Abstract
Hierarchical micro-mesoporous materials with the properties of Beta zeolite and mesoporous cellular foam (MCF) material were prepared by impregnation of MCF with a suspension of zeolite nanoparticles. Four different methods were used for MCF modification: (1) wet impregnation, (2) wet impregnation with acidification of Beta nanoseeds suspension, (3) incipient wetness impregnation, (4) incipient wetness impregnation with acidification of Beta nanoseeds suspension. The obtained materials were characterized using nitrogen sorption measurements, thermogravimetric analysis (TGA), X-ray diffraction (XRD), and diffuse reflectance infrared Fourier transform (DRIFT) techniques. The use of different impregnation methods resulted in introduction of various forms of iron species to the samples. The iron modified samples (iron was introduced using ion-exchange method) as well as mesoporous MCF silica and Beta zeolite (as reference samples) were tested as catalysts in the N2O decomposition reaction.
1. Introduction
One of the new trends in designing of advanced materials for catalysis and adsorption is related to the synthesis of materials with hierarchical porous structure. An example could be a combination of microporous, crystalline zeolite characterized by acidic and ion-exchange properties and high surface area mesoporous silica. It is expected that such hybrid composite materials, which show properties of both mesoporous silica materials and zeolites will be active, selective and stable catalysts of many reactions [Citation1–Citation3].
The origin of mesoporosity in zeolites can be fundamentally different, what greatly extends the areas of the synthesis methods. Ĉejka et al. [Citation4] and Egebald et al. [Citation5] proposed the following routes to obtain materials with hierarchical pore sizes distribution: (1) recrystallization of amorphous mesoporous materials, (2) supramolecular templating, (3) mesoporous framework build from the zeolite nanoseeds, (4) deposition of zeolite seeds onto mesoporous materials, (5) desilication and dealumination, (6) solid templating, (7) nontemplating method and (8) delamination of 2D zeolites. These methods can be matched and mixed together in many different combinations opening new possibilities in this field of science.
Presented studies were focused on preparation of hierarchical micro-mesoporous materials, which integrate the properties of Beta zeolite and mesopous MCF silica. These materials were obtained by impregnation of Beta zeolite nanoseeds on the MCF surface using four different impregnation methods. The obtained materials were modified with iron by wet ion-exchange method and tested as catalysts in N2O decomposition reaction.
2. Experimental methods
2.1. Catalysts preparation
The Beta zeolite nanoparticles solution, prepared according to Van Oers et al. [Citation6], was hydrothermal treated at 423 K for 24 h. The resulting milk-like suspension was impregnated on MCF silica (synthesis recipe presented in [Citation7]) using different impregnation methods: (1) wet impregnation (βMCF-w), (2) wet impregnation with acidification of Beta nanoseeds suspension (βMCF-wa), (3) incipient wetness impregnation (βMCF-i), (4) incipient wetness impregnation with acidification of Beta nanoseeds suspension (βMCF-ia). In case of wet impregnation 15 mL of the nanoparticles suspension per 1 g of MCF was added. The acidification of the Beta nanoparticles slurry (5 mL of concentrated HCl per 18 mL of nanoseeds suspension) resulted in limitation of the zeolite crystals growth. The impregnated samples were dried in ambient conditions and calcined at 823 K for 6 h. Conventional β zeolite used as the reference sample was obtained by hydrothermal treatment of the Beta nanoparticles solution at 423 K for 7 days.
Iron was introduced to the samples by ion-exchange method (6 h at 358 K in anaerobic atmosphere), using 0.06 M solution of FeSO4·7H2O (Sigma-Aldrich). The Fe-modified samples were dried in ambient conditions and calcined at 823 K for 6 h.
2.2. Catalysts characterization
The X-ray diffraction (XRD) patterns of the samples were recorded using Bruker D2 Phaser instrument, in the 2 theta ranges of 5–60° with steps of 0.02°.
The infrared spectroscopy (IR) measurements were performed using Nicolet 6700 FT-IR spectrometer (Thermo Scientific) equipped with DRIFT (diffuse reflectance infrared Fourier transform) accessory. The measurements were carried out in the wavenumber range of 400–4000 cm−1 with a resolution of 2 cm−1, for the 4 wt.% of the samples grounded in dried KBr.
