ABSTRACT
Mimosa pudica (Fabaceae) is a perennial herb and this plant is reported to have anticonvulsant, antimicrobial, antioxidant, antiulcer, antiasthmatic and wound healing activities. Diabetes dyslipidemic effect of Mimosa pudica is not clear. Hence the study is planned to investigate the antidiabetic and antihyperlipidemic activities of methanolic extract of Mimosa pudica (MEMP) on streptozotocin-induced diabetes mellitus in Sprague-Dawley rats. Antidiabetic and antihyperlipidemic effects of a MEMP was studied at 125, 250 and 500 mg/kg body weight (BW). The antidiabetic potential of MEMP was compared with glibenclamide 20 mg/kg BW. The body weight of rats and blood glucose levels were monitored at regular intervals during the experiment. At the end of the study, the blood sample was collected from the rats for biochemical analysis and they were sacrificed and their organs were used for histopathological analysis. Throughout the study, the diabetic controls rats showed a significant increase in glucose level, total cholesterol (TC), triglycerides (TG), low-density lipoprotein (LDL) and very-low-density lipoprotein (VLDL) when compared with that of control, whereas the animals treated with glibenclamide and MEMP showed a significant reduction in the levels of glucose, TG, LDL, and VLDL when compared with that of diabetic control. In conclusion, MEMP showed a significant antidiabetic and antihyperlipidemic activities in streptozotocin-induced diabetic rats.
Introduction
Diabetes and hyperlipidemia are the two major factors involved in the development of cardiovascular diseases. Diabetes is a heterogeneous disease characterized by hyperglycemia and insulin resistance or a combination of both of these factors [Citation1]. Diabetes frequently exhibits the pattern of elevated triglycerides and low-density lipoprotein (LDL), which is also known as diabetic dyslipidemia [Citation2]. Diabetic dyslipidemia is as the metabolic syndrome may lead to the development of the early cardiovascular disease [Citation3]. The burden of cardiovascular disease is increasing every year and it is one of the major causes of morbidity and mortality particularly high among patients with Type 2 diabetes mellitus [Citation4].
In diabetes, blood lipid levels affected because of the interrelationship between carbohydrates and lipid metabolism [Citation5]. Abnormalities in lipoprotein commonly present in type 2 diabetes. Insulin-resistant is a common abnormality observed in diabetes, which may increase very-low-density lipoprotein (VLDL) secretion [Citation6]. In Type 1 and Type 2 diabetes mellitus, poor glycemic control increases serum triglyceride (TG) levels, intermediate-density lipoproteins (IDL) and VLDL and decreases high-density lipoprotein (HDL) cholesterol levels. It is estimated that 30 – 60% of patients with Type 2 diabetes have dyslipidemia [Citation7].
Recently, there is high interest in the medicine plant compared to the oral hypoglycemic agents for the diabetes mellitus treatment as the oral hypoglycemic agents will bring more common undesirable side effects such as minor hypoglycemia and gastrointestinal problems [Citation8]. However, the medicine plants are highly effective and low cost as well as lesser side effects when compared to the oral hypoglycemic agents, thus they are prescribed [Citation9]. Marles and Farnsworth had estimated that approximately more than 1000 plant species was used as the traditional medicine for diabetes [Citation10]. The antidiabetic activity of the medicinal plants is through several mechanisms, including renal glucose reabsorption, insulin degradative processes inhibition, stimulation of beta cells of islets of Langerhans for insulin secretion, decrease the resistance of insulin, and regenerating or repairing the pancreatic beta cells by increasing the size and number of the cells in islets of Langerhans [Citation11].
Mimosa pudica is a perennial herb and identified as lajjalu in Ayurveda [Citation12]. This plant is reported to have anticonvulsant, antimicrobial, antioxidant, antiulcer, antiasthmatic, hepatoprotective and wound healing activities [Citation13–Citation15]. In preclinical experiments, nanoparticles of Mimosa pudica showed antimicrobial activities [Citation16]. The nanoparticles of aqueous extracts of Mimosa pigra also showed a promising effect on degradation of wastewater pollutants [Citation17].
Diabetes dyslipidemic effect of Mimosa pudica is not clear. Hence, the present study is planned to investigate the antidiabetic and antihyperlipidemic activity of methanolic extract of Mimosa pudica (MEMP) on streptozotocin (STZ)-induced diabetes mellitus in Sprague Dawley rats.
Materials and method
Plant profile
Mimosa pudica Linn. is a creeping annual or perennial herb often grown for its curiosity value, as the compound leaves fold inward and droop when touched and reopens within minutes. Mimosa is a genus of about 400 species of herbs and shrubs, in the subfamily Mimosoideae of the Legume family Fabaceae. The plant is a native of tropical America and naturalized nearly all through the tropical and subtropical parts of India. It is commonly distributed in open spaces, such as waste area, roadside, agricultural land, and natural forests. Phytomedicines potentially benefit to the community thus they are still being practiced in all traditional systems of therapies, including Greco-Arab (Unani-Tibb), Ayurveda, and Chinese medicine [Citation18]. Phytochemical studies on Mimosa pudica have revealed the presence of alkaloids, non-protein amino acid (mimosine), flavonoids C-glycosides, sterols, terpenoids, tannins, and fatty acids. In the phytochemical analysis, the root of Mimosa pudica showed the presence of ascorbic acid, crocetin, D-glucuronic acid, linoleic acid, linolenic acid, palmitic and stearic acids, mimosine, D-xylose, and b-sitosterols. Bufadienolide, D-pinitol, norepinephrine, P-coumaric acid, mimopudine, and mimosine are some of the isolated secondary metabolites of Mimosa pudica [Citation19].
