ABSTRACT
Chemotherapy remains the utmost treatment for Type 1 diabetes (T1D) patients. This however, predisposes the patients to a wide range of serious complications, thus, the need for alternative therapeutic agents that target pancreatic β-cell function. This study investigated the effects of aqueous leaf extract of M. lucida (MLE) on β-cell dysfunction and atherogenic dyslipidaemia in alloxan-induced T1D in rats. Twenty-five Wister rats (156- 168g) were randomly divided into normal, diabetic, diabetic + glibenclamide (5 mg/kg), diabetic + 120 mg/kg MLE and diabetic + 240 mg/kg MLE groups (n = 5/ group). Treatments were via oral route for 14 days. Our findings revealed that, 120 mg/kg MLE significantly reduced hyperglycaemia, improved insulinaemia as well as β-cell function, and attenuated weight loss in alloxan-induced diabetic rats. The extract also attenuated (p < 0.05) atherogenic dyslipidaemia and malondialdehyde. The activities of the extract compared favourably with glibenclamide. This study suggested that, hypoglycaemic and mitigating effects of aqueous leaf extract of M. lucida on atherogenic dyslipidaemia and pancreatic β-cell dysfunction were through reduction in lipid peroxidation. The extract may therefore represent an effective source of novel drugs against TID and cardiovascular diseases. Further study is recommended, to explore the extract’s mechanism of oxidative repair.
Introduction
Type 1 diabetes (T1D) is a poorly controlled chronic metabolic disorder that is characterized by beta-cell destruction, insulin deficiency and hyperglycemia [Citation1,Citation2]. It accounts for about 5–10% global incidence of diabetes mellitus, affecting approximately 20 million people worldwide, with rising incidence [Citation3]. Approximately 542,000 children (aged 0–14 years) have T1D and 86,000 new cases are diagnosed globally each year [Citation4]. Although, the disease is often referred to as ‘juvenile diabetes’ with symptoms such as polydypsia, polyphagia, polyuria and weight loss, alongside overt hyperglycemia [Citation5], it has been recently shown that individuals with T1D are mostly adults and almost half of the cases of T1D were diagnosed after age 30 years [Citation6–Citation8]. Furthermore, it has been reported that about 5–15% of adults diagnosed with T2D may actually have T1D [Citation9]. Hence, poor understanding of this disease has led to underestimation in adults. Pathogenetically, T1D results from an interplay between genetic and environmental factors that induce autoimmune destruction of insulin-producing pancreatic β-cells [Citation10]. Factors such as diet, enteroviruses and toxins have been proposed to influence the development of T-cell-dependent autoimmunity in genetically susceptible individuals [Citation11].
Normally, hyperglycemia inhibits glucagon secretion. However, during insulin insufficiency, hyperglycemia elevates glucagon level and body then utilizes fat for energy instead of glucose [Citation12,Citation13]. This leads to accumulation of ketones in the blood, consequently results in diabetic ketoacidosis and therefore slows down body function [Citation12,Citation13]. This may consequently result in coma and eventually death [Citation14]. Similarly, hyperglycemia induces multiple cellular changes which generate oxidative stress via several mechanisms such as nonenzymatic glycation, glucose oxidation and alterations in polyol pathway activity [Citation15,Citation16]. Consequently, the generated oxidative stress leads to DNA, lipid, protein and carbohydrate damage, and increases the risk of developing complications such as neuropathy, nephropathy, retinopathy and accelerated coronary artery disease [Citation17].
Chemotherapy, including insulin treatment, remains the utmost treatment for people with T1D [Citation18,Citation19]. These therapies act by improving insulin secretion, decreasing glucose release from the liver or reducing gastrointestinal absorption of sugars [Citation19]. However, while insulin and other commonly administered drugs do not provide absolute cure, they also predispose the patients to a wide range of serious complications, such as heart and kidney diseases and blindness [Citation20,Citation21]. To avoid these complications, production of new β-cells becomes necessary. These new β-cells should ideally be formed from already existing cell sources within an individual with diabetes to avoid immunosuppression [Citation22]. Alternatively, exogenous sources of surrogate β-cells, such as adult human pancreases donated after death, fetal pancreas, pluripotent and multipotent stem cells have also been propagated, but not much success has been recorded so far [Citation23].