The low-temperature nitrogen adsorption–desorption measurements were done using an ASAP 2010 sorptomat, while UV-vis-DR (diffuse reflectance ultraviolet–visible spectroscopy) spectra of the samples were recorded using an Evolution 600 (Thermo) spectrophotometer. Details of these measurements were presented in our previous work [Citation8].
Catalytic studies of N2O decomposition were performed in a fixed-bed quartz microreactor. Composition of outlet gases was analyzed by a gas chromatograph (SRI 8610C) equipped with thermal conductivity detector (TCD). The experiments were performed using 0.1 g of the catalyst (particles sizes in the range of 0.160–0.315 mm) in the temperature range from 473 to 823 K in the intervals of 25 K. The reaction mixture with the following composition: 1000 ppm of N2O, 40,000 ppm of O2 and 200 ppm of NO (optionally) diluted in helium was used. Total flow rate of the reaction mixture was 50 ml/min.
3. Results and discussion
The nitrogen adsorption–desorption isotherms of mesoporous MCF and β zeolite are presented in Figure . The isotherm obtained for MCF is type IV (according to the IUPAC classification) and is characteristic of mesoporous materials (Figure ). The hysteresis loop at P/P 0 relative pressure of about 0.8–0.9 proves the presence of large and uniform mesopores. The type I isotherm, which was obtained for β zeolite, confirms its microporous structure (Figure ).
Figure 1 Nitrogen adsorption–desorption isotherms recorded at 77 K, for (a) MCF and (b) Beta zeolite
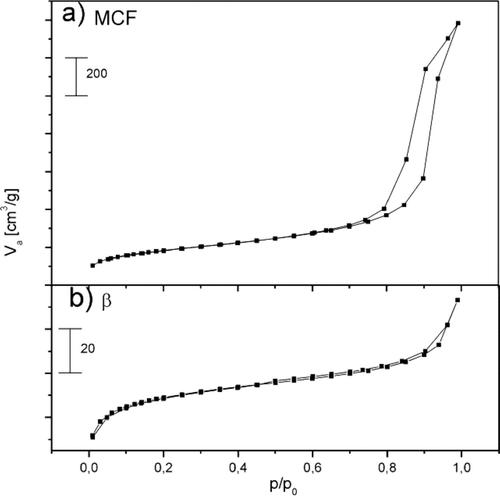
The textural parameters of MCF and β zeolite are presented in Table . The MCF mesoporous material is characterized by larger surface area as well as meso and macropore volume (634 m2/g and 2.069 cm3/g, respectively) in comparison to Beta zeolite, which possesses significantly higher micropore volume (0.235 cm3/g).
Table 1 Textural properties of the MCF and β samples determined from N2-sorption measurements at 77 K
Figure shows the X-ray powder diffraction patterns of β zeolite and the micro-mesoporous composites (βMCF-w, βMCF-wa, βMCF-i, βMCF-ia). The XRD patterns of the all-examined samples correspond to the pattern of Beta family topology, although in case of the impregnated samples a decrease in the samples crystallinity (decrease in the content of the crystalline phase) was observed. The reflections for the impregnated samples are shifted toward higher angles (decrease in d spacings) what could be explained by the shorter crystallization time and acid treatment. It is possible that acid treatment resulted in incomplete build-in of aluminum atoms into the zeolite framework or leaching of aluminum atoms form the zeolite structure (the average Si−O bond length is shorter than that of Al–O) [Citation9,10].
The intensity of the reflections in diffractograms of the samples obtained by incipient wetness impregnation (βMCF-i, βMCF-ia) is lower than that observed for the samples obtained by wet impregnation (βMCF-w, βMCF-wa). It could be explained by different amounts of deposited β zeolite nanoseeds.
In case of the acidified samples (βMCF-wa, βMCF-ia), three new reflections appeared. Probably they are connected with the structural changes in the zeolite seeds structure (stopping of the crystals growth, aluminum leaching) and also the formation of new phases (such as NaCl, Al(OH)3) during acid treatment of the samples cannot be excluded. This interesting phenomenon needs additional, more detailed studies.
