ABSTRACT
The objective of this study was to identify geographic, dietary, and other predictors for childhood exposure to perfluoroalkyl substances (PFASs), polychlorinated biphenyls (PCBs), and methylmercury in Greenlandic children. The study includes cross-sectional data from 367 Greenlandic children aged 7 to 12 years examined during 2012–2015.
A parent or guardian participated in a structured interview, and a blood sample from the child was analysed for PFASs, PCBs and total mercury. Predictors for the environmental exposures were identified using linear regression. Area of residence was found to have the strongest explanatory power, accounting for 24% to 68% of the variance in the serum concentrations. Information about diet was available for two-thirds of the children, and among these, consumption of traditional Greenlandic food accounted for 2% to 10% of the variance in the biomarker concentrations. Models including all predictors associated with at least one of the environmental chemicals explained 19% to 54% of the total variance. In conclusion, area is a likely proxy for a traditional marine diet, and together area and diet constitute the most important predictors of exposure to methylmercury, PCBs and PFASs among Greenlandic children.
Introduction
While Greenland and other arctic areas are remote from industrial centres, the Arctic region can act as a sink for global pollutants [Citation1]. In addition to the primary emissions, exposure levels are affected by changes in subsequent re-emissions under conditions of climate change, biomagnification, and traditional food habits of the Arctic residents. The Greenland population is of particular concern in regard to highly elevated exposures to polychlorinated biphenyls (PCBs) [Citation2] and mercury [Citation3,Citation4]. While mercury is a natural component of the planet that has been redistributed into the biosphere [Citation5], the PCBs are industrial compounds that have been banned since the 1970s, but remain as environmental contaminants due to their persistence and continued releases from products manufactured in the past [Citation6]. In contrast, the perfluoroalkyl substances (PFASs) are still being produced and applied in industrial and commercial products including firefighting foam and surface treatment for furniture, outdoor clothing, cookware, and food wrapping paper, and humans are therefore potentially exposed through contaminated water, food, and dust from treated textiles [Citation7–Citation9]. Due to the widespread use, PFASs continue to be released to the environment, where they accumulate [Citation8]. Thus, traditional Arctic food contains higher concentrations of PFASs, PCBs and mercury [Citation10], thereby putting the populations who rely on local marine food at an increased risk, especially since non-food sources are likely to be of lesser importance. Often, Greenlandic mothers breastfeed for an extended duration [Citation11], and both PCBs and PFASs appear to be transferred via human milk as an important pathway of exposures in children, while lowering the mother’s serum concentrations [Citation12,Citation13].
Human exposure assessments are needed to characterise the changing exposures in remote Arctic areas, where the huge and sparsely populated areas present logistic challenges, but where continued efforts are badly needed [Citation14]. The aim of this study was therefore to identify geographic, dietary, and other predictors for childhood exposure to PFASs, PCBs, and mercury in Greenlandic children.
Methods
Setting
Greenland is a self-governing country within the Kingdom of Denmark, with a population close to 56,000 that reside along the coasts of the island [Citation15]. The traditional Greenlandic diet consists of marine food, including whale and seal meat and blubber, thereby exposing the population to environmental chemicals that biomagnify in the marine food chain.
