ABSTRACT
This study evaluated the effect of dietary aflatoxin B1 (AFB1) on growth, milt and egg quality in matured Nile tilapia (Oreochromis niloticus). Triplicate groups of Nile tilapia (initial body weight 24.1 ± 2.6 g) were fed with either of four diets (Diets 1 to 4) designed to contain 0, 20, 200 and 2000 μg AFB1 kg−1 diets for 24 weeks. After 24 weeks of AFB1 exposure, growth was significantly (P <0.05) different between the control and the AFB1 exposed treatments in both sexes. No significant differences were observed in 17β-oestradiol, absolute fecundity, oocytes volume and diameters between AFB1 exposure groups and the control group. However, we observed a significant reduction in relative fecundity and gonad somatic index (GSI) in females fed 2000 μg AFB1 kg−1 diet. On the other hand, we observed significant differences (P <0.05) in gonadosomatic index (GSI), testosterone, milt count and motility between males in the control group and AFB1 treatments. We conclude that rearing Nile tilapia with aflatoxin-contaminated diets for a prolonged period affects milt quality, fecundity (at higher doses) and growth performance. This implies that for optimal seed production, provision of aflatoxin free diets should be part of the management practices in Nile tilapia hatcheries.
1. Introduction
Nile tilapia (Oreochromis niloticus) is East Africa’s most cultured fish species and is farmed under a range of semi-intensive and intensive systems [Citation1]. It is known for rapid growth, tolerance to high stocking densities and poor water quality, high reproductive rates and low susceptibility to disease. However, obtaining good quality locally made feed is the largest constraint for semi-intensive and intensive aquaculture of the species in the region [Citation1]. To lower costs, the use of plant-based ingredients in aquafeeds is increasing. However, plant-based ingredients are very susceptible to aflatoxin contamination at different stages of the agricultural chain such as pre-harvest, harvest and post-harvest handling. Thus, feeds are the possible source of aflatoxin exposure of farmed Nile tilapia [Citation2].
Aflatoxins (AF) are mycotoxins produced mostly by the fungi Aspergillus flavus and A. parasiticus, which grow on numerous feedstuffs when environmental conditions are favourable [Citation3]. There are four common types of aflatoxin–aflatoxins B1, B2, G1 and G2 and among them; AFB1 is the most potent one to human and animals [Citation4]. Aflatoxins are some of the most dangerous mycotoxins that are characterized and famous for their high toxicity to both animals and humans. Aflatoxins contamination has been reported to cause a reduction in fish productivity as well as fish mortality [Citation5]. They cause anaemia, haemorrhaging, liver impairment, decreased weight, increased vulnerability to secondary infectious diseases and increased mortality resulting in significant problems in aquaculture [Citation5].
Aflatoxins can affect reproductive performance directly generating hormonal dysfunction and inducing cell toxicity. When hormonal functions are disrupted in fish, it may affect their sexual maturation, gamete production and transport, sexual behaviour, fertility, gestation, modifications in other functions that are dependent on the integrity of the reproductive system [Citation6].
Aflatoxins have also been reported to reduce egg size and volume in gibel carp and reduce hatchability, egg production and quality in poultry [Citation7–Citation9]. Aflatoxins reduce sperm concentration, and impair survival of spermatozoa in boars, and decrease semen volumes and testis weights in juvenile Japanese quail [Citation10–Citation13]. Egg and milt quality of fish are critical in fertilization success [Citation14]. Also, milt quality is a very important variable in aquaculture broodstock management and influences egg fertilization rates [Citation14]. However, there is limited information about the effects of aflatoxins in egg and milt quality of Nile tilapia reared in ponds.
Many studies have documented the association between aflatoxins and growth, primary liver cancer, haematocrit and humoral immune response in Nile tilapia, but little is known about the relationship between this toxin and egg and milt quality in this species. Here, we investigate the toxic effects of AFB1 on the reproductive hormones, egg and milt quality of Nile tilapia reared in ponds for 24 weeks. Considering that toxic effect of AFB1 depends on the dose in the feeds as well as sex and the age of animal [Citation15], we also examined whether males and females of Nile tilapia exhibit differences in their growth response to dietary AFB1 contamination.