The DRIFT spectra of β zeolite and micro-mesoporous composites (βMCF-w, βMCF-wa, βMCF-i, βMCF-ia) in the region of OH stretching vibrations (Figure ) show three bands at about 3745, 3637 and 3000–3500 cm−1 [Citation9–Citation12]. The small and sharp band at 3745 cm−1, which corresponds to terminal silanols (Si–OH), increased for the acidified samples. It can be clearly visible by comparison of the spectra recorded for the βMCF-i and βMCF-ia samples. It proves that the acidification stopped the crystals growth because the increase in intensity of this band is connected with a decrease of the zeolite crystal sizes. The intensity of the band at 3637 cm−1, assigned to Si–OH–Al groups in the zeolite framework, decreased for the impregnated samples in comparison to β zeolite. This difference is a result of smaller content of the zeolite phase (shorter crystallization time of Beta nanoseeds) in the impregnated MCF samples. There is also a difference in intensity of this band in the spectra of the acidified and nonacidified samples. Because Si–OH–Al groups are considered to be responsible for Brønsted acidity, therefore it could be expected that in the βMCF-wa and βMCF-ia samples is lower content of this type acidity than in the βMCF-w and βMCF-i samples. The broad band at 3000–3500 cm−1 present in the all spectra is attributed to internal H-bonded silanols.
The diffusive-reflectance UV-vis spectra of iron-exchanged and calcined Fe-β zeolite, micro-mesoporous composites (Fe-βMCF-w, Fe-βMCF-wa, Fe-βMCF-i, Fe-βMCF-ia) and Fe-MCF sample are shown in Figure . The spectrum of iron species absorbance depending on the nature and distribution of Fe3+ species can be divided into three regions: (1) λ < 300 nm, for isolated iron cations, (2) 300 < λ < 400 nm, for oligonuclear FexOy clusters and (3) λ > 400 nm, for Fe2O3 nanoparticles. In case of isolated Fe3+, two oxygen to metal CT bands are expected (t 1→t 2 and t 1→e transitions) in the ranges of 190–250 nm and 250–300 nm [Citation13,Citation14].
Figure 3 DR UV-vis spectra of iron exchanged β zeolite, micro-mesoporous composites and MCF material
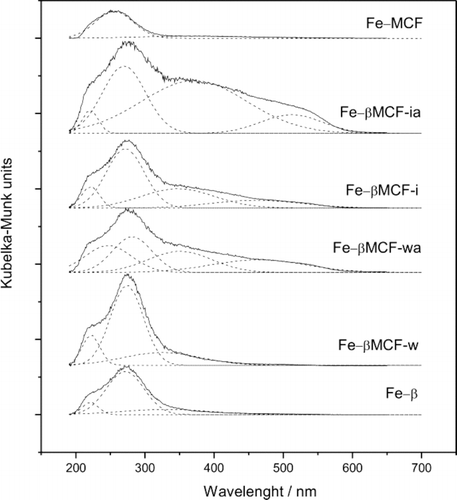
The Fe-β zeolite shows three absorption bands, which can be attributed to octahedrally coordinated Fe3+ ions (220 and 270 nm) and to oligonuclear FexOy species (350 nm). The same absorption bands were observed for the impregnated Fe-βMCF-w sample containing the highest amount of β nanoseeds among the impregnated samples. The intensities of absorption bands for this sample were significantly higher than for the Fe-β sample, what means that larger amount of iron was introduced to Fe-βMCF-w. It was probably caused by the smaller sizes of the zeolite crystals, what enhanced the accessibility of ion-exchange positions.
In the spectra of Fe-βMCF-wa, Fe-βMCF-i and Fe-βMCF-ia the new band characteristic of Fe2O3 clusters appeared. It could be explained by smaller content of the zeolitic phase in these samples, what resulted in accommodation of smaller amount of Fe3+ ions in the ion-exchange positions of zeolite and therefore, deposition of iron species also outside of the zeolite nanoseeds. Possibly these species were aggregated during calcinations with the formation of hematite particles. For the Fe-MCF sample only small absorption band, attributed to isolated octahedrally coordinated Fe3+ ions, was observed.
The temperature dependencies of N2O conversion over Fe-β zeolite, micro-mesoporous composites (Fe-βMCF-w, Fe-βMCF-wa, Fe-βMCF-i, Fe-βMCF-ia) and Fe-MCF sample are shown in Figure . All the hierarchical samples were found to be active catalysts for N2O decomposition. The used impregnation methods only slightly differentiated activity of the samples. For all the studied catalysts 95% of N2O conversion was obtained at about 823 K. The samples prepared using wet impregnation method (Fe-βMCF-w, Fe-βMCF-wa) presented slightly higher activity comparing to Fe-βMCF-i and Fe-βMCF-ia. It could be explained by higher content of zeolitic phase in the Fe-βMCF-w, Fe-βMCF-wa samples. The Fe-MCF sample was practically inactive in the studied temperature range, what shows a very important role of the zeolite phase in the process of N2O decomposition.