In order to meet up with the current challenge, therefore, there is a need to search for effective and safe alternative sources of treatment through herbal therapy, to provide improved treatment for diabetes and associated complications [Citation23]. Morinda lucida is one of the widely used medicinal plants in West Africa (especially Nigeria), for the management/treatment of various types of ailments [Citation24]. Antidiabetic effects of M. lucida extracts have been previously reported [Citation25–Citation28]. However, there is insufficient information on the effect of these extracts on pancreatic β-cell function and atherogenic dyslipidaemia. In this study, we investigated the ameliorative effects of M. lucida aqueous leaf extract on pancreatic β-cell function and atherogenic dyslipidaemia in alloxan-induced T1D.
Materials and method
Sample collection and preparation of extract
Fresh leaves of M. lucida were harvested from Rot-Tund farms Nigeria investment limited, Ila-Orangun, Osun State, Nigeria in December, 2017 and authenticated at the herbarium department, Forest Research Institute of Nigeria, Ibadan, Nigeria (Registration number FHI.110162). The leaves were properly cleaned, air dried and macerated. The macerated samples (100 g) were dissolved in 1000 ml of distilled water for 24 h and then filtered. The filtrate was concentrated under reduced pressure, using a rotary evaporator at 55°C to yield a crude semisolid mass, which was stored in a refrigerator at 4°C until required.
Experimental animals
Twenty-five male Wistar rats, weighing 156–168 g were procured from the Animal House, Department of Biochemistry, University of Ilorin, Ilorin, Nigeria. They had unrestricted access to standard feed and water. The animals were maintained under standard environmental conditions of temperature, relative humidity and dark/light cycle, in accordance with the guidelines of National Institutes of Health Guide for the Care and Use of Laboratory Animals and the Institutional Ethical Review Board of University of Ilorin. Body weight, food consumption and water intake were monitored throughout the period of administration.
Induction of diabetes
The rats were fasted overnight and then injected, intra-peritoneally, with a single dose of 0.5 ml of 160 mg/kg body weight (bw) of Alloxan monohydrate (a product of Mekphar Chemical Pharmaceutical Joint-Stock Company, Chiminh City, Vietnam), dissolved in freshly prepared normal saline, to induce T1D [Citation29]. The control animals (nondiabetic) were injected with 0.5 ml of the vehicle (normal saline). Stable hyperglycemia was confirmed on ninth day [Citation29], using glucometer (ACCU-Chek, Roche Diagnostics) and this was taken as week 0. Rats with fasting blood glucose level greater than 200 mg/dl were considered diabetic and used for this study.
Animal grouping and treatment
After 2 weeks of acclimatization, animals were randomly assigned to five groups (n = 5/group). Groups 1 (normal control) and 2 (diabetic control): received distilled water (vehicle) daily; Group 3: (diabetic + glibenclamide): received 5 mg/kg bw glibenclamide; Groups 4 (diabetic + 120 mg/kg MLE): received 120 mg/kg bw aqueous M. lucida leaf extract; while Group 5 (diabetic + 240 mg/kg MLE): received 240 mg/kg bw aqueous M. lucida leaf extract. The MLE doses used were in accordance with previous study [Citation25], with slight modification. All treatments were through oral administration and lasted for 14 days.
Blood collection
At the end of experiment, four (4) rats, from each group, were sacrificed by cervical dislocation and blood was then collected by cardiac puncture into plain bottle. Blood samples were centrifuged at 3000 rpm for 5 min and serum was stored frozen until needed for biochemical assays.
Biochemical assays
Insulin was determined using ELISA kit from Ray Biotech, Inc. (Georgia, USA), while malondialdehyde (MDA), a marker of lipid peroxidation, was measured by standardized method using kit obtained from Oxford Biomedical Research Ltd (Rochester Hills, MI). Estimation of total cholesterol (TC) and triglyceride (TG) was done by standardized enzymatic colorimetric methods using assay kit obtained from Fortress Diagnostics Ltd. (Antrim, United Kingdom). High-density lipoprotein-cholesterol (HDL-C) was measured by enzymatic clearance assay (Daiichi Pure Chemicals Co., Ltd., Tokyo, Japan) while low-density lipoprotein-cholesterol (LDL-C) was estimated using modified Friedewald’s formula [Citation30]. TC/HDL-C and TG/HDL-C ratios were estimated as markers of atherogenic lipid indices [Citation31].
