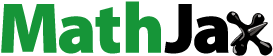
ABSTRACT
The main goal of this work is to fabricate a biocomposite material of hydroxyapatite (HA) and polylactic acid (PLA) loaded with different concentrations of curcumin for using in bone tissue scaffold engineering. PLA-HA-curcumin biocomposite was prepared via the precipitation method with PLA/HA ratio of 80/20 wt% and different ratios of curcumin. The structure and surface morphology of the prepared biocomposite were studied using Fourier Transform Infrared spectroscopy (FTIR), x-ray diffraction spectroscopy (XRD), transmission electron microscope (TEM) and scanning electron microscope (SEM). The bioactivity enhancement of the prepared HA-PLA biocomposite was examined after immersion in simulated body fluid (SBF) before and after addition of curcumin. Drug release of the HA-PLA biocomposite prepared with different concentrations of curcumin was studied in phosphate buffer solution. Our data confirm the nanostructure of the prepared HA-PLA biocomposite and show good miscibility and diffusion of curcumin inside the scaffold matrix. Furthermore, curcumin supplementation effectively enhanced the bioactivity of the HA-PLA biocomposite.
KEYWORDS:
Introduction
Bone replacement and repair are of the most successful applications of tissue engineering. The biodegradable porous scaffold materials act as three-dimensional templates for cell adhesion and subsequent bone regeneration [Citation1,Citation2]. Hydroxyapatite (HA) is a common ceramic bioactive and biocompatible material, which can be used to repair damaged calcified tissues in the human body. Hence, HA is used as a scaffold in human bone replacement [Citation3]. However, HA cannot be directly used as a healing supplementary implant in defected bones due to its low mechanical properties. In order to enhance the regeneration of bone tissue, it is important to synthesis materials with appropriate mechanical properties. These materials should also enhance the cell attachment with the prepared scaffold. To overcome the low mechanical strength of HA, it is important to combine HA, as a filler, in the biopolymer. In general, the human bone matrix is an organic and inorganic composite biomaterial [Citation4]. Biodegradable polymers such as polylactic acid (PLA), polycaprolactone (PCL) and its copolymer poly(lactic-glycolic) acid (PLGA) are used mainly for the biomedical applications [Citation5,Citation6].PLAisextensively used in the field of biomedical application [Citation7], with its biocomposite with HAconstituting the most common scaffold materials in literatures. PLA, when blended with HA, not only enhances the strength of the resultant biocomposite but also has an effective role in the formation of new bone [Citation8]. Curcumin is one of the main active components that are extracted from the rhizomes of turmeric plants (Curcuma longa L). The extensive uses of curcumin are related to its various medical applications and therapeutic activities [Citation9]. Curcumin has a highly pleiotropic action and can physically interact with an adverse range of molecular targets including growth factors, transcriptional factors, enzymes, cytokines, and genes regulating cell apoptosis and proliferation [Citation10–Citation12].
Various clinical and pre-clinical studies demonstrated that curcumin has an anti-microbial, anti-inflammatory, anti-oxidant, anti-arthritic, and anti-carcinogenic, and wound healing properties [Citation13–Citation15]. The ability of curcumin to enhance wound healing is related to its effectiveness in increasing biosynthesis of extracellular matrix proteins such as collagen [Citation16,Citation17].
The main purpose of this work is to fabricate a biocomposite suitable as a scaffold for bone tissue engineering and increase the effectiveness of the fabricated biocomposite in bone regeneration activity by the addition of curcumin.
Materials and methods
Materials
Calcium Nitrate A.R (Ca(NO3)2.4H2O) was purchased from Winlab, UK. Di-ammonium Hydrogen Phosphate ((NH4)2.HPO4) was purchased from Sisco Research Laboratories pvt. Ltd, India. Polylactic acid (PLA) pellets (Mw = 60,000) and curcumin powder (C21H20O6, curcumin longa (Turmeric)), were purchased from Sigma-Aldrich, UK. All chemicals were used without further purification.