Figure 4 Temperature dependence of N2O conversion for (a) β zeolite, micro-mesoporous composites and MCF material; 1000 ppm N2O in He, total flow 50 ml/min, weight of catalyst 0.1 g. (b) Fe-βMCF-wa sample at different conditions; 1000 ppm N2O, 40,000 ppm O2, 200 ppm NO in He; total flow 50 ml/min; weight of catalyst 0.1 g
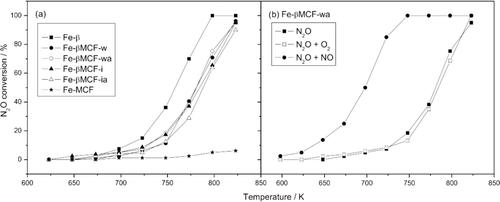
In the presence of the most active Fe-β zeolite sample, 100% of N2O conversion was reached at about 798 K, but it is also worth to mention that the activity of the micro-mesoporous composites is shifted only by about 50 K into higher temperatures in comparison to conventional Beta zeolite.
Figure shows the influence of O2 and NO (other components, beside N2O, present in flue gases from the nitric acid plants) on the N2O conversion over the chosen hierarchical sample – Fe-βMCF-wa. The presence of oxygen did not affect significantly the catalyst activity, while the addition of NO to the feed greatly enhances the rate of N2O decomposition. The strong promotion of N2O decomposition by NO is a known feature of Fe-zeolites [Citation15]. NO removes oxygen atom deposited on the catalysts surface with the formation of NO2 (2). The surface oxygen is a product of N2O decomposition (1) and its desorption is considered as a rate determining step in this process. The NO2 molecule reacts with another oxygen atom, liberating O2 and NO (3) [Citation16],
Despite only small amount of the zeolitic phase impregnated on the Fe-βMCF-wa sample, the small addition of NO to the feed gases caused the characteristic for Fe-zeolites increase in catalytic activity.
Despite only small amount of the zeolitic phase impregnated on the Fe-βMCF-wa sample, the small addition of NO to the feed gases caused the increase in catalytic activity, which is a typical feature observed for Fe-modified zeolites.
It should be noted that an opposite effect of NO addition is usually observed for other catalytic systems [Citation15].
4. Conclusions
Four different impregnation methods were used for synthesis of micro-mesoporous materials. All of them resulted in successful introduction of the zeolitic phase into MCF, which was confirmed by XRD method. Depending on the used impregnation method different amounts and characteristics of β zeolite phase deposited on the MCF surface were received. These differences affected the form of iron introduced to the samples by ion-exchange method. The iron-exchanged hierarchical materials as well as Fe-MCF and Fe-β (as reference samples) were tested as catalysts in the N2O decomposition reaction. The deposition of Beta nanoparticles on the surface of MCF significantly increased its catalytic activity. The highest activity presented conventional Beta zeolite (100% of N2O conversion at about 798 K), but the activity of hierarchical samples, despite the significantly lower content of the zeolitic phase, was shifted to higher temperatures only by about 50 K. It should be also noted that the production of the impregnated samples is preferable from the economical point of view (smaller amount the zeolitic phase and shorter crystallization time).
Acknowledgements
M.R. acknowledges the financial support from the International PhD-studies programme at the Faculty of Chemistry Jagiellonian University within the Foundation for Polish Science MPD Programme co-financed by the EU European Regional Development Fund. The research was carried out with the equipment purchased thanks to the financial support of the European Regional Development Fund in the framework of the Polish Innovation Economy Operational Program (contract no. POIG.02.01.00-12-023/08).
