirimat (TPOXX®) during the 2022 mpox outbreak in the US. Preprint at medRxiv. 2023.
- Smith GL, Talbot-Cooper C, Lu Y. How does vaccinia virus interfere with interferon? Adv Virus Res. 2018;100:355–378. doi:10.1016/bs.aivir.2018.01.003
- González JM, Esteban M. A poxvirus Bcl-2-like gene family involved in regulation of host immune response: sequence similarity and evolutionary history. Virol J. 2010 Mar 15;7:59. doi:10.1186/1743-422X-7-59
- Bowie A, Kiss-Toth E, Symons JA, et al. A46R and A52R from vaccinia virus are antagonists of host IL-1 and toll-like receptor signaling. Proc Natl Acad Sci USA. 2000 Aug 29;97(18):10162–7. doi:10.1073/pnas.160027697
- Stack J, Haga IR, Schröder M, et al. Vaccinia virus protein A46R targets multiple Toll-like-interleukin-1 receptor adaptors and contributes to virulence. J Exp Med. 2005 Mar 21;201(6):1007–1018. doi:10.1084/jem.20041442
- Harte MT, Haga IR, Maloney G, et al. The poxvirus protein A52R targets Toll-like receptor signaling complexes to suppress host defense. J Exp Med. 2003 Feb 3;197(3):343–351. doi:10.1084/jem.20021652
- Mansur DS, Maluquer de Motes C, Unterholzner L, et al. Poxvirus targeting of E3 ligase β-TrCP by molecular mimicry: a mechanism to inhibit NF-κB activation and promote immune evasion and virulence. PLoS Pathog. 2013 Feb;9(2):e1003183. doi:10.1371/journal.ppat.1003183
- Neidel S, Ren H, Torres AA, et al. NF-κB activation is a turn on for vaccinia virus phosphoprotein A49 to turn off NF-κB activation. Proc Natl Acad Sci USA. 2019 Mar 19;116(12):5699–5704. doi:10.1073/pnas.1813504116
- Neidel S, Maluquer de Motes C, Mansur DS, et al. Vaccinia virus protein A49 is an unexpected member of the B-cell Lymphoma (Bcl)-2 protein family. J Biol Chem. 2015 Mar 6;290(10):5991–6002. doi:10.1074/jbc.M114.624650
- Chen RA, Ryzhakov G, Cooray S, et al. Inhibition of IkappaB kinase by vaccinia virus virulence factor B14. PLoS Pathog. 2008 Feb 8;4(2):e22. doi:10.1371/journal.ppat.0040022
- Postigo A, Way M. The vaccinia virus-encoded Bcl-2 homologues do not act as direct Bax inhibitors. J Virol. 2012 Jan;86(1):203–213. doi:10.1128/JVI.05817-11
- Boys IN, Johnson AG, Quinlan M, et al. Structural homology screens reveal poxvirus-encoded proteins impacting inflammasome-mediated defenses. bioRxiv: the preprint server for biology. 2023 Apr 3.
- Unterholzner L, Sumner RP, Baran M, et al. Vaccinia virus protein C6 is a virulence factor that binds TBK-1 adaptor proteins and inhibits activation of IRF3 and IRF7. PLoS Pathog. 2011 Sep;7(9):e1002247. doi:10.1371/journal.ppat.1002247
- Lu Y, Zhao Y, Gao C, et al. HDAC5 is a positive regulator of IRF3 activation and is targeted for degradation by protein C6 from orthopoxviruses including monkeypox virus and variola virus. Cell Rep. 2024 Mar 26;43(3):113788.