Preparation of the biocomposite
Hydroxyapatite nano-powder was prepared via chemical preparation method previously described in [Citation18,Citation19]. Briefly, calcium nitrate and diammonium hydrogen phosphate were dissolved in distilled water then mixed at pH range 11–12 by adding drops of ammonium solution. The precipitate was dried and sintered to obtain the HA nano-powder.PLA was dissolved in chloroform then the required quantity of prepared nano-HA powder was dispersed in the PLA solution using probe sonicator. The ratio PLA/HA was kept as 80/20 wt%. For the curcumin release studies, the desired amount of curcumin powder was dissolved in chloroform solution and added to the biocomposite at different ratios 1, 2, 3, 4 and 5wt%to the total composite. After complete mixing of all solutions, the solutions were poured into Petri-dishes and dried at room temperature, then cut into pieces for characterizations.
Structural and morphological analyses
Fourier transform infrared (FTIR) absorption spectra of the pure and biocomposite samples were obtained using the FTIR spectrometer Nicolet iS10, USA, in the wavenumber range 4000–400 cm−1 at room temperature. X-ray diffraction (XRD) analysis was performed using X-ray diffractometer, copper Kα (λ = 1.540 A°) (PANalytical X’Pert PRO) within Bragg angle 5–70◦. High-resolution transmission electron microscope (HR-TEM) (JEOLJEM-2100, USA) with a CCD camera at an accelerating voltage of 200 kV was used to investigate the average size and size distribution of the nanoparticles. The Morphology of the prepared samples was examined using Field Emission scanning electron microscope (FESEM) (JEOL JSM 6510 LV, USA).
In vitro test
In vitro investigations were performed in 50 ml capacity screw polyethylene bottles, which contains 10 ml of SBF and 1 cm2 of each sample (in film form). The SBF solution for bioactivity studies was prepared by dissolving the following chemical reagents: 8.026g-NaCl, 0.352g-NaHCO3, 0.225g-KCl, 0.230g-K2HPO4.3H2O,0.311g-MgCl2.6H2O, 0.293g-CaCl2 and 0.072g-Na2SO4 into 700 ml distilled water. The temperature was kept at 37 ◦C and the pH was adjusted at 7.4 with 6.063 g of tri-hydroxymethyl-aminomethane and 40 ml hydrochloric acid [Citation20]. The weight/volume ratio between samples and SBF was adjusted to be 1 g:10 ml. Sealed bottles were kept at 37°C in a water bath. The samples were soaked in SBF for 14 days before they were taken out for testing and analyses. The SBF was replaced at constant times to keep the Ca2+ and P+5 ion concentrations constant. Formation and morphology of apatite-like structures in prepared samples were examined using FESEM and confirmed by EDX measurements.
Drug release studies
Similar pieces from the biocomposite films loaded with different ratios of curcumin 1, 2, 3, 4 and 5wt% were immersed in 10 ml of phosphate buffer solution (PBS) containing 30% ethanol at pH 7.4. The temperature was fixed at 37°C. The samples were then incubated on a shaker at 150 rpm. Following, 2 ml of curcumin solution was withdrawn at different time intervals to measure the release percentage. Calibration curve of curcumin solution was measured using UV spectrometer (T80+, UV/Vis. spectrometer, PG Instrument Ltd.) at λmax431 nm. Using the curcumin calibration curve, the drug content was determined, and the cumulative %drug release against time was calculated using a normal plot according to the equation:
Results and discussions
FTIR analyses
shows the FTIR spectra of pure curcumin, PLA and their biocomposite with HA. The FTIR spectrum of curcumin shows a sharp absorption band at 3508 cm−1 due to the OH group. The sharp absorption band at 1626 cm−1 is mainly due to the overlapping between C = C and C = O groups. A strong absorption band at 1601 cm−1 is attributed to the stretching symmetric vibrations of the (C = C) aromatic ring. The 1508 cm−1 absorption band is attributed to the (C = O), while the absorption band at 1272 cm−1is due to the enol group (C–O) [Citation21,Citation22]. The spectrum of PLA shows a sharp absorption band at 1737cm−1 attributed to the stretching vibration of the carbonyl group (C = O) in the – CO–O – group of the PLA. Another sharp absorption band at 1165 cm−1 is related to the stretching vibration of – C–O – in the – CH–O – group in the chain of PLA polymer. Triple mountainous like peaks at 1110, 1072 and 1025 cm−1, assigned as the stretching vibrations in–CO–O–group in the polymer chain [Citation23]. The spectrum of PLA-HA biocomposite shows the appearance of characteristic bands of HA inorganic phase with polymer matrix peaks.