2. Materials and methods
2.1. Experimental system and animals
The experiment was carried out in 12 cages (1 m3 each) placed in a 40 × 20 m pond at the National Aquaculture Research and Development Centre (NARDTC), Sagana, Kenya. Throughout the experiment water quality parameters were maintained as follows: temperature from 24.2°C to 26.8°C; pH from 7.5 to 7.65; dissolved oxygen, 4.6–6.3 mg L−1; total alkalinity, 146.65–225.95 mg L−1; and salinity, 0.03–0.05 ppt.
Nile tilapia fingerlings (24.1 ± 2.6 g) were obtained from NARDTC, Sagana. Fishes were first acclimated to their cages conditions and fed with the control diet for 15 days before dietary treatments.
2.2. Experimental design and feeding trials
A pure crystalline powder of AFB1 was obtained from Libios Chemical Company Ltd, Rue Edmond Michelet, France. The AFB1 (5 mg) was dissolved in 100 mL of pure methanol to form a stock solution containing 0.05 mg AFB1 mL−1 of methanol. Four experimental diets, Diet 1, 2, 3 and 4 were formulated and designed to, respectively, contain 0, 20, 200 and 2000 μg AFB1 kg−1 of feed and the determined levels were 0.68, 22.5, 235.4 and 2046.5 μg AFB1 kg−1, respectively. The formulation of the basal diet is shown in . Diets were made into pellets (5 mm, diameter), oven-dried at 60°C and stored at −20°C until fed. The given toxin levels and control feeds were cross-checked twice a month through Enzyme-Linked Immunosorbent Assay (ELISA) (BioTek Instruments, Inc., VT, USA).
Table 1. Formulation and chemical composition of basal diet (in dry weight).
2.3. Experimental feeding regime
A total of 300 Nile tilapia fingerlings were divided into 12 equal groups of 25 fish and placed in cages. Three replicates of 25 fish each were randomly assigned to each treatment and fed on experimental diets following acclimatization. Fish were fed to apparent satiation twice a day at 0900 and 1600 h. Pellets were slowly fed to the fish to minimize feed wastage. The trial lasted for 24 weeks. At the end of the experiment, fish from each cage was sampled randomly after 1 day of food deprivation.
2.4. Growth performance
Each month, all the fish in each experimental unit were sampled and measured to determine number, weight and length. At the end of the experiment, 18 fish (9 males and 9 females) from each dietary treatment were sampled randomly after a day of food deprivation. Average weight gain (AWG), average daily gain (AVG) and survival rate (SR%) were calculated according to Tola et al. [Citation16].
2.5. Fecundity
The females were weighed and dissected to remove ovaries which were also weighed. Gonadosomatic index (GSI) was calculated as ovary weight divided by body weight multiplied by 100. Egg numbers in the ovaries were counted to determine absolute fecundity (AF) and relative fecundity (RF) was calculated by dividing the total egg number by total body weight [Citation17]. Egg weight (dry and wet basis) was determined using 50-count egg samples: a sample of 50 eggs was weighed and oven-dried at 70°C for 48 h. Eggs diameter was measured using a microscope eye-piece graticule for length (L) and width (H). Egg volume was calculated by the formula: V = π LH2/6 [Citation18], where: V = egg volume (mm3), L = long axis (mm), H = short axis (mm), π = 3.14.
2.6. Milt quality
At the end of the experiment, males were selected randomly, weighed and dissected, testes removed and gonad somatic index (GSI) determined. Milt samples from males were taken by hand stripping. Milt motility was estimated by microscopic observation of milt diluted 100-fold in a distilled water solution. Forward motility was assessed according to Tekin [Citation19]. Milt volume was determined by measuring with a pipette and expressed in ml. Spermatozoa concentration was determined using haemocytometer and expressed as number of cells x 109 cell ml−1 [Citation19].