In the OCEANS study, we invited 398 Greenlandic children whose mothers had been included in the Greenlandic part of the INUENDO cohort study [Citation16] and/or in the IVAAQ cohort study [Citation17] during pregnancy. The pregnant women were recruited during 2002–2005, and their children were invited for the present study during 2012–2015. In the IVAAQ study, 450 pregnant women from Nuuk, the capital of Greenland, Illulisat in the Disko Bay area, and Maniitsoq, all on the west coast were invited, and 270 participated. From the INUENDO study, 598 pregnant women from all regions of Greenland had participated among 665 eligible [Citation18]. Some women participated in both the IVAAQ and the INUENDO study. All children from the INUENDO and IVAAQ studies presently living in Maniitsoq and Sisimiut (West coast, north of Nuuk), Ilulissat, Aasiaat, Qeqertarsuaq, Qasigiannguit (all part of the Disko Bay area), and Tasiilaq (East coast) were invited to participate in the OCEANS study, while children living in Nuuk were invited only until the point where the study had reached the required size. shows a map of Greenland with the study areas indicated. The eight towns differed in many aspects among which population size and dietary habits were germane to the study. Nuuk is the capital and largest town in Greenland and the consumption of marine food was the lowest. Maniitsoq and Sisimiut are among the larger towns and situated on the central west coast not far from Nuuk. The consumption of marine food was below average for the country. The four towns in the Disko Bay area (Aasiaat, Qasigiannguit, Ilulissat, and Qeqertarsuaq) are small and large towns on the northwest coast of Greenland with a consumption of marine food above average for the country. Finally, Tasiilaq is a small town on the remote east coast with the highest consumption of marine food. Together the towns approximated a reasonable average for the whole of Greenland. Among the 398 children invited to the OCEANS study, 367 children aged 7 to 12 years chose to participate, 242 from the INUENDO study, 81 from the IVAAQ study and 45 who had been included in both studies (). A parent or guardian was asked to participate in a structured interview including questions about breastfeeding, number of siblings, family smoking, indoor smoking in the child’s home, and how many times per week the child had traditional Greenlandic food as the hot meal (an indicator of locally harvested marine food). Furthermore, the child was weighed, measured, and asked to provide a blood sample for analyses of contaminants.
The OCEANS study was performed in accordance with the Helsinki declaration. The ethical review committee serving Greenland and Human Subjects Committee Review Board of Harvard T.H. Chan School of Public Health approved the study protocol and written informed consent was obtained from a parent/guardian to all children included in the study.
Mercury, PCB, and PFAS exposure assessment
A few children did not provide a blood sample, and unfortunately, all samples from Qasigiannguit were lost in transit, resulting in 338 available blood samples (92%). PFASs and PCBs were measured in all samples while mercury was measured in 333 blood samples because five children did not provide enough blood.
All analyses were conducted in the laboratory at University of Southern Denmark. Total mercury was determined in volume of 100 μL of whole blood on a Direct Mercury Analyzer system (DMA-80) from Milestone, Sorisole, Italy [Citation19]. Certified quality control samples from Seronorm Trace Elements (Sero, Norway) were included in all sample series, and the calculated imprecision was less than 5%.
Serum concentrations of major PCB congeners were determined by use of Solid-Phase Extraction (SPE) and isotope dilution using a Thermo Ultra Gas Chromatograph with a PTV injector coupled to a Thermo TSQ XLS Triple Quadrupole Mass Spectrometer (Thermo Scientific, San José, CA). The extraction of compounds was performed by Solid-Phase Extraction (SPE), as described by Petersen et al. [Citation20], although slightly modified for use with mass spectrometry. In brief, an aliquot of 300 μL of serum and 25 μL of an internal standard mixture of isotope-labelled versions of the analytes were diluted with 300 µL of Milli-Q water and 100 μL of concentrated formic acid, followed by whirl mixing and ultrasonic treatment for 5 min. The sample was transferred to a preconditioned Isolute C18 (100 mg, 3 mL) SPE column (Biotage, Uppsala, Sweden) and eluted slowly by use of positive pressure. The SPE column is then washed with 1.2 mL Milli-Q treated water, and the compounds are then eluted with 3 × 1.2 mL isooctane and collected in a conical glass test tube. The sample was evaporated to almost dryness at 30°C under a gentle stream of nitrogen and then resuspended in 200 μL isooctane. An aliquot of 4 µL is injected into a PTV injector on the GC-MS/MS system.
The concentration of the PCBs was expressed in relation to the total lipid concentration determined on basis of the cholesterol and triglyceride content of the serum sample [Citation21]. The cholesterol and triglyceride content were determined enzymatically by a kit-based routine analysis on a Konelab 20 Clinical Chemistry Analyzer (Thermo Scientific, Oy, Finland), with a between batch imprecision of <12%. The limit of detection (LOD) for PCB congeners in these analyses was 0.03 ng/mL, which corresponds to 0.003 μg/g lipid at an average serum-lipid concentration of 10 g/L [Citation20]. All values below the LOD were replaced with LOD/2. The three major PCB congeners CB-138, CB-153, and CB-180 account for approximately half of the total PCB concentration [Citation22], and a simplified ΣPCB concentration was calculated as the sum of these three congeners multiplied by 2.