Fasting blood glucose and pancreatic β-cells
Weekly fasting blood glucose was assessed using glucometer (ACCU-Chek, Roche Diagnostics). The pancreatic β-cell function was estimated using HOMA-β formula [Citation31].
Statistical analysis
Data were analyzed and presented as mean ± SEM. One-way analysis of variance (ANOVA) followed, by Bonferroni post hoc test was performed, using PRISM 5.0 (GraphPad Software, USA). Values were considered statistically significant at p < 0.05.
Results
Effect of M. lucida leaf extract (MLE) on fasting blood glucose (FBG) and fasting insulinemia in alloxan-induced diabetic rats
Fasting blood glucose was higher than 200 mg/dl in diabetic control as well as 240 mg/kg bw MLE-treated group (postinduction), throughout the experimental period ()), while treatment with glibenclamide and 120 mg/kg MLE progressively reduced FBG (p < 0.05). There was decrease (p < 0.05) in fasting insulinemia in diabetic control, compared with normal control ()). However, glibenclamide and 120 mg/kg MLE treatments significantly improved fasting insulinemia.
Figure 1. Effect of M. lucida leaf extract (MLE) on fasting glycemia and insulinemia in alloxan-induced diabetic rats. Fasting glycemia was kept above 200 mg/dl in both diabetic control and 240 mg/kg MLE group, while glibenclamide and 120 mg/kg MLE treatments lowered fasting glycemia. Meanwhile, alloxan reduced circulating insulin, while administration of glibenclamide and 120 mg/kg MLE elevated blood insulin level (*p < 0.05 vs. normal control; #p < 0.05 vs. diabetic control; n = 4).
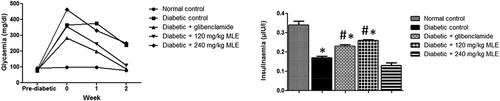
Effect of M. lucida leaf extract (MLE) on body weight and pancreatic β-cell function in alloxan-induced diabetic rats
Alloxan treatment resulted in gradual loss in body weight (), while glibenclamide and MLE increased weight loss. Similarly, pancreatic β-cell function was reduced (p < 0.05) in diabetic control when compared with normal control, whereas glibenclamide and 120 mg/kg MLE significantly improved β-cell function ().
Figure 2. Effect of glibenclamide and M. lucida leaf extract (MLE) on body weight in alloxan-induced diabetic rats. Alloxan treatment led to decrease in body weight, while alloxan + glibenclamide and alloxan + MLE (120 and 240 mg/kg) treatments improved body weight (n = 4).
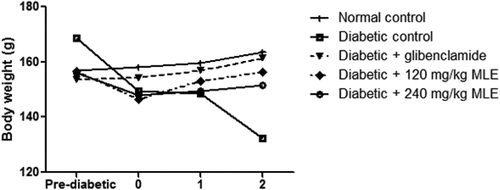
Figure 3. Effect of glibenclamide and M. lucida leaf extract (MLE) on pancreatic β-cell function in alloxan-induced diabetic rats. Alloxan treatment decreased pancreatic β-cell function. Meanwhile, treatment with glibenclamide and 120 mg/kg MLE improved β-cell function (*p < 0.05 vs. normal control; #p < 0.05 vs. diabetic control; n = 4).
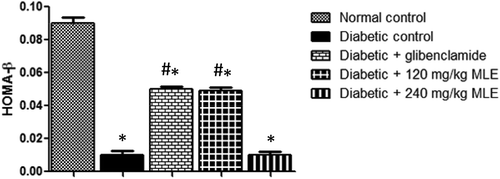
Effect of M. lucida leaf extract (MLE) on lipid profile, atherogenic lipids and malondialdehyde (MDA) in alloxan-induced diabetic rats
shows the effect of glibenclamide and MLE on lipid profile in alloxan-induced diabetic rats. There was increase (p < 0.05) in total cholesterol (TC) and LDL-C in diabetic control compared with normal control, whereas, HDL-C was decreased (p < 0.05) in diabetic control. Upon treatment with glibenclamide and MLE, alloxan-induced dyslipidemia was attenuated. As shown in , the atherogenic indices (TC/HDL-C and TG/HDL-C) were increased (p < 0.05) in diabetic control when compared with normal control. However, treatment with glibenclamide and MLE led to attenuation in atherogenic indices. Similarly, alloxan treatment elevated MDA in diabetic control (p < 0.05) as compared with normal control, while glibenclamide and MLE lowered MDA ().
