Disclaimer
As a service to authors and researchers we are providing this version of an accepted manuscript (AM). Copyediting, typesetting, and review of the resulting proofs will be undertaken on this manuscript before final publication of the Version of Record (VoR). During production and pre-press, errors may be discovered which could affect the content, and all legal disclaimers that apply to the journal relate to these versions also.Orthopoxviruses, such as variola virus (VARV), monkeypox virus (MPXV), ectromelia virus (ECTV), cowpox virus (CPXV), and vaccinia virus (VACV), pose severe threats to human, domestic, and wildlife health 1. Smallpox caused over 300 million deaths in the 20th century despite vaccination efforts 2. Since May 2022, MPXV has rapidly spread beyond Africa to 117 countries spanning all six WHO regions 3. From January 2022 to May 2024, there were 97,208 laboratory-confirmed cases, resulting in 186 deaths 4. Human-to-human transmission occurs primarily through close contact with respiratory droplets or direct contact with lesions, body fluids and contaminants, and may also occur vertically through the placenta 5. Treatment options for monkeypox virus infections are limited to tecovirimat and brincidofovir. Tecovirimat (ST-246) was approved for smallpox treatment in July 2018 6,7 but encountered resistance in a patient with progressive vaccinia 8. Brincidifovir, previously CMX001, received orphan drug designation in 2018 9 and was approved for smallpox treatment in June 2021. However, it led to increased liver enzyme activity in patients with MPXV infection in 2018 and 2021 10,11. Thus, novel antiviral compounds are urgently needed. VP37, as a viral structural protein of MPXV, plays an important role in the envelopment of the intracellular mature virus with Golgi-derived membrane to form enveloped virus 12. VP37 is highly conserved among orthopoxviruses (Supplementary Figure 1). It lacks homologous proteins in human and other mammalian cells with the highest structural similarity to VP37 is phospholipase D of Staphylococcus aureus 13, indicating that VP37 from orthopoxviruses is a promising and practical target for antiviral therapy. Here, we aimed to identify such novel compounds by screening against MPXV VP37 protein using an in-house compound library and in vitro experiments.
To pinpoint inhibitors targeting VP37, molecular docking was performed for >6,000 compounds, focusing on the predicted pocket of VP37 (A). The 3D structure of VP37 was colour-coded based on the per-residue accuracy of the structure (pLDDT) score, with an average predicted confidence score of 91.94% (A). Among these compounds, 45 showed superior docking scores (< -9.0 kcal/mol) compared to those of ST-246 (-8.2 kcal/mol) (Supplemental Table 1). These compounds were subjected to further in vitro testing.
Figure 1. In Vitro Antiviral Effect of Three Compounds against Orthopoxviruses. (A) Schematic of the experimental procedure. (B) Preliminary screening of compounds with antiviral activity at 20 or 2 μM concentration based on Vero cells infected with VACV-Fluc. X-axis = number of compounds, y-axis = inhibition rate. (C) EC50 values of the five compounds against VACV-Fluc determined using luciferase reporter assay. (D) EC50 values of the five compounds against VACV-Fluc determined using real-time PCR and plaque assay. (E) EC50 values of the five compounds against ECTV determined using real-time PCR and plaque assay. (F) EC50 values of the five compounds against MPXV determined using real-time PCR and plaque assay, respectively. (D, E, F) Blue lines represent dose response curve of real-time PCR, and red lines represent dose response curves of plaque assay. (G) Molecular docking of gs23 or DRN804 with MPXV VP37 protein. Residues of VP37 are shown as stick in gray, while gs23 is shown as sticks in magenta. Residues of VP37 are shown as stick in gray. Rupatadine is shown as sticks in blue. (H) Surface plasmon resonance analysis of the binding of gs23, DRN7 and DRN804 to monkeypox virus VP37 protein.
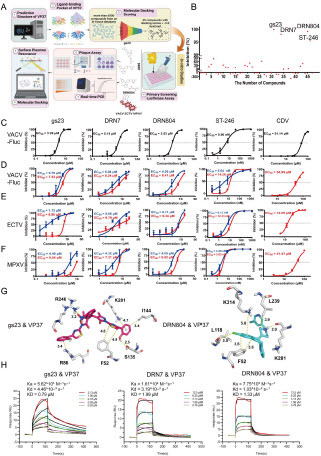
Luciferase Reporter Assay screening was conducted to evaluate the in vitro anti-vaccinia virus activity of these 45 compounds. A concentration of 20 μM was selected if cell viability exceeded 50%; otherwise, 2 μM was used (B). Three compounds—gs23 (CAS No. 307339-09-9), DRN7(CAS No. 158876-82-5, rupatadine), and DRN804 (CAS No. 182349-12-8, rupatadine fumarate)—showed >90% inhibitory activity against VACV-Fluc at a 20 μM concentration (C). ST-246 and Cidofovir served as the positive controls, with ST-246 showing nanomolar level inhibition. The anti-vaccinia virus activity of these three compounds was further assessed using real-time PCR and classical plaque assay. (D). Both methods confirmed the effectiveness of the 3 compounds against vaccinia virus in vitro (Supplemental Table 2).
Ectromelia virus (ECTV), causing mousepox, is a valuable model for studying the pathogenesis of poxvirus, which is closely related to variola virus, the cause of smallpox. As anticipated, three compounds inhibited ECTV infections (E, Supplemental Table 2). This suggests that all three compounds inhibit ECTV plaque formation and viral replication in vitro.
Monkeypox virus evolves, with the B.1 lineage being closely linked to the current human monkeypox outbreak 3,14. Hence, we investigated whether these compounds, with anti-VACV and anti-ECTV activities, could also inhibit MPXV B.1 infection. As expected, all three compounds inhibited monkeypox virus infection (F, Supplemental Table 2). These results indicate that all three compounds possess anti-panorthopoxvirus activity in vitro.