The five most abundant PFASs, i.e. perfluorohexane sulfonic acid (PFHxS), perfluorooctane sulfonic acid (PFOS), perfluorooctanoic acid (PFOA), perfluorononanoic acid (PFNA), and perfluorodecanoic acid (PFDA) were quantified using on-line solid-phase extraction followed by liquid chromatography and triple quadrupole mass spectrometry [Citation23]. Each analysed batch of samples included a calibration curve spiked in foetal bovine serum (Sigma Aldrich, St. Louis, MO), solvent and matrix blanks, as well as quality control (QC) samples. The QC samples were NIST SRM 1958, as well as in-house made QC samples. The batch imprecision was <5.2%, and the limit of detection (LOD) was 0.03 ng/mL. The LOD was assessed in foetal bovine serum, from a criteria of a signal-to-noise ratio of the peak >3 and the CV% of spiked samples <20%.
The accuracy of the PCB and PFAS methods is continuously secured by regular participation in the German Quality Assessment Scheme (G-EQUAS) organised by the German Society of Occupational Medicine. The accuracy of the PFAS measurements during analysis of the samples ranged from 98.5% to 111%, and the PCB measurements from 98.0% to 106%.
Statistics
Potential predictors for one or more of the environmental exposures were identified in the existing literature, i.e. diet [Citation24–Citation27], area of residence [Citation24], sex [Citation28], duration of breastfeeding [Citation25–Citation27,Citation29], number of siblings [Citation27], exposure to smoking [Citation27], age [Citation25,Citation30], and BMI [Citation25]. BMI was calculated as weight/height ratio squared. Age- and sex-adjusted cut-off points for overweight and obesity were calculated as suggested by Cole et al. [Citation31].
Correlations between the log-transformed exposures were examined using Person’s correlation coefficient. The associations between each environmental chemical and each potential predictor were assessed using unadjusted linear regression. Area of residence was assumed to be the most likely confounder for the associations, and all regression analyses were therefore repeated adjusting for this variable. Ordinal categorical variables were tested using a trend test. Due to the left skewed distribution of the environmental chemical concentrations, all concentrations were log-10-transformed, and the estimates of association from the regression models were transformed to express the per cent difference in environmental chemical concentrations. The explanatory power of the models containing different sets of predictors was evaluated using R-square. The distributions of model residuals were inspected in histograms and quantile-quantile (qq)-plots. Equality of variance was tested using Bartlett’s test when comparing groups and inspected in residuals-versus-fitted values (rvf)-plots for continuous variables and when performing multiple linear regression. Influential points were examined by inspecting dfbeta values, none of which exceeded 1. Bartlett’s test showed significant heteroskedasticity in some analyses and inspections of histograms and qq-plots showed slight deviations from the normal distribution of residuals for some analyses. All regression analyses were therefore performed with robust standard errors. Analyses were carried out in Stata version 15.0 (StataCorp, College Station, TX, USA), and a significance level of 0.05 was applied for detecting important associations.
Results
Characteristics of the 367 children included in the study are shown in . A total of 365 parents/guardians participated in a structured interview, and 345 children were weighed and measured. Six per cent of the children were obese and an additional 21% were overweight. Information about consumption of traditional Greenlandic food was missing for one-third of the children, including all children from Sisimiut, Aasiaat, and Qasigiannguit. Among the remaining children, 61% had traditional Greenlandic food as a hot meal at least twice a week. The consumption of traditional Greenlandic food was lowest in Nuuk and highest in the Disko Bay area (Ilulissat and Qeqertarsuaq) as illustrated in .