References
- Ĉejka , J , Centi , G , Perez-Pariente , J and Roth , W J . 2012 . Zeolite-based materials for novel catalytic applications: Opportunities, perspectives and open problems. . Catal Today. , 179 : 2 – 15 . doi: 10.1016/j.cattod.2011.10.006
- Corma , A. 2003 . State of the art and future challenges of zeolites as catalysts. . J Catal. , 216 : 298 – 312 . doi: 10.1016/S0021-9517(02)00132-X
- van Donk , S , Jannsen , A H , Bitter , J H and de Jong , K P . 2003 . Generation, characterization, and impact of mesopores in zeolite catalysts. . Catal Rev. , 45 : 297 – 319 . doi: 10.1081/CR-120023908
- Ĉejka , J and Mintova , S . 2007 . Perspectives of micro/mesoporous composites in catalysis. . Catal Rev. , 49 : 457 – 509 . doi: 10.1080/01614940701583240
- Egeblad , K , Christensen , C hH , Kustova , M and Christensen , C H . 2008 . Templating mesoporous zeolites. . Chem Mater. , 20 : 946 – 960 . doi: 10.1021/cm702224p
- Van Oers , C J , Stevens , W JJ , Bruijn , E , Mertens , M , Lebedev , O I , Van Tendeloo , G , Meynen , V and Cool , P. 2009 . Formation of a combined micro- and mesoporous material using zeolite Beta nanoparticles. . Micropor Mesopor Mater. , 120 : 29 – 34 . doi: 10.1016/j.micromeso.2008.08.056
- Meyen , V , Cool , P and Vansant , E F . 2009 . Verified syntheses of mesoporous materials. . Micropor Mesopor Mater. , 125 : 170 – 223 . doi: 10.1016/j.micromeso.2009.03.046
- Chmielarz , L , Rutkowska , M , Kuśtrowski , P , Drozdek , M , Piwowarska , Z , Dudek , B , Dziembaj , R and Michalik , M. 2011 . An influence of thermal treatment conditions of hydrotalcite-like materials on their catalytic activity in the process of N2O decomposition. . J Therm Anal Calor. , 105 : 161 – 170 . doi: 10.1007/s10973-011-1284-4
- Omegna , A , Vasic , M , van Bokhoven , J A , Pirngruber , G and Prins , R. 2004 . Dealumination and realumination of microcrystalline zeolite beta: An XRD, FTIR and quantitative multinuclear (MQ) MAS NMR study. . Phys Chem Chem Phys. , 6 : 447 – 452 . doi: 10.1039/b311925d
- Camblor , M A , Corma , A and Valencia , S. 1998 . Characterization of nanocrystalline zeolite Beta. . Micropor Mesopor Mater. , 25 : 59 – 74 . doi: 10.1016/S1387-1811(98)00172-3
- Dzwigaj , S , Massiani , P , Davidson , A and Che , M. 2000 . Role of silanol groups in the incorporation of V in B zeolite. . J Mol Catal A. , 155 : 169 – 182 . doi: 10.1016/S1381-1169(99)00332-5
- Baran , R , Millot , Y , Onfroy , T , Krafft , J-M and Dzwigaj , S. 2012 . Influence of the nitric acid treatment on Al removal, framework composition and acidity of BEA zeolite investigated by XRD, FTIR and NMR. . Micropor Mesopor Mater. , 163 : 122 – 133 . doi: 10.1016/j.micromeso.2012.06.055
- Pérez-Ramiréz , J , Groen , J C , Brückner , A , Kumar , M S , Bentrup , U , Debbagh , M N and Villaescusa , L A . 2005 . Evolution of isomorphously substituted iron zeolites during activation: Comparison of Fe-beta and Fe-ZSM-5. . J Catal. , 232 : 318 – 334 . doi: 10.1016/j.jcat.2005.03.018
- Gurgul , J , Łątka , K , Hnat , I , Rynkowski , J and Dzwigaj , S. 2013 . Identification of iron species in FeSiBEA by DR UV–vis, XPS and Mössbauer spectroscopy: Influence of Fe content. . Micropor Mesopor Mater. , 168 : 1 – 6 . doi: 10.1016/j.micromeso.2012.09.015
- Pérez-Ramiréz , J , Kapteijn , F , Mul , G and Moulijn , J A . 2002 . NO-Assisted N2O decomposition over Fe-Based catalysts: Effects of gas-phase composition and catalyst constitution. . J Catal. , 208 : 211 – 223 . doi: 10.1006/jcat.2002.3559
- Pirngruber , G D and Pieterse , J AZ . 2006 . The positive effect of NO on the N2O decomposition activity of Fe-ZSM-5: A combined kinetic and in situ IR spectroscopic study. . J Catal. , 237 : 237 – 247 . doi: 10.1016/j.jcat.2005.11.012