Molecular docking revealed that the oxygen atom on the nitro group of gs23 formed an electrostatic interaction with R246. Additionally, R86 formed a cation-π interaction with the phenyl moiety of gs23, and the thiazole moiety of gs23 formed a π-π interaction with F52. Another phenyl moiety of gs23 formed a cation-π interaction with K281 and a hydrophobic interaction with I144. Furthermore, the hydroxyl group of gs23 formed a hydrogen bond with S135 (G). Both rupatadine and rupatadine fumarate showed similar binding modes when interacting with VP37, as rupatadine fumarate dissociates into rupatadine in solution. The key residues for the binding of rupatadine fumarate to VP37 were identified as F52, L118, L239, K281, and K314 using docking results. The phenyl moiety of rupatadine fumarate formed π-π and cation-π interactions with F52 and K314, respectively. The piperidine moiety of rupatadine fumarate was predicted to interact hydrophobically with L239 and form a hydrogen bond with K281. Additionally, the chlorine atom of rupatadine fumarate formed a halogen bond with L118 (G). All the predicted interactions sites were conserved among the orthopoxviruses (Supplementary Figure 1).
To validate the target of three compounds, the affinity of three compounds to monkeypox virus VP37 were analyzed via surface plasmon resonance. Gs23 can bind monkeypox virus VP37 (H), with a dissociation constant (KD) of 0.79 µM. For fumarate, the KD was 1.99 µM. For rupatadine fumarate, the KD was 1.33 µM. However, ST-246 failed to bind VP37 (Data not shown), which suggests differences in its anti-panorthopoxvirus mechanism compared to that of the three compounds.
Further investigation is needed to fully evaluate the safety, dosage, and efficacy of rupatadine fumarate and rupatadine in animal models to establish their in vivo efficacy. The promising results of this study suggest that gs23, rupatadine fumarate, and rupatadine are valuable additions to antiviral therapies against monkeypox and other infections caused by orthopoxviruses. VP37 of orthopoxviruses could serve as a potential target for drug design.
Declaration of interest statement
The authors declare that they have no known competing financial interests
Acknowledgements
This work was supported by the National Key Research and Development Program of China (2022YFC2304100, 2023YFD1800405, 2022YFC2303401) and the National Natural Science Foundation of China (82322067). The funders had no role in study design, data collection and analysis, decision to publish, or preparation of the manuscript.
References
- Shchelkunova GA, Shchelkunov SN. Smallpox, Monkeypox and Other Human Orthopoxvirus Infections. Viruses. 2022;15(1). doi: 10.3390/v15010103
- Shchelkunov SN. Emergence and reemergence of smallpox: The need for development of a new generation smallpox vaccine. Vaccine. 2011;29:D49-D53. https://doi.org/10.1016/j.vaccine.2011.05.037
- Lum FM, Torres-Ruesta A, Tay MZ, et al. Monkeypox: disease epidemiology, host immunity and clinical interventions. Nat Rev Immunol. 2022;22(10):597-613. doi: 10.1038/s41577-022-00775-4
- https://worldhealthorg.shinyapps.io/mpx global/.
- Saied AA, Dhawan M, Metwally AA, Fahrni ML, Choudhary P, Choudhary OP. Disease History, Pathogenesis, Diagnostics, and Therapeutics for Human Monkeypox Disease: A Comprehensive Review. Vaccines. 2022;10(12). doi: 10.3390/vaccines10122091
- Hoy SM. Tecovirimat: First Global Approval. Drugs. 2018;78(13):1377-1382. doi: 10.1007/s40265-018-0967-6
- Delaune D, Iseni F. Drug Development against Smallpox: Present and Future. Antimicrob Agents Chemother. 2020;64(4). doi: 10.1128/AAC.01683-19
- Lederman ER, Davidson W, Groff HL, et al. Progressive vaccinia: case description and laboratory-guided therapy with vaccinia immune globulin, ST-246, and CMX001. J Infect Dis. 2012;206(9):1372-1385. doi: 10.1093/infdis/jis510
- Hung YP, Lee CC, Lee JC, Chiu CW, Hsueh PR, Ko WC. A brief on new waves of monkeypox and vaccines and antiviral drugs for monkeypox. J Microbiol Immunol Infect. 2022;55(5):795-802. doi: 10.1016/j.jmii.2022.08.016
- Adler H, Gould S, Hine P, et al. Clinical features and management of human monkeypox: a retrospective observational study in the UK. Lancet Infect Dis. 2022;22(8):1153-1162. doi: 10.1016/S1473-3099(22)00228-6
- Vaughan A, Aarons E, Astbury J, et al. Two cases of monkeypox imported to the United Kingdom, September 2018. Euro Surveill Bull Eur sur les Mal Transm = Eur Commun Dis Bull. 2018;23(38). doi: 10.2807/1560-7917.ES.2018.23.38.1800509
- Rizk JG, Lippi G, Henry BM, Forthal DN, Rizk Y. Prevention and Treatment of Monkeypox. Drugs. 2022;82(9):957-963. doi: 10.1007/s40265-022-01742-y
- Höer A, Schöneberg T, Harteneck C, Cetindag C, Oberdisse E. Enhancement of phospholipase D activity following baculovirus and adenovirus infection in Sf9 and COS-7 cells. Biochim Biophys Acta. 1998;1393(2-3):325-335. doi: 10.1016/s0005-2760(98)00087-3
- Li H, Zhang H, Ding K, et al. The evolving epidemiology of monkeypox virus. Cytokine Growth Factor Rev. 2022;68:1-12. doi: 10.1016/j.cytogfr.2022.10.002