2.7. Sex-steroid hormone measurements
The sex hormones (testosterone and 17β-oestradiol) were analysed using Enzyme-Linked Immunosorbent Assay (ELISA) (BioTek Instruments, Inc., VT, USA). This was done using commercially available ELISA kits from Human Chemical Company, Germany according to supplier’s instructions. (Intra-assay coefficients of variation were 3.5% and 3.8% while inter-assay coefficients of variation were 11.8% and 9.8% for testosterone and 17β-oestradiol, respectively.
2.8. Data analysis
Data analyses were performed using a two-way ANOVA (randomized block) (Genstat 4.0) that examined the main effects of AFB1 contamination, duration and their interactions and means were tested by Bonferroni test at the 5% level of significance using GenStat 4.0. For the effect of AFB1 contamination in sex hormones, egg and milt quality, one-way ANOVA followed by Bonferroni test at the 5% level of significance procedure was followed using GenStat 4.0.
3. Results
3.1. Growth performance
Aflatoxin significantly (P <0.05) depressed growth rate, average weight gain and average daily gain in both sexes (). Mean final weight was higher in control group of female Nile tilapia (91.67 g) compared to exposure groups (79.16, 68.86, 59.25 gfor diet 2, 3 and 4, respectively). Mean final weight of male Nile tilapia was also affected by AFB1 whereas the final mean weight of exposure groups was lower than that of control group (). Aflatoxin adversely affected average weight gain (P <0.05) in both sexes. AWG was decreased with increased AFB1 concentrations. As shown in , the AWG of male Nile tilapia is lower than that of female Nile tilapia.
Table 2. Final body weight and survival rate of male and female Nile tilapia fed with different dietary AFB1 for 24 weeks.
The effect of AFB1 on growth rate depended on the duration of exposure whereas, in the first 2 months, there were no differences in growth between groups with different AFB1 levels but by 3 months the weight gain of fish exposed to 200 and 2000 μg AFB1 kg−1 diets were lower than that of the control and 20 μg AFB1 kg−1 diet ().
Figure 1. Bodyweight change of Nile tilapia fed with different dietary AFB1 for a duration of 24 weeks.
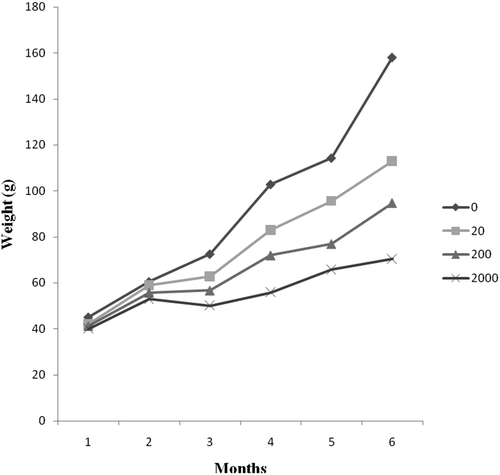
There was no significant difference in survival rate between the sexes. Survival rate was 99–100% for both male and female Nile tilapia and fish appeared healthy ().
3.2. Effect of AFB1 on reproductive hormones, egg and milt quality of Nile tilapia
Effects of aflatoxins on fecundity, egg and milt quality and sex hormones of Nile are shown in . There was no significant difference (P <0.05) in absolute fecundity between the control group and exposure groups. The absolute fecundity of Nile tilapia fed in control group was 435 g decreasing to 431 g in diet 4. Relative fecundity ranged from 308 ± 2.06, 310 ± 2.05, 314 ± 2.28, 550 ± 3.80 number of eggs per body weight (g) for fish fed diets 1, 2, 3 and 4, respectively. A significant difference (P <0.05) occurred in relative fecundity between control group and diet 4.
Table 3. Effects of aflatoxin on fecundity, egg and milt quality of Nile tilapia fed with different dietary AFB1 for 24 weeks (mean ± SE).
Oocyte diameter ranged from 2.80 mm in control group to 2.70 mm in diet 4, however, no significant difference was observed between control and AFB1 supplemented diets.
Egg volume ranged from 5.78, 5.72, 5.69 and 5.67 mm3 for diet 1, 2, 3 and 4, respectively, and there were no significant differences (P <0.05) between control group and AFB1 supplemented group. There was a decrease in total egg volume/ovary on fish exposed to AFB1 diet. Also, there were no significant differences (P <0.05) in egg dry weight between the control group and exposure groups.