Table 1. Characteristics of children included in the study
Figure 3. Traditional Greenlandic food consumption by area. Information about diet was not available for children from Sisimiut, Aasiaat and Qasigiannguit
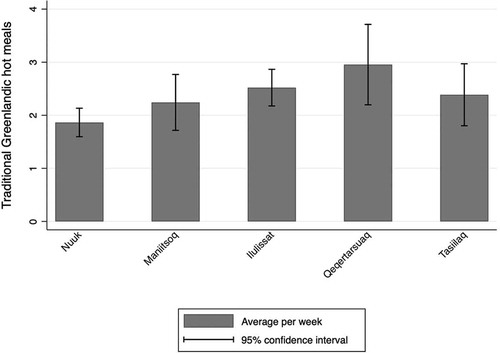
Mercury and all five PFASs were found in concentrations above the LOD in all measured samples. PCB congeners CB-138 and CB-153 were each found to be below the LOD in five samples, while CB-180 was found to be below the LOD in 12 samples. Only one sample had concentrations of all three congeners below the LOD. The median mercury concentration was 3.83 μg/L, the median ΣPCB was 0.26 μg/g lipid and PFAS medians were between 0.49 (PFDA) and 8.68 (PFOS) ng/mL (). Most of the correlations between the exposures were moderate or low, and PFOA was only negligible correlated to mercury and PCB exposure. However, PFNA was highly correlated with PFHxS, PFOS and PFDA, and PFHxS was additionally highly correlated with PFOS ().
Table 2. Pearson’s correlation coefficients for the log-transformed environmental exposures
Area of residence was significantly associated with exposure to all of the environmental chemicals (). Children in Nuuk had the lowest concentrations of mercury, ΣPCB, and PFOS, whereas children in Sisimiut had the lowest concentrations of PFHxS, PFOA, PFNA, and PFDA. However, the differences between Nuuk and Sisimiut were significant only for ΣPCB, PFOA, and PFDA with concentrations being 55% higher, 16% lower, and 63% lower in Sisimiut than in Nuuk, respectively. Children in Tasiilaq (East Greenland) had the highest concentrations of ΣPCB, PFHxS, PFOS, PFNA, and PFDA with concentrations being 9.1, 3.3, 2.6, 2.1, and 2.4-fold higher, respectively, than those found among children in Nuuk. Children in Qeqertarsuaq had the highest concentrations of mercury with concentrations being 4.1 times higher than in Nuuk, while children in Ilulissat had the highest concentrations of PFOA with concentrations being 29% higher than in Nuuk. In general, children in the Disko Bay area (Ilulissat, Aasiaat, and Qeqertarsuaq) had higher exposures to most of the environmental chemicals compared to children in Nuuk. However, PFDA concentrations were 58% lower in Aasiaat compared to Nuuk ().
Table 3. Per cent difference in mercury, ΣPCB, and PFASs with potential predictors. For total numbers in subgroups see
Consumption of Greenlandic food was also significantly associated with increased exposure to all the environmental chemicals (). Adjusting for area of residence slightly attenuated the associations, and the association between consumption of Greenlandic food and exposure to PFOA did not remain significant (). In the adjusted model, each extra day per week with consumption of Greenlandic food was associated with 13% increased mercury and ΣPCB, 8% increased PFHxS and PFOS, 7% increased PFNA, and 9% increased PFDA concentrations.
Table 4. Per cent difference in mercury, ΣPCB, and PFASs with potential predictors – adjusted for area of residence. For total numbers in subgroups see
Older age, being a girl, shorter duration of being breastfed, being overweight or obese, living with smokers, and living in a house with indoor smoking were each significantly associated with decreased concentrations of one or two environmental chemicals (). However, after adjusting for area of residence, some of the associations changed, and indoor smoking was no longer associated with any of the environmental chemicals, while having two or more younger siblings were significantly associated with decreased concentrations of PFOS. The number of older siblings was not associated with any of the environmental chemicals.
Among the different predictors, area of residence had the strongest explanatory power, accounting for between 24% (PFOA and PFNA) and 68% (PFDA) of the variance in the chemical exposures when using all cases and between 13% (PFOA) and 42% (PFHxS) when using complete cases only, which excludes children from Sisimut, Aasiaat, and Qasigiannguit. Greenlandic food consumption accounted for between 2% (PFOA) and 10% (PFDA), and the combined effect of area of residence and Greenlandic food consumption accounted for between 13% (PFOA) and 47% (PFHxS) using complete cases. Models including all predictors associated with at least one of the environmental chemicals explained between 18% (PFOA) and 54% (ΣPCB) of the total variance using complete cases ().
