ABSTRACT
Wild game consumption has been associated with health benefits but the acute influence on human protein metabolism remains unknown. We compared feeding-induced responses of equivalent amounts of free-range reindeer (FR) and commercial beef (CB) on protein kinetics using stable isotope methodology. Seven participants (age: 40 ± 14 years; body mass index: 24 ± 3 kg/m2) completed two randomised studies, ingesting 2 oz of FR or CB. L-[ring 2H5]phenylalanine & L-[ring 2H2]tyrosine were delivered via primed, continuous intravenous infusion. Blood samples were collected during the basal period and following consumption of FR or CB. Feeding-induced changes in whole-body protein synthesis (PS), protein breakdown (PB), and net protein balance (NB) were determined via plasma sample isotope enrichment analysis by gas chromatography-mass spectrometry; plasma essential amino acid (EAA) concentrations were determined by liquid chromatography-electrospray ionisation-mass spectrometry. Plasma post-prandial EAA concentrations were higher with FR compared to CB (P < 0.05). The acute feeding-induced PS response was not different, but PB was reduced and contributed to a superior level of NB (P < 0.00001) in FR compared to CB. Our results demonstrate that FR may influence more favourable protein metabolism than CB. These data support potential health benefits of wild game onf whole-body protein.
Abbreviations: BMI: body mass index; DIAAS: digestible indispensable amino acid score; CB: commercial beef; EAA: essential amino acids; FR: free-range reindeer; Ra: rate of appearance; UAF: University of Alaska Fairbanks; USDA: USA Department of Agriculture
Introduction
The practical health benefits of wild, free-range red meats are particularly relevant to Alaska Native and Indigenous peoples whose ageing populations are increasing and challenged by the high risk of disability [Citation1–3]. Many of these disabilities are often associated with the initial loss of muscle that may occur in some individuals after age 30. Severe muscle loss (sarcopenia) is a progressive, insidious, and multifactorial disease that presents with decreased functional performance, age-related bone loss, increased falls and fractures, obesity, type II diabetes mellitus, depression, hospitalisation, and even mortality [Citation4]. Prevention of sarcopenia is particularly important in Alaska and other rural/remote areas. The interaction between healthcare delivery and disability is especially complex due to limited economic competition, higher profit margins, expensive medical infrastructure, and unique environmental and socio-demographic elements [Citation5]. Inadequate nutrition is often associated with sarcopenia [Citation6] and as ageing progresses, a protein shortfall occurs (loss of appetite, inadequate protein utilisation, anabolic resistance, and chronic diseases). This inadequacy can be deterred by consuming high-quality proteins which can stimulate protein synthesis and counteract muscle breakdown to create an overall positive net protein balance throughout the ageing process [Citation7]. Protein intake consisting of wild, free-range red meats with high-quality proteins and fats and high amounts of essential amino acids can reverse protein breakdown and improve muscle maintenance during ageing. Therefore, consumption of wild, free-range red meat may play an important role in supporting adequate net protein balance necessary for healthy and independent ageing [Citation8], especially in Alaska Native peoples.
The amino acid profile of wild, free-range red meat is an important contributor to its overall quality. Twenty total amino acids compose muscle protein, and nine of those are essential amino acids (EAA’s) [Citation9]. For positive net protein balance to occur, EAA’s must be provided through dietary intake and available in sufficient amounts [Citation10]. Free-range wild game is naturally high in EAA’s [Citation11]. The digestible indispensable amino acid score (DIAAS) is used to assess protein quality and monitor protein adequacy of foods sold to consumers [Citation12]. As a function of the amount and profile of EAAs per gram of meat and the true ileal digestibility [Citation13], the DIAAS may also be higher in wild, free-range red meats [Citation14]. While the influence of protein quality and quantity on muscle health has received global attention [Citation15], questions remain with regard to wild, free-range red meat and its effect on muscle preservation, an elusive goal for many older adults combatting sarcopenia [Citation16].
Consumers choose to prepare their meat protein choices based on portion sizes or by weight rather than nutritional composition or EAAs/serving. For this reason, the USA Department of Agriculture (USDA) recommends food servings by ounce-equivalents [Citation17]. To our knowledge, the acute ingestion of USDA-established equivalent servings of wild, free-range red meat and commercial red meat on protein kinetics in humans has not been evaluated. According to origins inferred from mitochondrial DNA sequences, reindeer and caribou represent a species of deer that vary in size and distribution throughout northern Europe, Siberia, and North America [Citation18]. While precise descriptions of nutrient composition data on free-range reindeer are scarce to non-existent, forage composition in free-range reindeer and caribou in Alaska are very similar; positively influencing the total amount of essential amino acids/total weight [Citation11,Citation19]. Therefore, we used the Nutritiondata.self.com [Citation20] database to access data on the nutritional composition of wild caribou as a theoretical equivalent to free-range reindeer (). If wild and/or free-range red meat/total weight provides a greater benefit in maintaining whole body net protein balance, well-informed decisions can be more clearly made by consumers or hunters as they evaluate their options for meat consumption.