Gonad somatic index (GSI) for female Nile tilapia values amounted 3.30% ± 0.01, 3.278% ± 0.02, 3.26% ± 0.12, 2.82% ± 0.37 for control, diet 1, diet 2, diet 3 and diet 4 respectively. There were significant differences in GSI between control group and diet 4.
Gonad somatic index (GSI) for male Nile tilapia was significantly higher in control group (1.88% ± 0.21) compared to diet 2, 3 and 4 (1.86% ± 0.28, 1.69% ± 0.17% and 0.96% ± 0.03%, respectively). The mean milt concentration among males varied from 10.4 × 109 to 7.5 × 109 sp ml−1 among the diets. The highest level of milt concentration was recorded in control group and lowest in diet 4. However, among the males having milt, mean individual milt volume did not reveal any significant differences between the diets (P >0.05), . Results from analysis of variance (ANOVA) showed that milt motility, milt concentration and gonad somatic index (GSI) were significantly lower in fish fed with AFB1 supplemented diet ().
A slight decrease in the blood serum oestradiol-17β levels were observed (3.40 ng ml−1 for control group and 3.19 ng ml−1 for diet 4), . However, no significant differences (P > 0.05) were observed among the four groups studied regarding blood serum oestradiol-17β in female Nile tilapia. The control group showed significantly higher concentration of testosterone in male Nile tilapia (2.06 ng ml−1) when compared with diet 4 (0.29 ng ml−1).
4. Discussion
4.1. Growth performance
The present study revealed a significant decrease in growth performances in Nile tilapia after 24 weeks period of AFB1 treatment. The highest growth performance was recorded in fish fed on control diet compared with the AFB1 supplemented diet. These results indicate that growth performance was significantly affected by AFB1. In general, during the 24 weeks investigation period, growth rate decreased with increased AFB1 concentrations. This is in line with Deng et al. [Citation15]; El-Banna et al. [Citation20]; Cagauan and Tayaban [Citation21] who found that AFB1 exposure affected feed intake and growth performance in fish. The significant decrease in weight gain in treated groups suggests that normal digestive and nutrient absorptive functions of the intestinal epithelium were affected [Citation22]. Deng et al. [Citation15] and Mahfouz [Citation23] reported that negative effects of AFB1 on growth could be observed only after long periods of feeding with the toxin. Similarly, in the present study, we found no difference in growth between the control group and groups with different AFB1 levels in the first 2 months, but by the 3 months, the weight of fish exposed to 200 and 2000 μg AFB1 kg−1 diets was lower than that of the control and 20 μg AFB1 kg−1 diets. Other studies found out that AFB1 did not affect growth on other fish species like Huso huso and common carp after being exposed with 100 and 2000 μg AFB1 kg−1 diets for 12 and 24 weeks, respectively [Citation9,Citation24]. This indicates that the effect of AFB1 on growth performance in fish is dependent on species, dose and duration.
We also observed lower mean weight of male Nile tilapia compared to females in all AFB1 supplemented diet. Female Nile tilapia fed with 2000 μg AFB1 kg−1 diet had mean body weight of 59.25 g while male Nile tilapia had 55.78 g body weight. Although, data analysis indicated no significant difference in response to AFB1 (P >0.05) on the mean body weight between male and female Nile tilapia, considering that males normally grow larger than females, this small reduction is nevertheless worth noting. On the other hand, there was a significant sex difference in response to aflatoxin B1 (P <0.05) on the AWG in the current study. Similar results were found in studies of other animal species. For example growth rates for males of pigs, birds and rats were more affected by aflatoxins exposure than their female counterparts [Citation25–Citation28]. More studies exposing Nile tilapia with higher concentrations of aflatoxins beyond the highest doses in the present study may determine whether male and female tilapia responds differently in growth performance and feed intake. In a previous study [Citation29], we found aflatoxins contamination levels of up to 806 µg kg−1 in fish feeds and ingredients. This suggests a more serious problem that may cause low yields and economic losses in the region. Hence, controlling aflatoxin contamination throughout the fish feed value chain is necessary.