In comparison with the pure PLA, PLA-HA biocomposite has two strong peaks appear at 563 and 602 cm−1 characteristic for the bending modes of PO4−3. The sharp band at 1384 cm−1 is assigned as N-O stretching mode of NO3−. The stretching mode of PO4−3 is assigned at 1031 cm−1. These characteristic peaks indicate the presence of HA in the composite [Citation18]. The spectra for the PLA-HA-curcumin scaffold show the same characteristic peaks of PLA and HA without disturbance. This stability of the biocomposite may be attributed to the formation of ion-dipole bond between oxygen in the ester group of PLA and the calcium in HA [Citation24]. This confirms the tight bonding between the HA and the PLA matrix. The curcumin peaks appear in the biocomposite matrix without any shift due to its loading without chemical interaction with the scaffold materials. This physical interaction is important for the drug release mechanism.
X-ray diffraction (XRD) analysis
XRD diffraction spectra in show the presence of characteristic peaks of the different composition of the biocomposite. XRD of pure HA shows the characteristic diffraction peaks represented at 2θ = 25.95°, 31.51°, 39.70°, 46.78°, and 48.00° [Citation18]. The PLA spectrum shows the most intense peaks of PLA, especially at 2θ = 17° and 19°. The PLA-HA and PLA-HA-curcumin biocomposite spectra confirmed the existence of crystalline HA phase in the biocomposite. All diffraction peaks of HA in the biocomposite appear at almost the same angles and intensity before and after the addition of curcumin. This confirms that the presence of PLA and curcumin do not affect the structure of HA crystals. In the spectra of curcumin and PLA-HA-curcumin biocomposite, the characteristic peaks confirm the presence of curcumin in the crystalline form as indicated by the main peaks at 2θ = 7.96, 8.90, 12.26, 14.54, 17.24° [Citation21]. It appears that some peaks of curcumin are absent in the spectrum of the biocomposite scaffold, which suggests the good diffusion of curcumin inside the scaffold matrix. Thus, the curcumin peaks are too broadened, and the XRD pattern is dominated by the structure of the PLA-HA components.
Morphology of the biocomposite
shows the images obtained by the transmission electron microscope of the prepared biocomposite. The figure shows the formation of nano-hydroxyapatite surrounded by PLA and curcumin with good homogeneity. The appeared agglomeration can be due to the ion-dipole bond between ester group of PLA and the calcium in HA which is in agreement with the FTIR results.
shows the FESEM images of the surface morphology of the prepared biocomposite. As shown in , HA and curcumin were successfully integrated into the biocomposite matrix with no higher visible agglomeration of the HA particles. shows the surface morphology of the PLA-HA and PLA-HA-curcumin biocomposites after in vitro bioactivity test. The microimages show the precipitation of apatite-like structure on the biocomposite surface with precipitation increasing after the addition of curcumin. The higher perception of bone-like apatite structure in curcumin containing biocomposite may be due to the effect of curcumin in increasing mineralization and nucleation of the apatite crystals [Citation25]. The EDX analysis () shows that the precipitated crystals are apatite-like structures with Ca:P ratio of 1.665 which is comparable to the Ca:P ratio in human bone (1.67) [Citation26].
Curcumin release studies
To study the percent of curcumin release from the curcumin-loaded biocomposite, the calibration curve of curcumin is shown in . The following equation calculated from the calibration curve of curcumin used to calculate the release percent at different times, and the release behavior is shown in .