Table 1. Nutritional composition
We compared the acute dietary influence of two ounces of free-range reindeer (FR) and commercial beef (CB) on protein metabolism via stable isotope methodology using a randomised, double blind, parallel study design in humans. As previously mentioned, FR is similar to wild caribou [Citation18], but can be processed and sold as a USDA-certified meat source for human consumption [Citation21]. We chose 70% lean/30% fat CB for comparison, as it represents the largest percentage of ground beef types sold in the USA each year by almost three-fold [Citation22]. These selections allowed practical comparisons between equal serving sizes of FR which might be analogous to caribou be consumed by a hunter to the CB that is preferentially purchased by the majority of the USA population. While the total amount of protein ingested is 30% higher with FR than CB, the total amount of calories is 40% less. Therefore, consumption of FR may enhance optimal net protein balance but reduce consumption of calories found in CB of an equivalent weight or serving size.
We hypothesised that FR would promote a higher whole body net protein balance compared to CB due to the greater amount of essential amino acids in FR. If our data support the superior efficacy of FR consumption per ounce on whole body net protein balance, we assert that wild, free-range red meats may augment the retention of whole-body protein to a greater extent than CB.
Materials and methods
Eight male and female participants of varying ages and ethnicities were recruited from the Fairbanks, Alaska area through a combination of local newspaper advertisements and flyers. All testing occurred at the Clinical Research and Imaging Facility on the University of Alaska Fairbanks (UAF) campus except baseline blood sampling for medical screening performed at LabCorp, Inc (1626 30th Ave, Fairbanks, AK 99701). All participants were consented prior to enrolment and copies of the consent forms were provided. All aspects of the study were reviewed and approved by the UAF Institutional Review Board.
The existence of metabolic disease can complicate feeding-induced changes in protein kinetics [Citation23]. Therefore, our strategy was directed towards the influence of FR and CM on protein kinetics in healthy adults and how potential differences might be relevant over the lifespan. We utilised the expertise of one study physician, licenced by the Alaska State Medical Board, who appraised all health data points to determine the eligibility of the participants. General health was evaluated through careful consideration of answers to a health questionnaire, bloodwork results, and body composition values. Inclusion criteria incorporated participants between the ages of 20 and 70 years of age with a Body Mass Index (BMI) of 20–38 kg/m2 and deemed as generally healthy by the study physician. Exclusion criteria included any individuals with a pacemaker, previously diagnosed diabetes, or chronic inflammatory condition, taking any type of medication or supplement that could have affected glucose metabolism, active cancers or malignancies, or taking corticosteroids by mouth, injection, or trans-dermally. Exclusion criteria also included females of childbearing ages who tested positively for pregnancy. Finally, any individual with any other disease that would have placed them at increased risk of harm if they were to participate would have been excluded at the discretion of the study physician.
To be considered for eligibility, participants completed a health questionnaire during the initial consent and screening visit. All medications and vitamins were listed, and participants were asked whether they had been diagnosed with diabetes or high blood pressure, experienced a heart attack, arrhythmia, heart valve disease, stents, pacemakers, defibrillators, chest pains, shortness of breath, or heart failure. Also included were questions concerning dizziness or numbness of limbs, difficulty walking, cramping, or joint or muscle problems. Participants revealed any benign or malignant cancers, thyroid/pituitary disease, and/or intestinal problems, and described alcohol and tobacco use. Body mass index was determined using height and weight. Blood sampling and analysis were completed by LabCorp provided lipid and metabolic values for screening purposes. Females of childbearing age were provided pregnancy tests to ensure eligibility for inclusion. Answers to the health questionnaire and values for body composition and bloodwork were evaluated by researchers and the study physician and any values that met the exclusion criteria would have confirmed ineligibility for the study. All volunteers that expressed interest in the study were eligible for participation by meeting all the inclusion criteria and none of the exclusion criteria.
Participant confidentiality was ensured for all participants. Protected records were kept in a locked file cabinet behind a locked door in a facility with restricted, swipe-card access. Blood samples collected by the study nurse were stored in a locked freezer of a swipe-card, access-restricted facility until analysis of isotopic enrichments, and then immediately destroyed. All blood samples collected by LabCorp were immediately destroyed following analysis.