In the present study, survival rates did not differ significantly between Nile tilapia fed the control and diets containing AFB1 and were generally high (99–100%) for both males and females. These results are similar with Deng et al. [Citation15]; Usanno et al. [Citation30]; Chávez-Sánchez et al. [Citation31] who found out that AFB1 does not induce mortality in tilapia fed with a diet containing more than 1000 µg kg−1 AFB1. In other studies, Tuan et al. [Citation32] and Chávez-Sánchez et al. [Citation31] reported that diets containing 10 and 30 mg AFB1 kg−1 respectively did not lead to mortality in Nile tilapia and concluded that tilapia can sustain a higher dose of dietary AFB1.
4.2. Effect of AFB1 on reproductive hormones, egg and milt quality of Nile tilapia
The most sensitive test for spermatogenesis is sperm count and it is highly correlated with fertility [Citation33]. In fish, milt count is an important variable in influencing the fertilization of eggs. The present study found mean milt concentration varying from 10.4 × 109 to 7.5 × 109 sp ml−1 among the diets. The highest level of milt concentration was recorded in the control group and was lowest in 2000 μg AFB1 kg−1 diets. However, milt volume showed no significant differences between the diets (P > 0.05). Similar results have been reported in other animal species like Japanese quail [Citation12], mouse [Citation34] and rams [Citation35]. The mechanism behind the effects of AFB1 on sperm count and motility in fish is not yet known but it has been described in other animal species [Citation22,Citation36]. Verma and Nair [Citation37] reported that reduced succinic dehydrogenase and ATPase activity could explain the reduced sperm count and motility and increases the number of non-viable spermatozoa observed in aflatoxin-treated mice. In the present study, we monitored neither succinic dehydrogenase nor ATPase activity. However, results of the present study suggest that feeding male Nile tilapia with AFB1 supplemented diet for a long duration affects their milt count and may be acting in a similar manner to other animals.
The male control group showed a significantly higher concentration of testosterone (2.06 ng ml−1) when compared with 2000 μg AFB1 kg−1 diets (0.29 ng ml−1). Similar results were reported in other animal species by Bashandy et al. [Citation7]; Kosutzka et al. [Citation38]; Ortatatli et al. [Citation39]; Piskac el al. [Citation40] who found lowered plasma testosterone concentrations in rats, quail and roosters fed on AFB1. Decrease in testosterone levels may result in growth impairment because it has an anabolic effect on protein synthesis [Citation36,Citation41]. The significant decrease in the testosterone levels in the present study may be attributed to the reduction of Leydig cells and the degeneration of sertoli cells due to the presence of AFB1 in the diet. It is suggested by the previous study of Bbosa et al. [Citation42] that the binding of DNA to form complexes and inhibit nucleic acid synthesis is one of the most common mechanisms for the action of AFB1. This mode of action may explain the direct effect of AFB1 on Leydig cells and Sertoli cells in the testes, and thus, the reduction of the testosterone [Citation42,Citation43].
Contrary to the study of Huang et al. [Citation9] who found a reduction in absolute fecundity, egg size and volume in gibel carp fed AFB1 contaminated feed at 2 mg AFB1 kg−1, no significant differences in absolute fecundity, egg volume, egg dry weight and serum oestradiol-17β concentrations were found in the present study. This suggests the presence of genetic variation in sensitivity to aflatoxin among different farmed fish species which could be genetic. Further studies on intraspecies variation in sensitivity to aflatoxin would enable us to determine whether the trait is heritable and whether it can be reduced through selective breeding. This may be likely because of the physiological mechanism through which the effects of aflatoxin have been shown to operate in other species. For example in poultry, AFB1 significantly decreased egg production, egg volume and serum oestradiol-17β concentrations [Citation44–Citation46]. In another study of Washburn et al. [Citation47] found out that AFB1 caused a reduction in egg size and increase in eggshell percentage, which was attributed by the fact that aflatoxins are metabolized in the liver, where the synthesis and transport of the precursors required to yolk production occur.