Table 5. Explanatory power for models containing different sets of predictors using all cases and complete cases
Discussion
The Greenlandic children in this study had blood-mercury concentrations 12-fold higher than those found among 6–11-year-old children in the US National Health and Nutrition Examination Survey (NHANES) (2015–16) [Citation32], and serum-PCB concentrations in the Greenlandic children were likewise highly elevated as compared to American 12–19-year-olds in NHANES 2003–04 [Citation33], though lower than those found at age 5 and 7 among Faroese children born in 1999–2001 [Citation34]. Of particular interest, PFOS concentrations in the Greenlandic children were almost doubled and PFNA and PFDA highly elevated compared to those occurring in American 6–11-year-olds in NHANES 2013–14 [Citation32] and Faroese 5-year-olds born in 2007–09 [Citation35]. PFOA concentrations were also slightly higher than those found in the American 6–11-year-olds and Faroese 5-year-olds, while PFHxS was higher than among the Faroese 5-year-olds but lower than among American 6–11-year-olds [Citation32,Citation35]. These differences suggest that elevated exposures occur for some chemicals, but available comparison data are insufficient in regard to the ages and the calendar years covered to allow detailed conclusions.
In this study, we performed a large number of tests, thereby increasing the risk of type I errors. Thus, more emphasis should be given to predictors being consistently associated with the environmental chemicals than to those for which only single significant associations were found. Among main predictors, area of residence was consistently associated with exposure to environmental chemicals. Children in Nuuk and Sisimiut were less exposed to environmental chemicals than children in Maniitsoq, the Disko area, and Tasiilaq. Furthermore, increased consumption of traditional Greenlandic food was associated with increased concentrations of environmental chemicals, which is in accordance with the findings from a recent study among Greenlandic adults [Citation36]. PFOA exposure was not as strongly associated with Greenlandic food consumption as the other environmental chemical exposures, indicating that PFOA must have other origins, such as food wrapping materials [Citation37], which is consistent with PFOA concentrations varying less by area compared to the other environmental chemicals. These findings are similar to observations in the Faroes, where PFOS and PFNA correlated well with the frequency of whale meat dinners, while PFOA did not [Citation38]. Contaminant concentrations in edible species may vary geographically and with size, and such variations makes it hard to obtain exposure information from dietary questionnaires.
The dietary questionnaire was brief in order to secure maximal participation and details on portion sizes, species and similar details were not obtained. Because of the limited dietary information, and because blood concentrations reflect the impact of exposures during a time period much longer than can be reasonably assessed by a questionnaire, we are unable to pinpoint specific dietary sources of the environmental chemical exposures. Greenlandic food includes fish, which is likely not heavily contaminated, while mammals are more contaminated by the substances measured in the blood samples [Citation1]. The frequency of Greenlandic food consumption is thus an imprecise proxy measure of exposure. More detailed dietary information covering a substantial period may have produced stronger associations with the environmental chemical exposures. Furthermore, information about consumption of traditional Greenlandic food was missing for one-third of the children, including all children from three of the study areas, thereby also preventing us from imputing the missing data. Thus, we cannot draw strong conclusions on the associations between diet and contaminant exposures.
In accordance with the existing knowledge [Citation25], we found overweight and obesity to be associated with lower serum concentrations of PCB, most likely explained by a greater lipid compartment that reduces the lipid-based serum-PCB concentrations. Furthermore, duration of breastfeeding was associated with increased serum concentrations of PCBs and PFHxS. These substances are transferred through breast milk [Citation12,Citation13,Citation26,Citation29], and the long elimination half-lives of these compounds makes it possible to detect this effect in serum several years later [Citation39], although the effects are not as strong as seen among younger Faroese and Norwegian children [Citation13,Citation29].