Our study was completed in the Clinical Research and Imaging Facility in Room 015 of the Murie Building at UAF. There is a basement entry to the facility, providing privacy for all participants in the study. The facility is accessed by a swipe-card-only entry, and only those researchers that were part of this study could enter. The participants, while sitting for the isotope infusion procedures for 7 hours on two occasions, had access to medical-grade recliners specifically designed for long-term use. Participants were given iPads for watching movies, though some chose to use their computers. Several worked remotely while the isotope infusion/blood sampling was in progress. Participants were fasted upon arrival but could drink water throughout the day. The isotope infusate was placed on a mobile intravenous pole stand that could be rolled to the restroom at the request of the participants.
Eligible participants visited the Clinical Research and Imaging Facility at UAF on three separate occasions: a) consent and determination of eligibility status, b) feeding/tracer study #1, and c) feeding/tracer study #2. The feeding/tracer studies were performed using a randomised, c, parallel study design. In doing so, the response to the ingestion of 2-ounce pre-cooked servings of randomly assigned 95% lean/5% fat FR or 70% lean/30% fat CB was evaluated. These servings are consistent with the portion sizes listed in the Dietary Guidelines 2015–2020 [Citation24]. The nutritional profiles for FR and CB are listed in as derived from the Nutritiondata.self.com database.
The isotope infusion/feeding studies were completed on two occasions at least three days apart and after an overnight fast beginning at 2200 hours [Citation10]. To achieve simultaneous infusion/sampling, intravenous catheters were placed into each forearm; one for the infusion of stable isotope tracers and the other for the collection of arterialised blood sampling via the heated hand technique [Citation25]. A baseline blood sample was collected to determine background isotopic enrichments. Primed continuous infusions of l-[ring-2H5]phenylalanine (prime, 5.04 μmol/kg body weight; rate, 5.04 μmol·kg−1·h−1) and l-[ring-2H2]tyrosine (prime, 2.16 μmol/kg; rate, 1.995 μmol·kg−1·h−1) were performed to determine rates of protein synthesis, protein breakdown, and net protein balance at the whole body level [Citation10] (). All isotope tracers were purchased from Cambridge Isotope Laboratories (Andover, MA) and compounded by a licenced pharmacist. Tracer enrichment and plasma responses of amino acids were determined from blood samples collected at 0, 60, and 180 min to provide the fasted blood samples. Consumption of FR and CB occurred at 180 minutes, and additional blood samples followed at t = 210, 240, 300, 330, 360, and 420 min. A total of 12 blood samples were drawn during the study ().
Analytical methods
Plasma samples were deproteinized using dry sulfosalicylic acid, and frozen at −80°C until analysis. Enrichment analysis was performed by gas chromatography-mass spectrometry (Agilent 5975; Santa Clara, CA) [Citation26]. Ions of mass to charge ratios of 234, 235, and 239 for phenylalanine and 466, 467, 468, and 470 for tyrosine were utilised. Plasma amino acid analysis was performed by liquid chromatography-electrospray ionisation-mass spectrometry (QTrap 5500 MS; AB Sciex) with Express HT Ultra LC (Eksigent Div.; AB Sciex) after derivatisation with 9-fluoren-9-methoxycarbonyl, and concentrations were measured using the internal standard method [Citation27].
The rate of appearance (Ra) of phenylalanine and tyrosine into the plasma and the rate of phenylalanine hydroxylation to tyrosine was determined from the tracer enrichments in plasma. These parameters allowed calculation of protein synthesis, protein breakdown, and net protein balance using the bioavailability approach as previously validated and described [Citation10,Citation28,Citation29]. Located inside the Clinical Research and Imaging Facility at UAF, we utilised a dual-energy X-ray absorptiometry (GE iDXA; Chicago, IL) scanner for the measurement of body composition, which provided values for fat mass and fat free mass. More specific than BMI, these scans provided similar ratios of fat free mass/total mass from our participants and allowed us to express our data relative to total body mass [Citation29].
Statistical methods
A one-way analysis of variance with repeated measures was used to examine overall differences in plasma essential amino acid concentrations between FR and CB; a Bonferroni post-test was used to detect potential differences in the plasma concentrations of essential amino acids at individual time points. A two-way analysis of variance was utilised to evaluate differences in protein synthesis, protein breakdown, and net protein balance in response to the ingestion of FR or CB. All data were analysed using the GraphPad Prism 6 for Mac (GraphPad Software, Inc. La Jolla CA), and presented as mean ± SD.
Results
Eight participants (40.4 ± 14.0 years of age) with a BMI of 24.0 ± 2.9 kg/m2 were recruited and enrolled in the study (). The participants included two males and five females. Seven participants were Caucasian, and one participant was Alaska Native. One participant dropped out due to a personal matter unrelated to the study. Therefore, the study resulted in seven participants completing all aspects of the study.
Table 2. Clinical characteristics
Plasma concentrations of EAAs and leucine were higher with FR compared to CB at t = 240 and 270 min (P= 0.01) (), respectively).