- Stuart JH, Sumner RP, Lu Y, et al. Vaccinia virus protein C6 inhibits type I IFN signalling in the nucleus and binds to the transactivation domain of STAT2. PLoS Pathog. 2016 Dec;12(12):e1005955. doi:10.1371/journal.ppat.1005955
- Lu Y, Stuart JH, Talbot-Cooper C, et al. Histone deacetylase 4 promotes type I interferon signaling, restricts DNA viruses, and is degraded via vaccinia virus protein C6. Proc Natl Acad Sci USA. 2019 Jun 11;116(24):11997–12006. doi:10.1073/pnas.1816399116
- Peng C, Wyatt LS, Sg G-S, et al. Zinc-finger antiviral protein (ZAP) is a restriction factor for replication of modified vaccinia virus Ankara (MVA) in human cells. PLoS Pathog. 2020 Aug;16(8):e1008845. doi:10.1371/journal.ppat.1008845
- Zhai D, Yu E, Jin C, et al. Vaccinia virus protein F1L is a caspase-9 inhibitor. J Biol Chem. 2010 Feb 19;285(8):5569–5580. doi:10.1074/jbc.M109.078113
- Maluquer de Motes C, Cooray S, Ren H, et al. Inhibition of apoptosis and NF-κB activation by vaccinia protein N1 occur via distinct binding surfaces and make different contributions to virulence. PLoS Pathog. 2011 Dec;7(12):e1002430. doi:10.1371/journal.ppat.1002430
- Schröder M, Baran M, Bowie AG. Viral targeting of DEAD box protein 3 reveals its role in TBK1/IKKepsilon-mediated IRF activation. EMBO J. 2008 Aug 6;27(15):2147–2157. doi:10.1038/emboj.2008.143
- Torres AA, Macilwee SL, Rashid A, et al. The actin nucleator Spir-1 is a virus restriction factor that promotes innate immune signalling. PLoS Pathog. 2022 Feb;18(2):e1010277.
- Ferguson BJ, Benfield CTO, Ren H, et al. Vaccinia virus protein N2 is a nuclear IRF3 inhibitor that promotes virulence. J Gen Virol. 2013 Sep;94(Pt 9):2070–2081. doi:10.1099/vir.0.054114-0
- Fang M, Sigal LJ. Antibodies and CD8+ T cells are complementary and essential for natural resistance to a highly lethal cytopathic virus. J Immunol (Baltimore, MD: 1950). 2005 Nov 15;175(10):6829–6836. doi:10.4049/jimmunol.175.10.6829
- Li Y, Sheng Y, Chu Y, et al. Seven major genomic deletions of vaccinia virus Tiantan strain are sufficient to decrease pathogenicity. Antiviral Res. 2016 May;129:1–12. doi:10.1016/j.antiviral.2016.01.013
- Jiao P, Fan W, Ma X, et al. SARS-CoV-2 nonstructural protein 6 triggers endoplasmic reticulum stress-induced autophagy to degrade STING1. Autophagy. 2023 Dec;19(12):3113–3131. doi:10.1080/15548627.2023.2238579
- Lu Y, Michel HA, Wang PH, et al. Manipulation of innate immune signaling pathways by SARS-CoV-2 non-structural proteins. Front Microbiol. 2022;13:1027015. doi:10.3389/fmicb.2022.1027015
- Zheng Y, Zhuang MW, Han L, et al. Severe acute respiratory syndrome coronavirus 2 (SARS-CoV-2) membrane (M) protein inhibits type I and III interferon production by targeting RIG-I/MDA-5 signaling. Signal Transduct Target Ther. 2020 Dec 28;5(1):299. doi:10.1038/s41392-020-00438-7
- Rolhion N, Furniss RC, Grabe G, et al. Inhibition of nuclear transport of NF-ĸB p65 by the Salmonella type III secretion system effector SpvD. PLoS Pathog. 2016 May;12(5):e1005653. doi:10.1371/journal.ppat.1005653
- Fagerlund R, Kinnunen L, Köhler M, et al. NF-{kappa}B is transported into the nucleus by importin {alpha}3 and importin {alpha}4. J Biol Chem. 2005 Apr 22;280(16):15942–15951. doi:10.1074/jbc.M500814200
- Fagerlund R, Melén K, Cao X, et al. NF-kappaB p52, RelB and c-Rel are transported into the nucleus via a subset of importin alpha molecules. Cell Signal. 2008 Aug;20(8):1442–1451. doi:10.1016/j.cellsig.2008.03.012
- Wang C, Sun M, Yuan X, et al. Enterovirus 71 suppresses interferon responses by blocking Janus kinase (JAK)/signal transducer and activator of transcription (STAT) signaling through inducing karyopherin-α1 degradation. J Biol Chem. 2017 Jun 16;292(24):10262–10274. doi:10.1074/jbc.M116.745729
- Wang R, Nan Y, Yu Y, et al. Porcine reproductive and respiratory syndrome virus Nsp1β inhibits interferon-activated JAK/STAT signal transduction by inducing karyopherin-α1 degradation. J Virol. 2013 May;87(9):5219–5228. doi:10.1128/JVI.02643-12
- Cautain B, Hill R, de Pedro N, et al. Components and regulation of nuclear transport processes. FEBS J. 2015 Feb;282(3):445–462. doi:10.1111/febs.13163
- Yang Y, Xue J, Teng Q, et al. Mechanisms and consequences of Newcastle disease virus W protein subcellular localization in the nucleus or mitochondria. J Virol. 2021 Mar 10;95(7):e02087-20.