Living together with one or more smokers was found to be associated with lower serum concentrations of PFOS and PFDA, which is in accordance with findings from the Faroe Islands [Citation27]. When adjusting for area of residence, indoor smoking by itself was not associated with any of the chemical exposures, indicating that the association between living with smokers and reduced PFAS exposure is not causal but more likely due to underlying differences, e.g. family health behaviour.
Older age was associated with lower PCB and PFOA concentrations, possibly due to a decrease with age of chemical concentrations from exposure in-utero and through breastfeeding. It might, however, also be a result of younger children consuming more food relative to their body size and more frequently transferring chemicals from the surrounding environment to their mouth [Citation40,Citation41]. Finally, being female was associated with lower mercury exposure, but not with any of the other environmental chemical exposures. In adolescents and adults, men have been found to have higher serum-PFAS concentrations compared to women [Citation27,Citation42], but this can to some degree be explained by PFASs being eliminated with menstruation, childbirth and breastfeeding [Citation43]. Thus, it seems reasonable that we do not find significant sex-differences in PFAS exposure in our study population of children.
To our knowledge, not much is known about the relative exposure from the different sources in Greenland. We have tried to contribute with information about exposures in the data we have, but we do not have information about all potential sources. Other factors than those included in this study are likely to affect exposure to environmental chemicals among Greenlandic children, which is also demonstrated by the fact that only 18–53% of the variance in chemical exposure was explained by our full model. Area alone explains a large proportion of the variance, and it should be considered that some areas are not included in the full model due to missing data. Indoor dust is a potential PFAS source, though with more limited access to some consumer products, PFAS intake from dust in Greenland is probably less than in other locations, where it is of minor impact [Citation44]. Furthermore, household dust was found not to contribute to PFAS exposure among Alaska natives [Citation45]. In other settings, environmental exposures have also been associated with socioeconomic status (SES) [Citation46], but SES is unlikely to be a major predictor of exposure among Greenlandic children. Although there are variations in SES within the Greenlandic population, the differences are less pronounced than in, e.g. the U.S. population.
A major strength of this study is the willingness among the children to provide a blood sample, ensuring information about environmental chemical exposure for 92% of the participating children. However, this study does not cover all inhabited areas and therefore may not fully represent all Greenlandic children.
In conclusion, we find that area of residence and consumption of traditional Greenlandic food are the most important predictors of exposure to mercury, PCBs and PFASs among Greenlandic children.
Disclosure statement
No potential conflict of interest was reported by the authors.
Additional information
Funding
References
- Arctic Monitoring and Assessment Programme (AMAP). AMAP Assessment 2016: Chemicals of Emerging Arctic Concern. Oslo, Norway: Arctic Monitoring and Assessment Programme (AMAP); 2017.
- Bjerregaard P, Dewailly E, Ayotte P, et al. Exposure of inuit in Greenland to organochlorines through the marine diet. J Toxicol Environ Health Part A. 2001;62:69–11.
- Johansen P, Pars T, Bjerregaard P. Lead, cadmium, mercury and selenium intake by Greenlanders from local marine food. Sci Total Environ. 2000;245:187–194.
- Weihe P, Hansen JC, Murata K, et al. Neurobehavioral performance of inuit children with increased prenatal exposure to methylmercury. Int J Circumpolar Health. 2002;61:41–49.
- Chen CY, Driscoll CT, Lambert KF, et al. Connecting mercury science to policy: from sources to seafood. Rev Environ Health. 2016;31:17–20.
- Beyer A, Biziuk M. Environmental fate and global distribution of polychlorinated biphenyls. Rev Environ Contam Toxicol. 2009;201:137–158.
- Domingo JL, Nadal M. Per- and Polyfluoroalkyl Substances (PFASs) in food and human dietary intake: A review of the recent scientific literature. J Agric Food Chem. 2017;65:533–543. Epub 2017/ 01/05.
- Agency for Toxic Substances and Disease Registry. Toxicological profile for perfluoroalkyls. Draft for public comment. U.S.: Department of Health and Human Services; 2018.