Table 1. Effect of glibenclamide and M. lucida leaf extract (MLE) on lipid profile in alloxan-induced diabetic rats.
Figure 4. Effect of glibenclamide and M. lucida leaf extract (MLE) on atherogenic indices (TC/HDL-C and TG/HDL-C) in alloxan-induced diabetic rats. Alloxan treatment increased atherogenic indices. Glibenclamide and MLE treatments attenuated atherogenic indices (*p < 0.05 vs. normal control; #p < 0.05 vs. diabetic control; n = 4).

Discussion
This study investigated the effect of aqueous leaf extract of M. lucida on pancreatic beta-cell function and dyslipidemia in type 1 diabetic rats, using alloxan induction. Alloxan is a cytotoxic diabetogenic compound, widely used in experimental diabetes research [Citation32]. It stimulates insulin-dependent diabetes (T1D) by inducing selective necrosis of the beta-cells of pancreatic islets, thus, destroying β-cells and reducing their function [Citation33,Citation34]. Our results showed that M. lucida aqeous leaf extract lowered alloxan-induced hyperglycemia at low dose (120 mg/kg), which compared favorably with glibenclamide. The main etiology of T1D is destruction of pancreatic β-cells [Citation35]. In this study, treatment of diabetic rats with the extract improved pancreatic β-cell function and thus, insulin production, better than the standard drug (glibenclamide) used. This may imply that, the leaf extract of M. lucida induced regeneration of pancreatic β-cell, and hence, enhanced its function. Our observation agrees with an earlier study, which reported that leaf extracts of M. lucida ameliorated alloxan-induced pancreatic damage [Citation36].
Insulin deficiency in T1D results in stimulation of lipase, which hydrolyzes stored adipocyte triglyceride to fatty acids and glycerol, and consequently leads to loss of body weight [Citation35]. In conformity with previous report [Citation24], this study showed that M. lucida leaf extract improved alloxan-induced weight loss following increase in insulinemia. Our results thus imply that, the extract is capable of increasing metabolic efficiency via stimulation of insulin secretion. It may, therefore, be a good therapeutic agent for alleviating metabolic inefficiency in T1D and similar conditions.
Atherogenic indices (TC/HDL-C and TG/HDL-C ratios) have been identified as successful markers for predicting individuals at the risk of atherosclerosis [Citation36,Citation37], which begins early in life [Citation38]. Therefore, our results which showed that alloxan increased TC/HDL-C and TG/HDL-C ratios and that treatment with leaf extract of M. lucida attenuated rise in the ratios are very prominent. The results suggest that, monitoring TC/HDL-C and TG/HDL-C ratios may assist in early detection of associated atherogenic dyslipidemia in T1D and that, leaf extract of M. lucida could serve as a good therapeutic agent for early intervention against cardiometabolic disorders.
It has been reported that reactive oxygen species produced by oxidative stress can penetrate through cell membranes, causing damage to the pancreatic β-cells and reducing insulin sensitivity [Citation39,Citation40]. Hence, oxidative stress plays a vital role in the pathophysiology of diabetes and pancreatic β-cell dysfunction [Citation40,Citation41]. Our result which shows elevated MDA level in diabetic control indicates increased oxidative stress (lipid peroxidation), and possibly increased tissue damage, by free radicals, in alloxan-induced diabetes [Citation17]. This is in consonance with a previous human study [Citation42] as well as previous observation which showed that alloxan-induced beta-cell destruction is mediated by lipid peroxidation [Citation32]. Upon treatment with the extract, MDA was significantly reduced. This suggests that aqueous leaf extract of M. lucida can improve body antioxidant defense. Meanwhile, earlier study has reported antioxidant activity of M. lucida extracts, attributed to the presence of bioactive components such as phenols, flavanoids and tannins [Citation43]. We therefore suggest that antidiabetic effect of M. lucida leaf extract is through inhibition of lipid peroxidation, which consequently improves β-cell function and attenuates atherogenic dyslipidaemia.
Conclusion
This present study demonstrated that aqueous leaf extract of M. lucida improved pancreatic β-cell function and attenuated dyslipidemia in T1D condition by inhibiting lipid peroxidation. It may therefore represent an effective alternative source of novel drugs, for better management/treatment of T1D and cardiovascular diseases. Further study to explore the mechanism of oxidative repair is recommended.