Figure 2. Plasma concentrations of essential amino acide during the fasted (t = 1–180 min) and Fed state (t = 210–420 min). *Denotes significant difference between FR and CB (P = 0.01). (b) Plasma concentrations of essential leucine during the fasted (t = 1–180 min) and Fed state (t = 210–420 min). *Denotes significant difference between FR and CB (P = 0.01)
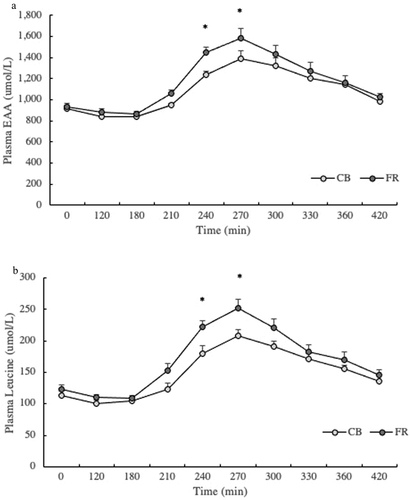
Basal post-absorptive rates of protein synthesis, protein breakdown, and net protein balance were not different in response to equivalents amounts of FR and CB. As previously described in feeding studies using identical stable isotope methodologies [Citation10,Citation26], we have calculated protein synthesis, protein breakdown, and net protein balance relative to their changes from the fasted to the fed state. While there was a similar increase in protein synthesis after FR and CB ingestion, the greater suppression of protein breakdown following FR consumption (P< 0.0001) contributed to a substantially greater enhancement of net protein balance (). We also calculated the rates of protein kinetics relative to the ingested amounts of EAAs and total amino acids. There was a greater reduction in protein breakdown/EAAs ingested in FR compared to CB (P < 0.01), while there were no significant differences between protein synthesis/EAA ingested and net protein balance/EAA ingested (). The suppression in protein breakdown/total amino acids was significantly greater in FR than CB (P< 0.001), contributing to higher net protein balance/amino acids (P< 0.0001) ().
Figure 3. Whole-body protein kinetics: A) changes in rates of whole-body protein synthesis (PS), breakdown (PB), and net balance (NB) from the fasted state; B) changes in rates of PS, breakdown PB, and NB/EAA from the fasted state; and C) changes in rates of PS, PB, and NB/Total Amino Acids from the fasted state. *Denotes significant difference between FR and CB (P < 0.05)
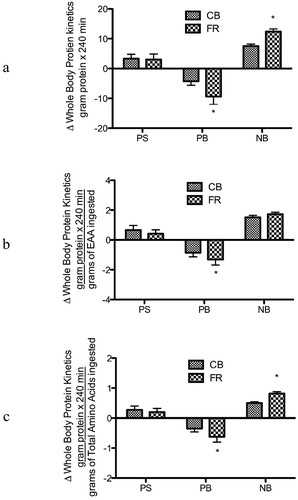
Discussion
The most important observation of this study is that FR resulted in a greater anabolic response, reflected by higher net protein balance, than an equal serving of CB. To our knowledge, this is the first report demonstrating the benefits of free-range red meat analogous to caribou consumed in the traditional, Alaska Native diet, on immediate changes in protein metabolism. Greater net protein balance was linked to higher plasma EAA concentrations stemming from the greater EAA content per ounce-equivalent serving of FR compared to CB. Higher plasma EAA concentrations following ingestion of FR likely contributed to the suppression of protein breakdown. In another recent study, suppression of protein breakdown was even greater in response to consumption of 4 and 11 ounces of red meat [Citation30]. Thus, there appears to be a dose response effect between increases in plasma EAAs and beneficial alterations in protein breakdown and net protein balance.
It is well accepted that EAAs provoke the stimulation of protein synthesis [Citation31], while non-essential amino acids are not primary factors [Citation32]. Leucine is an essential amino acid that operates as a transcriptional programmer or a switch, manipulating signalling pathways that set the stage for protein anabolism [Citation33]. Plasma EAAs and leucine were significantly higher in FR compared to CB shortly after meat ingestion. Taken together, this suggests a more permissive molecular environment in conjunction with greater availability of EAAs needed for optimal feeding-induced increments in net protein balance. Significant correlations have been reported between overall dietary protein and net protein balance (R2 = 0.765; P< 0.0001) [Citation34]. This correlation suggests that non-essential amino acids and overall amino acid intake may play a role in the suppression of protein breakdown. Our assertion is further supported by the consensus that the delivery of 0.24 g of amino acids/kg/meal in 2 oz of FR equates to the maximal response of protein synthesis relative to the overall influence on net protein balance [Citation35]. While isolating the individual contributions of each non-essential amino acid is beyond the scope of the current investigation, the ingestion of arginine, glutamine, glutamate, proline, and glycine have all been implicated in the preservation of lean tissue [Citation9]. Recommendations for amino acid take (essential and non-essential) should also consider variations in growth rates, reproductive status, and ageing [Citation36], and that the “indispensable” characterisation of non-essential amino acids may represent an oversimplification [Citation37].