In this study, no significant decrease in egg size and oestradiol-17β concentrations was observed between control and exposed group. However, the result shows a trend towards decreasing levels of egg size and oestradiol-17β concentrations with increase in AFB1 concentrations in the diet.
In addition, in the present study, a significant decrease in GSI was observed only in higher‐doses (2000 μg AFB1 kg−1) in female Nile tilapia. On the other hand, in male Nile tilapia a significant decrease in GSI was observed in 200 and 2000 μg AFB1 kg−1 diets suggesting sex-linked variation in levels of sensitivity among Nile tilapia.
5. Conclusion
Prolonged feeding of Nile tilapia with high levels of AFB1 for 24 weeks reduced body weight of Nile tilapia in the present study. Males of Nile tilapia were more susceptible to dietary exposure of AFB1 than their female counterparts as exhibited by the effects on different reproductive parameters. On the contrary, absolute fecundity, egg weight and size, oocyte diameters and oestradiol-17β of female Nile tilapia were not affected by AFB1. This indicates the necessity of improved management practices during preparation and handling of fish feeds as this may affect pond yields and production of fish seed in hatcheries. Studies on the existence of genetic variation in sensitivity to aflatoxin within species may point to possibility for control of aflatoxin effects through genetic means.
Acknowledgments
This research was supported by the Organization for Women in Science for Developing World (OWSD) and Tanzania commission for science (COSTECH). Harrison Charo-Karisa was supported by the CGIAR Research Program on Fish Agri-Food Systems (FISH CRP). The authors are grateful to the National Aquaculture Research and Development Centre (NARDTC), Sagana, Kenya for the provision of ponds, cages and fingerlings. In addition, we gratefully acknowledge the assistance of Mr Hesbon Odongo of Physiology laboratory, Ann Owiti of Biotechnology Centre, Peter Thimbu of Parasitology laboratory and Francis Okumu of Histology laboratory at the University of Nairobi. Mr Peter Miruka, Ms Sally Mwarumba, Ms Florence Mbugua, Mr Elijah Gichana and Mr Nathan Chuma in the field and laboratory work at NARDTC.
Disclosure statement
No potential conflict of interest was reported by the authors.
References
- Liti D, Cherop L, Munguti J, et al. Growth and economic performance of Nile tilapia (Oreochromis niloticus L.) fed on two formulated diets and two locally available feeds in fertilized ponds. Aquac Res. 2005;36:746–752.
- Spring P, Fegan DF, Lyons TP, et al. Mycotoxins - a rising threat to aquaculture. Nutr. Biotechnol. Feed food Ind. Proc. Alltech’s 21st Annu. Symp; Lexington, Kentucky, USA: Alltech UK; 2005. p. 323–331.
- Diaz-Guerra TM, Mellado E, Cuenca-Estrella M, et al. Genetic similarity among one Aspergillus flavus strain isolated from a patient who underwent heart surgery and two environmental strains obtained from the operating room. J Clin Microbiol. 2000;38:2419–2422.
- Okoth S. Improving the evidence base on aflatoxin contamination and exposure in Africa. Nairobi, Kenya: The Technical Centre for Agricultural and Rural Cooperation (CTA); 2016. p. 1–128.
- Santacroce MP, Conversano MC, Casalino E, et al. Aflatoxins in aquatic species: metabolism, toxicity and perspectives. Rev Fish Biol Fish. 2008;18:99–130.
- Arukwe A. Cellular and molecular responses to endocrine-modulators and the impact on fish reproduction. Mar Pollut Bull. 2001;42:643–655.
- Bashandy S, Galil M, Bashandy M, et al. Effect of dietary aflatoxin and vitamin C on gonadal activity in male rats. Al-Azhar Bull Sci. 1994;5:697–707.
- Zaghini A, Martelli G, Roncada P, et al. Mannanoligosaccharides and aflatoxin B1 in feed for laying hens: effects on egg quality, aflatoxins B1 and M1 residues in eggs, and aflatoxin B1 levels in liver. Poult Sci. 2005;84:825–832.