Author contributions
All the authors have accepted responsibility for the entire content of this submitted manuscript and approved submission.
Disclosure statement
No potential conflict of interest was reported by the authors.
References
- Atkinson MA. Thirty years of investigating the autoimmunebasis for type 1 diabetes. Diabetes. 2005;54:1253–1263.
- Baynes HW. Classification, Pathophysiology, Diagnosis and Management of Diabetes Mellitus. J Diabetes Metab. 2015;6:541.
- American Diabetes Association. Diabetes 2001 Vital statistics.Alexandra. VA: ADA; 2010.
- International Diabetes Federation. IDF Diabetes Atlas. 2015. 7th edn. Available from: www.diabetesatlas.org
- Maahs DM, West NA, Lawrence JM, et al. Epidemiology of type 1diabetes. Endocrinol Metab Clin North Am. 2010;39:481–497.
- Weng J, Zhou Z, Guo L, et al. T1D China Study Group. Incidence of type 1 diabetes in China, 2010–13: population based study. BMJ. 2018;360:j5295.
- Rogers MAM, Kim C, Banerjee T, et al. Fluctuations in the incidence of type 1 diabetes in the United States from 2001 to 2015: a longitudinal study. BMC Med. 2017;15:199.
- Thomas NJ, Jones SE, Weedon MN, et al. Frequency and phenotype of type 1 diabetes in the first six decades of life: a cross-sectional, genetically stratified survival analysis from UK Biobank. Lancet Diabetes Endocrinol. 2018;6:122–129.
- Palmer JP, Hampe CS, Chiu H, et al. Is latent autoimmune diabetes in adults distinctfrom type 1 diabetes or just type 1 diabetes at an older age? Diabetes. 2005;54:62–67.
- Bluestone JA, Herold K, Eisenbarth G. Genetics, pathogenesis and clinical interventions in type 1 diabetes. Nature. 2010;464:1293–1300.
- Devendra D, Liu E, Eisenbarth GS. Type 1 diabetes: recent developments. BMJ. 2004;328:750–754.
- Raju SM, Raju B. Illustrated medical biochemistry. 2nd ed. New Delhi, India: Jaypee Brothers Medical Publishers ltd; 2010. p. 645.
- Siddique AA, Siddiqui AS, Ahmad S, et al. Diabetes: mechanism, pathophysiology and management. Int J Drug Dev Res. 2013;5:1–23.
- Figueiredo LF, Schuster S, Kaleta C, et al. Cansugars be produced from fatty acids? A test case forpathway analysis tools. Bioinformatics. 2009;25:152–158.
- Srivastasan R, Gadde R, Manoj-kumar K, et al. Antioxidants and lipids peroxidation status in diabetic patient with and without complication. Arch Iran Med. 2007;12:121–127.
- Rains JL, Jain SK. Oxidative stress, insulin signaling, anddiabetes. Free Radic Biol Med. 2011;50:567–575.
- Peerapatdit T, Likidlilid A, Patchnans N, et al. Antioxidant status and lipid peroxidation end products in patients of Type 1 Diabetes mellitus. J Med Assoc Thai. 2006;89:141–146.
- Sena MC, Bento CF, Pereira P. Diabetes mellitus: challengesand innovative therapies. Epma J. 2010;1:138–163.
- Corathers SD, Peavie S, Salehi M. Complications of Diabetes Therapy. Endocrinol Metab Clin North Am. 2013;42(4):947–970.
- Morales A. A better future for children with type 1 diabetes: reviewof the conclusions from the diabetes control andcomplications trial and the epidemiology of diabetes interventions and complications study. J Ark Med Soc. 2009;106:90–93.
- Rezaei A, Farzadfard A, Amirahmadi A, et al. Diabetes mellitus and its management with medicinal plants: A perspective based on Iranian research. J Ethnopharmacol. 2015;175:567–616.
- Furth ME, Atala A. Stem cell sources to treat diabetes. J Cell Biochem. 2009;106:507–511.
- Bamisaye FA, Odutuga AA, Minari JB, et al. Evaluation of hypoglycemic and toxicological effects ofleaf extracts of Morinda lucida in hyperglycemic albinorats. Int Res J Biochem Bioinf. 2013;3:37–43.