In addition to the importance of amino acids in the modulation of protein kinetics, the concentration of omega-3 fatty acids is higher in wild, free-range red meat than in CB. While the beneficial influence of omega-3 fatty acids on protein metabolism has gained some attention [Citation38], small increments in the acute ingestion of omega-3 fatty acids would not be likely to influence protein metabolism [Citation39], Nonetheless, consistent consumption of FR or another wild, free-range red meats may offer even greater benefits with regard to lean tissue preservation. Many of the advantages of omega-3 fatty acids are derived from potential improvements in the sensitivity of mammalian target of rapamycin (mTOR) and downstream signalling targets that positively affect protein synthesis and therefore muscle preservation over time [Citation40]. Substantial evidence suggests that omega-3 fatty acids may also mitigate protein breakdown [Citation39], but longitudinal supplementation is necessary for anabolic benefits [Citation38], supporting the beneficial impact of consumption of wild, free-range red meat over the course of a lifetime.
The more anabolic profiles of EAAs and omega-3 fatty acids in FR were accompanied by lower calories compared to CB of an equivalent serving size. The onset of muscle atrophy during ageing is typically accompanied by increased deposition of adipose tissue, creating a condition referred to as sarcopenic obesity [Citation41]. This decrease in muscle mass combined with an increase in fat compounds the detrimental effects of sarcopenic-related issues such as diabetes, heart disease, decreases in mobility and independence, and increases in depression [Citation42]. Even with 40% fewer calories in an equivalent serving of CB, FR promoted a ~ 50% greater feeding-induced increment in whole body net protein balance. Therefore, consumption of wild, free-range game may improve whole body net protein balance to counteract sarcopenia while reducing the obligatory calories that would otherwise increase the risk of obesity.
Consumption of wild, free-range game may be a foreign concept to the general population, but for many Indigenous communities, it represents a generational nutritional history, as well as cultural and spiritual ancestry [Citation43]. However, in recent years, there has been a transition from traditional foods such as wild, free-range red meat to more processed and commercial meats [Citation44,Citation45]. Combined with historic and contemporary social determinates in many Indigenous populations, which are linked to health disparities, this food migration has increased the risk of chronic disease in Alaska Native peoples [Citation46,Citation47]. Immediate health benefits of wild, free-range red meats can influence the continuation of and/or return to traditional food pathways, which also provides the healthy benefit of increased physical activity [Citation48].
Positive health benefits of wild, free-range red meat may also have a positive influence on the public perception of the hunter/gatherer lifestyle [Citation49], and create niche markets for wild, free-range game [Citation50] for those individuals who appreciate the value of the resource but lack adequate access. That being said, higher amounts of EAAs/total weight in FR or higher protein quality were largely influenced by the consumption of wild forage which has also been demonstrated in grass-fed or organic cattle [Citation19]. Unfortunately, access to “grass-fed” beef and other sources of high-quality proteins my be cost-prohibitive or impossible to access for many individuals [Citation51]. A better understanding of how wild, free-range red meats affect muscle maintenance will inform consumer choices related to either hunting wild game or purchasing CB.
Additionally, our data could positively leverage the health and monetary values of wild, free-range red meats and the rights of individuals who hunt by choice or necessity. Environmental efforts to protect the land and game resource may also use this information to increase support for conservation. The value proposition provided by FR may now better inform governmental, public, and healthcare policies specific to those who choose to provide a superior, wild and free-range meat protein source to their families.
Lifestyle interventions are often used as a preventative strategy against the development of sarcopenia, related chronic conditions, and costs of care. However, the World Health Organization 3maintains that adherence to such structured interventions is especially difficult for elderly people [Citation52]. This is particularly true for those who may already be experiencing declines in health or living in deprived and/or secluded areas such as remote areas of Alaska [Citation53,Citation54]. As part of a more comprehensive perspective, preventative nutritional approaches at younger ages have been implemented to combat disability and optimise health throughout a lifetime in countries such as Japan, known for exceptional health and longevity [Citation55,Citation56]. Similarly, in epidemiological studies of Arctic Indigenous populations, nutritional consumption of traditional, wild game/fish have demonstrated a positive association with improved health [Citation57]
Comparisons among individuals with various stages of metabolic abnormalities were beyond the scope of our study and prevent us from comparing individuals based on body weight, ethnicity, and sex. Nevertheless, wild, free-range game, with a direct benefit to muscle preservation, provides a value proposition that could potentially influence laws and regulations surrounding the protection of the environment, as well as human rights to obtain that source of nutritional sustenance. It is our intention to utilise the results of this investigation as a platform upon which we will base future grants for research focused on the health benefits of other traditional sources of foods for Indigenous populations, particularly Alaska Native peoples.