- Huang Y, Han D, Xiao X, et al. Effect of dietary aflatoxin B1 on growth, fecundity and tissue accumulation in gibel carp during the stage of gonad development. Aquaculture. 2014;428–429:236–242.
- Maryamma KI, Sivadas CG. Aflatoxicosis in goats (an experimental study). Indian Vet J. 1975;52:385–392.
- Pícha J, Cerovský J, Píchová D. Fluctuation in the concentration of sex steroids and aflatoxin B1 in the seminal plasma of boars and its relation to sperm production. Vet Med (Praha). 1986;31:347–357.
- Doerr JA, Ottinger MA. Delayed reproductive development resulting from aflatoxicosis in juvenile Japanese quail. Poult Sci. 1980;59:1995–2001.
- JS S, Howarth B Jr, RD W. Effect of dietary aflatoxin on reproductive performance of mature White Leghorn males. Poult Sci. 1980;59:1311–1315.
- Bobe J, Labbé C. Egg and sperm quality in fish. Gen Comp Endocrinol. 2010;165:535–548.
- Deng SX, Tian LX, Liu FJ, et al. Toxic effects and residue of aflatoxin B1 in tilapia (Oreochromis niloticus x O. aureus) during long-term dietary exposure. Aquaculture. 2010;307:233–240.
- Tola S, Bureau DP, Hooft JM, et al. Effects of wheat naturally contaminated with Fusarium mycotoxins on growth performance and selected health indices of red tilapia (Oreochromis niloticus × O. mossambicus). Toxins (Basel). 2015;7:1929–1944.
- Munkittrick KR, Dixon DG. Growth, fecundity, and energy stores of white sucker (Catostomus commersoni) from lakes containing elevated levels of Copper and Zinc. Can J Fish Aquat Sci. 1988;45:1355–1365.
- Rana K. Reproductive biology and the hatchery rearing of tilapia eggs and fry. In: Muir JF, Roberts RJ, editors. Dordrecht: Springer Netherlands (NL);1988. p. 343–406. DOI: 10.1007/978-94-011-9743-4
- Tekin N, Secer S, Akcay E, et al. The effect of age on spermatological properties in rainbow trout (Oncorhynchus mykiss W., 1792). Turkish J Vet Anim Sci. 2003;27:37–44.
- El-Banna R, Teleb HM, Hadi MM, et al. Performance and tissue residue of tilapias fed dietary aflatoxin. Vet Med J. 1992;40:17–23.
- Cagauan A, Tayaban R. Effect of aflatoxin-contaminated feeds in Nile tilapia (Oreochromis niloticus L.). In: Abstract of the 6th International Symposium on Tilapia in Aquaculture (ISTA 6) Section: health management and diseases; Manila: Philippines; 2004. p. 172–178.
- Verma J, Johri TS, Swain BK. Effect of aflatoxin, ochratoxin and their combination on protein and energy utilisation in white leghorn laying hens. J Sci Food Agric. 2007;87:760–764.
- Mahfouz ME, Sherif AH. A multiparameter investigation into adverse effects of aflatoxin on Oreochromis niloticus health status. J Basic Appl Zool. 2015;71:48–59.
- Sepahdari A, Ebrahimzadeh Mosavi HA, Sharifpour I, et al. Effects of different dietary levels of AFB1 on survival rate and growth factors of Beluga (Huso huso). Iran J Fish Sci. 2010;9:141–150.
- Bryden WL, Cumming RB, Lloyd AB. Sex and strain responses to aflatoxin B1 in the chicken. Avian Pathol. 1980;9:539–550.
- Andretta I, Kipper M, Lehnen C, et al. Meta-analytical study of productive and nutritional interactions of mycotoxins in growing pigs. Animal. 2012;6:1476–1482.
- Castegnaro M, Mohr U, Pfuhl-Leszkowicz A, et al. Sex- and strain-specific induction of renal tumors by ochratoxin A in rats correlates with DNA adduction. Int J Cancer. 1998;77:70–75.
- Gurtoo HL, Motycka L. Effect of sex difference on the in vitro and in vivo metabolism of aflatoxin B1 by the rat. Cancer Res. 1976;36:4663–4671.