As shown in , the relation plotted between drug release percent and time for different-loaded curcumin samples shows a nonlinear relationship, which confirms that curcumin release is a biphasic process [Citation27]. The biphasic release behavior is described by an initial burst release phase (fast release) and then a sustained successive release phase [Citation28]. The burst release from the biocomposite sample was about 50% of the loaded curcumin during the first 2 h. This burst curcumin release may be due to the existence of curcumin on or near the biocomposite surface. The curcumin is then released slowly and reach its maximum value after 7 to 9 h of release in the saline medium. Percent of release is then reduced gradually with 2–4% from its maximum value after 25 h of immersion in the saline medium. The long-term release and value of the maximum curcumin release from the biocomposite was attributed to the low degradation rate of polylactic acid in the water saline medium [Citation29]. Taking into account the controlled release profile of PLA-HA-curcumin scaffolds, the long-term anti-inflammatory effect can be expected, when they are applied as drug-loaded scaffolds for bone repair and regeneration [Citation28]. These results agree with the previous curcumin release experiments by Suwantong et. al [Citation30]. and Mai et. al [Citation22].
Conclusions
PLA-HA-curcumin biocomposite scaffolds were prepared via the precipitation method with PLA/HA ratio of 80/20 wt% and different ratios of curcumin. The FTIR analysis shows a good stability of the biocomposite due to the ion-dipole binding between PLA and HA and show no chemical interaction between curcumin and scaffold materials, which is important for the drug release mechanism. XRD analysis suggested good miscibility and diffusion of curcumin inside the scaffold matrix, which appeared also in FESEM micro-images. Curcumin addition increased the surface bioactivity of the HA-PLA biocomposite through the formation of the bone-like structure after immersion in SBF. All results confirm that HA-PLA-curcumin biocomposite has a high degree of biological activity, which makes it very suitable for the field of biomaterials and other various medical applications.
Highlights
PLA-HA-curcumin biocomposite scaffolds with PLA/HA ratio of 80/20 wt% and different ratios of curcumin were prepared via the precipitation method.
Spectroscopic analysis proves the formation of ion-dipole between PLA and HA which reveal good stability of the biocomposite.
Addition of curcumin increased the surface bioactivity of the HA-PLA biocomposite through the formation of the bone-like structure after immersion in SBF.
The in vitro and drug release behavior studies confirm that the fabricated biocomposite is appropriate for medical applications.
Disclosure statement
No potential conflict of interest was reported by the authors.
References
- Rutledge K, Cheng Q, Pryzhkova M, et al. Enhanced differentiation of human embryonic stem cells on extracellular matrix-containing osteomimetic scaffolds forbone tissue engineering. Tissue Eng Part C Methods. 2014;20:865–874.
- Skrtic D, Antonucci JM, Eanes E. Amorphous calcium phosphate-based bioactive polymeric composites for mineralized tissue regeneration. J Res Natl Inst Stand Technol. 2003;108:167–182.
- Stevens MM. Biomaterials for bone tissue engineering. Mater Today. 2008;11:18–25.
- Liu X, Ma PX. Polymeric scaffolds for bone tissue engineering. Ann Biomed Eng. 2004;32:477–486.
- Yazdimamaghani M, Razavi M, Vashaee D, et al. Development and degradation behavior of magnesium scaffolds coated with polycaprolactone for bone tissue engineering. Mater Lett. 2014;132:106–110.
- Ramot Y, Haim-Zada M, Domb AJ, et al. Biocompatibility and safety of PLA and its copolymers. Adv Drug Deliv Rev. 2016;107:153–162.
- Boskey A, Camacho NP. FT-IR imaging of native and tissue-engineered bone and cartilage. Biomaterials. 2007;28:2465–2478.
- Hrubovčáková M, Kupkova M, Džupon M, et al. Biodegradable polylactic acid and polylactic acid/hydroxyapatite coated iron foams for bone replacement materials. Int J Electrochem Sci. 2017;12:11122–11136.
- Maheshwari RK, Singh AK, Gaddipati J, et al. Multiple biological activities of curcumin: a short review. Life Sci. 2006;78:2081–2087.
- Alok A, Singh ID, Singh S, et al. Curcumin: pharmacological actions and its role in head and neck squamous cell carcinoma-A review. J Indian Acad Oral Med Radiol. 2017;29:115–118.
- Zhu Y, Bu S. Curcumin induces autophagy, apoptosis, and cell cycle arrest in human pancreatic cancer cells. Evid Based Complement Alternat Med. 2017;2017:1–13.
- Lou S, Wang Y, Yu Z, et al. Curcumin induces apoptosis and inhibits proliferation in infantile hemangioma endothelial cells via downregulation of MCL-1 and HIF-1α. Medicine (Baltimore). 2018;97: 1–7.