- Adeneye AA, Olagunju JA, Olatunji BH, et al. Modulatory effect of morinda lucida aqeous stem bark extract on blood glucose and lipid profile in Alloxan induced diabetic rats. Afr.j.Biomed.Res. 2017;20:75–84.
- Adeneye AA, Agbaje EO. Pharmacological evaluationof oral hypoglycemic and antidiabetic effects of fresh leavesethanol extract of Morinda lucida benth. In normal and alloxaninduced diabetic rats. Afr.j.Biomed.Res. 2008;11:65–71.
- Ojewunmi O, Oshodi T, Ogundele O, et al. Evaluation of the antidiabetic and antioxidant activities of aqueous extracts of Morinda lucida and Saccharum officinarum leaves in alloxan-induced diabetic rats. Int J Biochem Res Rev. 2013;3:266–277.
- Chougale AD, Shrimant P, Gurao PM, et al. Optimization of Alloxan dose is essential to induce stable diabetes for prolonged period. Asian J Biochem. 2007;2:402–408.
- Friedewald WT, Levy RI, Fredrickson DS. Estimationof the concentration of low-density lipoprotein cholesterol in plasma, without use of the preparativeultracentrifuge. Clin Chem. 1972;18:499–502.
- Michael OS, Olatunji LA. Ameliorative effect ofnicotine exposure on insulin resistance is accompanied by decreased cardiac glycogen synthasekinase-3 and plasminogen activator inhibitor-1 during oral oestrogen-progestin therapy. Arch Physiol Biochem. 2017. DOI:10.1080/13813455.2017.1369549
- Etuk EU. Animal models for studyingdiabetes mellitus. Agric Biol J North Am. 2010;1:130–134.
- Viana GS, Medeiros AC, Lacerda AM, et al. Hypoglycemic and anti-lipemic effects ofthe aqueous extract from Cissus sicyoides. BMC Pharmacol. 2004;8:4–9.
- Rohilla A, Ali S. Alloxan induced diabetes: mechanismand effect. IJRPBS. 2012; 3:2229–3701.
- Siddique AA, Siddiqui AS, Ahmad S, et al. Diabetes: mechanism, pathophysiology and management. Int J Drug Dev Res. 2013;5:1–23.
- Atanu FO, Momoh S. Amelioration of histological changes and associated metabolic abnormalities by a combination of Morinda lucida and metformin in diabetic rat. J Phytopharmacol. 2018;7:253–256.
- Dokken DB, Tsao T. The physiology of body weight regulation: arewe too efficient for our own good? Diabetic Spectr. 2007;20:166–170.
- Dobiasova M, Frohlichb J. The plasma parameter log (TG/HDL-C) as an Atherogenic index: correlation with lipoprotein particle size and esterification rate in apob Lipoprotein depleted plasma. Clin Biochem. 2001;34:583–658.
- Davidson MH. Ezetimibe/simvastatin compared with atorvastatin or rosuvastatin in lowering to specified levels both LDL-C andeach of five other emerging risk factors for coronary heart disease: non–HDL-cholesterol,TC/HDL-C, apolipoprotein B, apo-B/apo-A-I, or C-reactive protein. Clin Lipidol. 2008;2:436–446.
- Jarvisalo MJ, Jartti L, Nanto-Salonen K, et al. Increased aortic intima-media thickness: a marker of preclinical atherosclerosis in high-risk children. Circulation. 2001;104:2943–2947.
- Chen H, Li X, Epstein PN. MnSOD and catalase transgenes demonstrate that protection of islets from oxidative stress does not alter cytokine toxicity. Diabetes. 2005;54:1437–1446.
- Lepore DA, Shinkel TA, Fisicaro N, et al. Enhanced expression of glutathione peroxidase protects islet beta cells from hypoxia-reoxygenation. Xenotransplantation. 2004;11:53–59.
- Hurrle S, Hsu WH. The etiology of oxidative stress in insulin resistance. Bio Med J. 2017;40:257–262.
- Kantoosh MM, Naiem A, El- Sayad M, et al. Dyslipidemia and lipid peroxidation in type 1 Diabetic children with good glycemic control: response to antioxidant therapy. Alex J Pediatr. 2002;16:357–364.
- Adejo GO, Atawodi SE, Ameh DA, et al. Anti-peroxidant, protective and ameliorative properties of methanol extract of all part of morinda lucida benth in CC14-induced liver injury. Nat Prod Chem Res. 2014;2:S1–003.