Conclusion
We have demonstrated that the acute beneficial influence of FR on net protein balance exceeds that of CB. Our study results support the rationale that wild, free-range red meats may offer a greater nutritional value by weight, given the administration of equivalent portion sizes most often used by consumers [Citation58]. These results have valuable implications for societies that rely heavily on wild, free-range red meats for nutritive value, subsistence, and health of heritage.
Statement of author contributions
Author contributions: M.S.C., N.E.P.D., R.R.W., R.H.C., conceived and designed study; M.S.C., S.E.S., R.R.W., R.H.C., performed experiments; M.S.C., S.E.S., R.H.C., analyzed data; M.S.C., S.P., R.H.W., N.E.P.D., R.R.W., R.H.C., interpreted results of experiments; M.S.C., S.P., R.H.W., A.A.F., N.E.P.D., R.R.W., R.H.C., prepared tables and figures; M.S.C., S.P., R.H.C. drafted manuscript; M.S.C., R.H.C., edited and revised manuscript; M.S.C., S.E.S., S.P., R.H.W., N.E.P.D., R.R.W., R.H.C., approved final version of manuscript: M.S.C., S.E.S., S.P., R.H.W., A.A.F., N.E.P.D., R.R.W., R.H.C.
Acknowledgments
wish to thank graduate committee members, Drs. Todd Brinkman, Josh Greenberg, Mark Lindberg, Lawrence Duffy, and faculty advisor Barbara Taylor for their guidance and direction on this project. We also recognize Tiffany Aguilar for processing of blood samples and Gwendolyn Quigley and Kaylee Ladd for their organizational contributions. We would like to express our sincere appreciation to our dedicated participants from the Fairbanks, Alaska area that volunteered their time and effort for this study.
Disclosure statement
No potential conflict of interest was reported by the authors.
Additional information
Funding
References
- Burge R, Dawson-Hughes B, Solomon DH, et al. Incidence and economic burden of osteoporosis-related fractures in the USA, 2005-2025. J Bone Miner Res. 2007;22(3):465–10.
- Goins RT, Moss M, Buchwald D, et al. Disability among older American Indians and Alaska Natives: an analysis of the 2000 Census Public Use Microdata Sample. Geronotologist. 2007;47:690–696.
- Schure MB, Goins RT. An examination of the disablement process among older American Indians: the native elder care study. Gerontologist. 2016;56(5):948–955.
- Beaudart C, Rizzoli R, Bruyère O, et al. Sarcopenia:Burdenand challenges for public health. Arch Public Heal. 2014;72(45). DOI: https://doi.org/10.1186/20493258-72-45
- Grazko J (2017). Alaska medical cost are more than double the national average. Premera Voices, Premara Blue Cross. [cited 2021 Jan 9]. Available from: https://www.premera.com/Premera-Voices/All-Posts/Alaska-medical-costs-are-more-than-double-the-national-average/
- Lim HS, Park YH, Suh K, et al. Association between sarcopenia, sarcopenic obesity, and chronic disease in Korean elderly. J Bone Metab. 2018;25(3):187–193.
- Deer RR, Volpi E. Protein intake and muscle function in older adults. Curr Opin Clin Nutr Metab Care. 2015;18(3):248–253.
- Wolfe RR. Branched-chain amino acids and muscle protein synthesis in humans: myth or reality. J Int Soc Sports Nutr. 2017;14:30.
- Hou Y, Yin Y, Wu G. Dietary essentiality of “nutritionally non-essential amino acids” for animals and humans. Exp Biol Med. 2015;240(8):997–1007.
- Coker RH, Shin K, Scholten K, et al. Essential amino acid-enriched meal replacement promotes superior net protein balance in older, overweight adults. Clin Nutr. 2019;38(6):2821–2826.
- Strazdiņa V, Jemeļjanovs A, Sterna V (2013). Nutrition value of wild animal meat. Proceedings of the Latvian Academy of Sciences. Section B, Natural, Exact and Applied Sciences. 67: 4–5.
- Lester S. The 2013 FAO report on dietary protein quality evaluation in human nutrition: recommendations and implications. Nutr Bull. 2013. DOI:https://doi.org/10.1111/nbu.12063
- Huang S, Wang LM, Sivendiran T, et al. Review: amino acid concentration of high protein food products and an overview of the current methods used to determine protein quality. Crit Rev Food Sci Nutr. 2018;58(15):2673–2678.