- Cai Z, Zhang MX, Tang Z, et al. USP22 promotes IRF3 nuclear translocation and antiviral responses by deubiquitinating the importin protein KPNA2. J Exp Med. 2020 May 4;217(5):e20191174.
- Wagstaff KM, Sivakumaran H, Heaton SM, et al. Ivermectin is a specific inhibitor of importin α/β-mediated nuclear import able to inhibit replication of HIV-1 and dengue virus. Biochem J. 2012 May 1;443(3):851–856. doi:10.1042/BJ20120150
- Ghildyal R, Ho A, Wagstaff KM, et al. Nuclear import of the respiratory syncytial virus matrix protein is mediated by importin beta1 independent of importin alpha. Biochemistry. 2005 Sep 27;44(38):12887–12895. doi:10.1021/bi050701e
- Truant R, Cullen BR. The arginine-rich domains present in human immunodeficiency virus type 1 Tat and Rev function as direct importin beta-dependent nuclear localization signals. Mol Cell Biol. 1999 Feb;19(2):1210–1217. doi:10.1128/MCB.19.2.1210
- Soderholm JF, Bird SL, Kalab P, et al. Importazole, a small molecule inhibitor of the transport receptor importin-β. ACS Chem Biol. 2011 Jul 15;6(7):700–708. doi:10.1021/cb2000296
- Brennan G, Stoian AMM, Yu H, et al. Molecular mechanisms of poxvirus evolution. mBio. 2023 Feb 28;14(1):e0152622. doi:10.1128/mbio.01526-22
- Hendrickson RC, Wang C, Hatcher EL, et al. Orthopoxvirus genome evolution: the role of gene loss. Viruses. 2010 Sep;2(9):1933–1967. doi:10.3390/v2091933
- McLysaght A, Baldi PF, Gaut BS. Extensive gene gain associated with adaptive evolution of poxviruses. Proc Natl Acad Sci USA. 2003 Dec 23;100(26):15655–15660. doi:10.1073/pnas.2136653100
- Zhang L, Villa NY, Rahman MM, et al. Analysis of vaccinia virus-host protein-protein interactions: validations of yeast two-hybrid screenings. J Proteome Res. 2009 Sep;8(9):4311–4318. doi:10.1021/pr900491n
- Tseng SF, Chang CY, Wu KJ, et al. Importin KPNA2 is required for proper nuclear localization and multiple functions of NBS1. J Biol Chem. 2005 Nov 25;280(47):39594–39600. doi:10.1074/jbc.M508425200
- Radhakrishnan K, Park SJ, Kim SW, et al. Karyopherin α-2 Mediates MDC1 nuclear import through a functional nuclear localization signal in the tBRCT domain of MDC1. Int J Mol Sci. 2020 Apr 10;21(7):2650. doi:10.3390/ijms21072650
- Kim EG, Shin EY. Nuclear Rac1 regulates the bFGF-induced neurite outgrowth in PC12 cells. BMB Rep. 2013 Dec;46(12):617–622.
- Babkin IV, Babkina IN. The origin of the variola virus. Viruses. 2015 Mar 10;7(3):1100–1112. doi:10.3390/v7031100
- Li Y, Carroll DS, Gardner SN, et al. On the origin of smallpox: correlating variola phylogenics with historical smallpox records. Proc Natl Acad Sci USA. 2007 Oct 2;104(40):15787–15792. doi:10.1073/pnas.0609268104
- Cui H, Guan J, Lu H, et al. Rapid onsite visual detection of Orf virus using a recombinase-aided amplification assay. Life (Basel, Switzerland). 2023 Feb 10;13(2):494.
- Hughes AL, Friedman R. Poxvirus genome evolution by gene gain and loss. Mol Phylogenet Evol. 2005 Apr;35(1):186–195. doi:10.1016/j.ympev.2004.12.008
- Esteban DJ, Hutchinson AP. Genes in the terminal regions of orthopoxvirus genomes experience adaptive molecular evolution. BMC Genom. 2011 May 23;12:261.
- Yu H, Bruneau RC, Brennan G, et al. Battle Royale: innate recognition of poxviruses and viral immune evasion. Biomedicines. 2021 Jul 1;9(7):765.
- Alcamí A. Was smallpox a widespread mild disease? Science (New York, NY). 2020 Jul 24;369(6502):376–377.