- Anand P, Thomas SG, Kunnumakkara AB, et al. Biological activities of curcumin and its analogues (Congeners) made by man and mother nature. Biochem Pharmacol. 2008;76:1590–1611.
- Menon VP, Sudheer AR. Antioxidant and anti-inflammatory properties of curcumin. The molecular targets and therapeutic uses of curcumin in health and disease. Advances in Experimental Medicine and Biology, Springer; 2007. p. 105–125.
- Basniwal RK, Buttar HS, Jain V, et al. Curcumin nanoparticles: preparation, characterization, and antimicrobial study. J Agric Food Chem. 2011;59:2056–2061.
- Panchatcharam M, Miriyala S, Gayathri VS, et al. Curcumin improves wound healing by modulating collagen and decreasing reactive oxygen species. Mol Cell Biochem. 2006;290:87–96.
- Sidhu GS, Mani H, Gaddipati JP, et al. Curcumin enhances wound healing in streptozotocin induced diabetic rats and genetically diabetic mice. Wound Repair Regener. 1999;7:362–374.
- El‐Bahy G, Abdelrazek E, Allam M, et al. Characterization of in situ prepared nano‐hydroxyapatite/polyacrylic acid (HAp/PAAc) biocomposites. J Appl Polym Sci. 2011;122:3270–3276.
- Abdel-Aziz MS, Hezma A. Spectroscopic and antibacterial evaluation of nano-hydroxapatite polyvinyl alcohol biocomposite doped with microbial-synthesized nanogold for biomedical applications. Polym-Plast Technol Eng. 2013;52:1503–1509.
- Paital SR, Balani K, Agarwal A, et al. Fabrication and evaluation of a pulse laser-induced Ca–P coating on a Ti alloy for bioapplication. Biomed Mater. 2008;4:015009.
- Singh PK, Wani K, Kaul-Ghanekar R, et al. From micron to nano-curcumin by sophorolipid co-processing: highly enhanced bioavailability, fluorescence, and anti-cancer efficacy. RSC Adv. 2014;4:60334–60341.
- Mai TTT, Nguyen TTT, Le QD, et al. A novel nanofiber Cur-loaded polylactic acid constructed by electrospinning. Adv Nat Sci. 2012;3:025014.
- Wang N, Zhang X, Ma X, et al. Influence of carbon black on the properties of plasticized poly (lactic acid) composites. Polym Degrad Stab. 2008;93:1044–1052.
- Zhang J, Yin H-M, Hsiao BS, et al. Biodegradable poly (lactic acid)/hydroxyl apatite 3D porous scaffolds using high-pressure molding and salt leaching. J Mater Sci. 2014;49:1648–1658.
- Moran JM, Roncero-Martin R, Rodriguez-Velasco FJ, et al. Effects of curcumin on the proliferation and mineralization of human osteoblast-like cells: implications of nitric oxide. Int J Mol Sci. 2012;13:16104–16118.
- Cengiz B, Gokce Y, Yildiz N, et al. Synthesis and characterization of hydroxyapatite nanoparticles. Colloids Surf A Physicochem Eng Asp. 2008;322:29–33.
- Hasan A, Waibhaw G, Tiwari S, et al. Fabrication and characterization of chitosan, polyvinylpyrrolidone, and cellulose nanowhiskers nanocomposite films for wound healing drug delivery application. J Biomed Mater Res A. 2017;105:2391–2404.
- Hu Y, Gu X, Yang Y, et al. Facile fabrication of poly (l-lactic acid)-grafted hydroxyapatite/poly (lactic-co-glycolic acid) scaffolds by pickering high internal phase emulsion templates. ACS Appl Mater Interfaces. 2014;6:17166–17175.
- Razak SA, Sharif N, Rahman W. Biodegradable polymers and their bone applications: a review. Int J Basic Appl Sci. 2012;12:31–49.
- Suwantong O, Opanasopit P, Ruktanonchai U, et al. Electrospun cellulose acetate fiber mats containing curcumin and release characteristic of the herbal substance. Polymer. 2007;48:7546–7557.