- Sunderland EM, Hu XC, Dassuncao C, et al. A review of the pathways of human exposure to poly- and perfluoroalkyl substances (PFASs) and present understanding of health effects. J Expo Sci Environ Epidemiol. 2019;29:131–147. Epub 2018/ 11/25.
- Arctic Monitoring and Assessment Program. Chemicals of emerging Arctic concern. Oslo, Norway: Arctic Monitoring and Assessment Programme (AMAP); 2017.
- Knudsen AK, Long M, Pedersen HS, et al. Lifestyle, reproductive factors and food intake in Greenlandic pregnant women: the ACCEPT - sub-study. Int J Circumpolar Health. 2015;74:29469.
- Jensen AA, Slorach S. Chemical contaminants in human milk. Boca Raton: CRC Press; 1991.
- Mogensen UB, Grandjean P, Nielsen F, et al. Breastfeeding as an exposure pathway for perfluorinated alkylates. Environ Sci Technol. 2015;49:10466–10473.
- Odland JO, Nieboer E. Human biomonitoring in the arctic. Special challenges in a sparsely populated area. Int J Hyg Environ Health. 2012;215:159–167.
- Statistics Greenland. Greenland in figures. 2016. Available from: www.stat.gl
- Hoyer BB, Ramlau-Hansen CH, Henriksen TB, et al. Body mass index in young school-age children in relation to organochlorine compounds in early life: a prospective study. Int J Obes (Lond). 2014;38:919–925. Epub 2014/ 04/11.
- Bjerregaard P, Holm A, Olesen I, et al. Ivaaq—the Greenland inuit child cohort, a preliminary report. Vol. 17. Copenhagen: National Institute of Public Health. DICE - Writings on Greenland; 2007.
- Toft G, Axmon A, Giwercman A, et al. Fertility in four regions spanning large contrasts in serum levels of widespread persistent organochlorines: a cross-sectional study. Environ Health. 2005;4:26. Epub 2005/ 11/11.
- Kim BM, Choi AL, Ha EH, et al. Effect of hemoglobin adjustment on the precision of mercury concentrations in maternal and cord blood. Environ Res. 2014;132:407–412.
- Petersen MS, Halling J, Damkier P, et al. Caffeine N3-demethylation (CYP1A2) in a population with an increased exposure to polychlorinated biphenyls. Eur J Clin Pharmacol. 2006;62:1041–1048.
- Phillips DL, Pirkle JL, Burse VW, et al. Chlorinated hydrocarbon levels in human serum: effects of fasting and feeding. Arch Environ Contam Toxicol. 1989;18:495–500. Epub 1989/ 07/01.
- Grandjean P, Weihe P, Needham LL, et al. Relation of a seafood diet to mercury, selenium, arsenic, and polychlorinated biphenyl and other organochlorine concentrations in human milk. Environ Res. 1995;71:29–38.
- Haug LS, Thomsen C, Becher G. A sensitive method for determination of a broad range of perfluorinated compounds in serum suitable for large-scale human biomonitoring. J Chromatogr A. 2009;1216:385–393.
- Deutch B, Dyerberg J, Pedersen HS, et al. Dietary composition and contaminants in north Greenland, in the 1970s and 2004. Sci Total Environ. 2006;370:372–381.
- Burns JS, Williams PL, Sergeyev O, et al. Predictors of serum dioxins and PCBs among peripubertal Russian boys. Environ Health Perspect. 2009;117:1593–1599.
- Haug LS, Huber S, Becher G, et al. Characterisation of human exposure pathways to perfluorinated compounds–comparing exposure estimates with biomarkers of exposure. Environ Int. 2011;37:687–693.
- Timmermann CA, Budtz-Jorgensen E, Jensen TK, et al. Association between perfluoroalkyl substance exposure and asthma and allergic disease in children as modified by MMR vaccination. J Immunotoxicol. 2017;14:1–11.
- Timmermann CA, Budtz-Jorgensen E, Petersen MS, et al. Shorter duration of breastfeeding at elevated exposures to perfluoroalkyl substances. Reprod Toxicol. 2017;68:164–170.