- Pham TH, Vidal NP, Manful CF, et al. Moose and caribou as novel sources of functional lipids: fatty acid esters of hydroxy fatty acids, diglycerides and monoacetyldiglycerides. Molecules. 2019;24(2):232.
- Lonnie M, Hooker E, Brunstrom JM, et al. Protein for life: review of optimal protein intake, sustainable dietary sources and the effect on appetite in ageing adults. Nutrients. 2018;10(3):360.
- Moore DR, Philp A. Editorial: nutritional strategies to promote muscle mass and function across the health span. Front Nutr. 2020;7:569270.
- Schap T, Kuczynski K, Hiza H. PRACTICE APPLICATIONS USDA center for nutrition policy and promotion corner. J Acad Nutr Diet. 2017;117:519–521.
- Flagstad O, Røed KN. Refugial origins of reindeer (Rangifer tarandus L.) inferred from mitochondrial DNA sequences. Evolution. 2003;57(3):658–670.
- Patel M, Sonesson U, Hessle A. Upgrading plant amino acids through cattle to improve the nutritional value for humans: effects of different production systems. Animal. 2017;11(3):519–528.
- NutritionData.self.com. Game meat, caribou, cooked, roasted. 2021 [cited 2021 Jan 08]. Available from: https://nutritiondata.self.com/facts/lamb-veal-and-game-products/4632/2
- Finstad GL, Kielland KK, Schneider WS. Reindeer herding in transition: historical and modern day challenges for Alaskan reindeer herders. Nomadic Peoples. 2006;10(2):31–49. .
- Beef. It’s What’s for Dinner. 2019. Available from: https://www.beefitswhatsfordinner.com/retail/sales-datashopper-insights/ground-beef-sales
- Atawia RT, Bunch KL, Toque HA, et al. Mechanisms of obesity-induced metabolic and vascular dysfunctions. Front Biosci (Landmark Ed). 2019;24:890–934.
- Agriculture, U. S. D. of H. and H. S. and U. S. D. of. 2015 – 2020 dietary guidelines for Americans. 2015 – 2020 dietary guidelines for Americans. 8th ed.; 2015. Available at http://health.gov/dietaryguidelines/2015/guidelines/. doi:https://doi.org/10.1097/NT.0b013e31826c50af
- Abumrad NN, Rabin D, Diamond MP, et al. Use of a heated superficial hand vein as an alternative site for the measurement of amino acid concentrations and for the study of glucose and alanine kinetics in man. Metabolism. 1981;30(9):936–940.
- Kim I-Y, Schutzler SE, Schrader A, et al. The anabolic response to a meal containing different amounts of protein is not limited by the maximal stimulation of protein synthesis in healthy young adults. Am J Physiol Endocrinol Metab. 2015a;310(1):E73–E80.
- De Betue CTI, Joosten KFM, Deutz NEP, et al. Arginine appearance and nitric oxide synthesis in critically ill infants can be increased with a protein-energy-enriched enteral formula. Am J Clin Nutr. 2013;98(4):907–916.
- Chinkes DL, Wolfe RR. Isotope tracers in metabolic research: principles and practice of kinetic analysis. Second ed. Wiley; 2005.
- Wolfe RR, Park S, Kim IY, et al. Advances in stable isotope tracer methodology part 2: new thoughts about an “old” method-measurement of whole body protein synthesis and breakdown in the fed state. J Invest Med. 2020;68(1):11–15.
- Kim I-Y, Schutzler SE, Schrader A, et al. Quantity of dietary protein intake, but not pattern of intake, affects net protein balance primarily through differences in protein synthesis in older adults. Am J Physiol Endocrinol Metab. 2015b;308(1):E21–E28.
- Volpi E, Kobayashi H, Sheffield-Moore M, et al. Essential amino acids are primarily responsible for the amino acid stimulation of muscle protein anabolism in healthy elderly adults. Am J Clin Nutr. 2003;78(2):250–258.
- Børsheim E, Tipton KD, Wolf SE, et al. Essential amino acids and muscle protein recovery from resistance exercise. Am J Physiol Endocrinol Metab. 2002;283(4):E648–E657.
- Deane CS, Ely IA, Wikinson DJ, et al. (2020). Dietary protein, exercise, ageing and physical activity: interactive influences on skeletal muscle proteostasis. Proceeding of the Nutrition Society. 1–12. DOI: https://doi.org/10.1017/S0029665120007879
- Kim I-Y, Deutz NEP, Wolfe RR. Update on maximal anabolic response to dietary protein. Clin Nutr. 2018;37(2):411–418.
- Moore DR, Churchward-Venne TA, Witard O, et al. Protein ingestion to stimulate myofibrillar protein synthesis requires greater relative protein intakes in healthy older versus younger men. J Gerontol A. 2015;70(1):57–62.