- Marijani E, Wainaina JM, Charo-Karisa H, et al. Mycoflora and mycotoxins in finished fish feed and feed ingredients from smallholder farms in East Africa. Egypt J Aquat Res. 2017;43:169–176.
- Usanno O, Chaisilapasung S, Sukrakanchana N, et al. Effects of aflatoxin B1 on sex reversed red tilapia. J Sci Technol. 2005;27:187–197.
- Chavezsanchez MC, Palacios CAM, Moreno IO. Pathological effects of feeding young Oreochromis niloticus diets supplemented with different levels of Aflatoxin B1. Aquaculture. 1994;127:49–60.
- Tuan NA, Manning BB, Lovell RT, et al. Responses of Nile tilapia (Oreochromis niloticus) fed diets containing different concentrations of moniliformin or fumonisin B1. Aquaculture. 2003;217:515–528.
- El-Kashoury AA, Salama AF, Selim AI, et al. Chronic exposure of dicofol promotes reproductive toxicity in male rats. Life Sci J. 2010;7:5–19.
- Agnes VF, Akbarsha MA. Spermatotoxic effect of aflatoxin B1 in the albino mouse. Food Chem Toxicol. 2003;41:119–130.
- Ataman MB, Dönmez HH, Dönmez N, et al. Protective effect of esterified glucomannan on aflatoxin-induced changes in testicular function, sperm quality, and seminal plasma biochemistry in rams. Theriogenology. 2014;81:373–380.
- Clarke RN, Doerr JA, Ottinger MA. Age-related changes in testicular development and reproductive endocrinology associated with aflatoxicosis in the male chicken. Biol Reprod. 1987;36:117–124.
- Verma RJ, Nair A. Ameliorative effect of vitamin E on aflatoxin-induced lipid peroxidation in the testis of mice. Asian J Androl. 2001;3:217–221.
- Kosutzka E, Kosutzky J, Zeman M. Effect of aflatoxin B1 on the reproductive and metabolic functions of Japanese quail (Coturnix coturnix japonica). Hydin Ved Pr Vysk. 1988;23:95–104.
- Ortatatli M, Ciftci MK, Tuzcu M, et al. The effects of aflatoxin on the reproductive system of roosters. Res Vet Sci. 2002;72:29–36.
- Piskac A, Drábek J, Halouzka R, et al. The effect of long term administration of aflatoxins on the health status of male rats and pigs with respect to morphological changes in the testes. Vet Med. 1982;27:101–111.
- Clarke RN, Doerr JA, Ottinger MA. Relative importance of dietary aflatoxin and feed restriction on reproductive changes associated with aflatoxicosis in the maturing White Leghorn male. Poult Sci. 1986;65:2239–2245.
- Bbosa GS, Kitya D, Odda J, et al. Aflatoxins metabolism, effects on epigenetic mechanisms and their role in carcinogenesis. Health (Irvine Calif). 2013;05:14–34.
- Ewuola E, Bello JOA, Jimoh O., et al. Haematological response of growing West African dwarf goats to micro doses of dietary Aflatoxins. Mycotoxicology. 2014;1:9–14.
- Pandey I, Chauhan SS. Studies on production performance and toxin residues in tissues and eggs of layer chickens fed on diets with various concentrations of aflatoxin AFB1. Br Poult Sci. 2007;48:713–723.
- Kim JG, Lee YW, Kim PG, et al. Reduction of aflatoxins by Korean soybean paste and its effect on cytotoxicity and reproductive toxicity–part 3. Inhibitory effects of Korean soybean paste (doen-jang) on aflatoxin toxicity in laying hens and aflatoxin accumulation in their eggs. J Food Prot. 2003;66:866–873.
- Verma J, Swain BK, Johri TS. Effect of various levels of aflatoxin and ochratoxin A and combinations thereof on protein and energy utilisation in broilers. J Sci Food Agric. 2002;82:1412–1417.
- Washburn KW, Wyatt RD, Potts PL, et al. Effects and mechanism of aflatoxin on variation in shell strength. Poult Sci. 1985;64:1302–1305.