- Caspersen IH, Kvalem HE, Haugen M, et al. Determinants of plasma PCB, brominated flame retardants, and organochlorine pesticides in pregnant women and 3 year old children in the norwegian mother and child cohort study. Environ Res. 2016;146:136–144.
- Harris MH, Rifas-Shiman SL, Calafat AM, et al. Predictors of Per- and Polyfluoroalkyl Substance (PFAS) plasma concentrations in 6-10 year old American children. Environ Sci Technol. 2017;51:5193–5204.
- Cole TJ, Bellizzi MC, Flegal KM, et al. Establishing a standard definition for child overweight and obesity worldwide: international survey. BMJ. 2000;320:1240–1243.
- U.S. Department of Health and Human Services Centers for Disease Control and Prevention (CDC). Fourth National Report on Human Exposure to Environmental Chemicals - Updated Tables, January 2019, Volume One. 2019.
- U.S. Department of Health and Human Services Centers for Disease Control and Prevention (CDC). Fourth National Report on Human Exposure to Environmental Chemicals - Updated Tables, January 2019, Volume Two. 2019.
- Heilmann C, Budtz-Jorgensen E, Nielsen F, et al. Serum concentrations of antibodies against vaccine toxoids in children exposed perinatally to immunotoxicants. Environ Health Perspect. 2010;118:1434–1438. Epub 2010/ 06/22.
- Grandjean P, Heilmann C, Weihe P, et al. Estimated exposures to perfluorinated compounds in infancy predict attenuated vaccine antibody concentrations at age 5-years. J Immunotoxicol. 2017;14:188–195.
- Schaebel LK, Bonefeld-Jorgensen EC, Vestergaard H, et al. The influence of persistent organic pollutants in the traditional inuit diet on markers of inflammation. PLoS One. 2017;12:e0177781.
- Trier X, Granby K, Christensen JH. Polyfluorinated surfactants (PFS) in paper and board coatings for food packaging. Environ Sci Pollut Res Int. 2011;18:1108–1120. Epub 2011/ 02/18.
- Weihe P, Kato K, Calafat AM, et al. Serum concentrations of polyfluoroalkyl compounds in Faroese whale meat consumers. Environ Sci Technol. 2008;42:6291–6295.
- Barr DB, Weihe P, Davis MD, et al. Serum polychlorinated biphenyl and organochlorine insecticide concentrations in a Faroese birth cohort. Chemosphere. 2006;62:1167–1182. Epub 2005/ 09/20.
- Trudel D, Horowitz L, Wormuth M, et al. Estimating consumer exposure to PFOS and PFOA. Risk Anal. 2008;28:251–269. Epub 2008/ 04/19.
- Kato K, Calafat AM, Wong LY, et al. Polyfluoroalkyl compounds in pooled sera from children participating in the national health and nutrition examination survey 2001-2002. Environ Sci Technol. 2009;43:2641–2647.
- Kato K, Wong LY, Jia LT, et al. Trends in exposure to polyfluoroalkyl chemicals in the U.S. population: 1999-2008. Environ Sci Technol. 2011;45:8037–8045.
- Wong F, MacLeod M, Mueller JF, et al. Enhanced elimination of perfluorooctane sulfonic acid by menstruating women: evidence from population-based pharmacokinetic modeling. Environ Sci Technol. 2014;48:8807–8814.
- Mitro SD, Dodson RE, Singla V, et al. Consumer product chemicals in indoor dust: A quantitative meta-analysis of U.S. studies. Environ Sci Technol. 2016;50:10661–10672. Epub 2016/ 09/15.
- Byrne S, Seguinot-Medina S, Miller P, et al. Exposure to polybrominated diphenyl ethers and perfluoroalkyl substances in a remote population of Alaska natives. Environ Pollut. 2017;231:387–395. Epub 2017/ 08/19.
- Buekers J, Colles A, Cornelis C, et al. Socio-economic status and health: Evaluation of human biomonitored chemical exposure to per- and polyfluorinated substances across status. Int J Environ Res Public Health. 2018;15(12):2818. Epub 2018/ 12/ 14. doi: 10.3390/ijerph15122818.