- Phang JM, Liu W, Hancock C. Bridging epigenetics and metabolism: role of non-essential amino acids. Epigenetics. 2013;8(3):231–236.
- Wu G. Dietary requirements of synthesizable amino acids by animals: a paradigm shift in protein nutrition. J Anim Sci Biotechnol. 2014;5(1):34.
- Di Girolamo FG, Situlin R, Mazzucco S, et al. Omega-3 fatty acids and protein metabolism: enhancement of anabolic interventions for sarcopenia. Curr Opin Clin Nutr Metab Care. 2014;17(2):145–150.
- Magee P, Pearson S, Whittingham-Dowd J, et al. PPARγ as a molecular target of EPA anti-inflammatory activity during TNF-α-impaired skeletal muscle cell differentiation. J Nutr Biochem. 2012;23(11):1440–1448.
- Drummond MJ, Dreyer HC, Fry CS, et al. Nutritional and contractile regulation of human skeletal muscle protein synthesis and mTORC1 signaling. J Appl Physiol. 2009;106(4):1374–1384.
- Morgan PT, Smeuninx B, Breen L. Exploring the impact of obesity on skeletal muscle function in older age. Front Nutr. 2020;7:569904.
- Sanada K, Chen R, Wilcox B, et al. Association of sarcopenic obesity predicted by anthropometric measurements and 24-y all-cause mortality in elderly men: the Kuakini Honululu Heart Program. Nutrition. 2018;46:97–102.
- Nu J, Bersamin A. Collaborating with Alaska Native communities to design a cultural food intervention to address nutrition transition. Prog Community Health Partnerships Res Educ Action. 2017;11(1):71–80.
- Batal M, Johnson-Down L, Moubarac JC, et al. Quantifying associations of the dietary share of ultra-processed foods with overall diet quality in First Nations peoples in the Canadian provinces of British Columbia, Alberta, Manitoba and Ontario. Public Health Nutr. 2018 Jan;21(1):103–113. . Epub 2017 Jul 25. PMID: 28738909.
- Gerlach SC, Loring PA. Rebuilding northern foodsheds, sustainable food systems, community well-being, and food security. Int J Circumpolar Health. 2013;72(1):21560.
- Center for Disease Control and Prevention. 2016. Health promotion and diabetes prevention in American Indian and alaska native communities-traditional foods project, 2008-2014. Available from: https://www.cdc.gov/mmwr/volumes/65/su/su6501a3.htm
- DeBruyn L, Fullerton L, Satterfield D, et al. Integrating culture and history to promote health and help prevent type 2 diabetes in American Indian/Alaska native communities: traditional foods have become a way to talk about health. Prev Chronic Dis. 2020;17:E12.
- Coker MS, Ladd K, Murphy CJ, et al. Alaska backcountry expeditionary hunting promotes rapid improvements in metabolic biomarkers in healthy males and females. Physiol Rep. 2021;9(1):e14682.
- Byrd E, Lee JG, Olynk NJ. Perceptions of hunting and hunters by U.S. respondents. Animals. 2017;7(11):83.
- Marescotti ME, Caputo V, Demartini E, et al. Discovering market segments for hunted wild game meat. Meat Sci. 2019;149:163–176.
- Henchion M, Hayes M, Mullen AM, et al. Future protein supply and demand: strategies and factors influencing a sustainable equilibrium. Foods. 2017;6(7):53.
- Burton LA, Sumukadas D. Optimal management of sarcopenia. Clin Interv Aging. 2010;5:217–228.
- Knechel NA. The challenges of enrolling older adults into intervention studies. Yale J Biol Med. 2013;86(1):41–47.
- Liljas AEM, Walters K, Jovicic A, et al. Strategies to improve engagement of ‘hard to reach’ older people in research on health promotion: a systematic review. BMC Public Health. 2017;17(1):349.
- Arai H, Ouchi Y, Toba K, et al. Japan as the front-runner of super-aged societies: perspectives from medicine and medical care in Japan. Geriatrics Gerontology Int. 2015;15(6):673–687. .
- Gabriel AS, Ninomiya K, Uneyama H. The role of the Japanese traditional diet in healthy and sustainable dietary patterns around the world. Nutrients. 2018;173. DOI:https://doi.org/10.3390/nu10020173.
- Lavigne-Robichaud M, Moubarac JC, Lantagne-Lopez S, et al. Diet quality indices in relation to metabolic syndrome in an Indigenous Cree (Eeyouch) population in northern Québec, Canada. Public Health Nutr. 2018 Jan;21(1):172–180. . Epub 2017 Jul 7. PMID: 28683844.
- Agriculture, U. S. D. of. All about the protein foods group. USDA ChooseMyPlate; n.d.