Collection of the plant
Taxonomically identified Mimosa pudica (Fabaceae) plant leaves were collected from rural parts of Sungai Petani (5.6436°N, 100.4894°E, 17 m above the mean sea level), Kedah in Malaysia, after confirmation of its identification and authenticated. The plant leaves were removed from the entire plants and were dried under the shade for a few days until complete dryness was obtained. The dried leaves were then powdered into uniform size by using an electric grinder.
Extraction of leaves
The powdered leaves of Mimosa pudica were weighed and extracted with methanol using direct maceration method. In this method, the coarsely powdered leaves were placed in a stopped conical flask with the methanol solvent. It was allowed to stand for at least 3 to 4 days at the room temperature with frequent agitation until all the soluble matters were dissolved in the solvent. The extraction was then filtered through muslin cloth and the filtrate/ extract was concentrated to a dry mass by evaporation under reduced pressure using rotary evaporator (Rotavapor® R-210, BUCHI Corporation). The MEMP was stored at room temperature until use.
Phytochemical analysis
Phytochemical tests were carried out to identify the presence of glycoside, alkaloid, saponin, steroid, flavonoid, tannins and phenolic compounds in MEMP [Citation20]. The total phenolic content was quantified by using the modified spectrophotometric method. The extract was reconstituted with methanol in the concentration of 0.1, 0.2, 0.4, 0.6, 0.8, 1.0 mg/ml respectively and used for the analysis. The reaction solution was prepared by adding 0.5ml of the sample, 2.5 ml of 0.75% sodium carbonate solution along with the 2.5 ml of 1% Folin-Ciocalteu reagent. The solution was incubated for 15 minutes at a temperature of 45°C. The absorbance was measured at 765 nm. The steps were repeated for the standard gallic acid. The standard calibration curve was constructed by plotting the absorbance at 765 nm versus gallic acid concentration. The total phenolic content was calculated from the calibration curve, and the results were expressed as gallic acid equivalent in mg/g [Citation21].
Animals
Healthy, adult, Sprague-Dawley rats (180 ± 20 g) were used for acute toxicity testing and, antidiabetic and antihyperlipidemic studies. The animals were obtained from Central animal house, AIMST University, Malaysia. Prior approval was obtained from the AIMST University Human and Animals Ethics Committee (AUHAEC/FOP/2018/02) to carry out the study. The animals were housed in large, spacious polyacrylic cages at ambient room temperature with 12 h light/12 h dark cycle. A minimum of 5 days of acclimatization period was allowed before the animals are used in the experiment. The animals were fed with water and normal rodent pellet diet ad libitum.
Acute toxicity testing
Healthy, adult female SD rats were used for the experiment. The acute toxicity testing was performed by using the fixed-dose procedure. Overnight fasted rats were orally fed with the MEMP in increasing dose levels of 250, 500, 1000 and 2000 mg/kg body weight (n = 3 per dose), respectively. The animals were observed for their behavioral, neurological and autonomic profiles continuously for 24 h. After a period of 24 h, the animals were observed for 14 days for mortality. [Citation22].
Antidiabetic and antihyperlipidemic effects of methanolic extract of Mimosa pudica
Healthy, adult male SD rats were used for the experiment. Diabetes mellitus was induced in overnight-fasted rats by administration of single intraperitoneal (I.P.) injection of freshly prepared STZ 55 mg/kg/mL. STZ (110 mg) was dissolved in 2 mL of distilled water (55 mg/kg/mL, I.P.). The preparation was adjusted to a pH of 4–4.5 [Citation19]. After 24 h of diabetes mellitus induction, the rats were given 5% w/v of glucose solution (2 ml/kg BW) to prevent hypoglycemic mortality. Diabetes mellitus was confirmed after 48 h of induction by measuring fasting blood glucose level. Rats with fasting blood glucose of more than 200 mg/dL were considered as diabetics and used for the experiment. Diabetic animals were randomly divided into five groups (Group II–VI) as follows:
Group I: Normal control
Group II: Diabetic control
Group III: Diabetic animals treated with glibenclamide (20 mg/kg/P.O.)
Group IV: Diabetic animals treated with MEMP (125 mg/kg/P.O.)
Group V: Diabetic animals treated with MEMP (250 mg/kg/P.O.)
Group VI: Diabetic animals treated with MEMP (500 mg/kg/P.O.)
Group I (normal control) and group II (diabetic control) rats received 0.5% w/v carboxymethyl cellulose (CMC) while the rats in group III were treated with 20mg/kg body weight (BW) of glibenclamide and rats in group IV-VI were administered with MEMP at dose levels of 125, 250, 500 mg/kg BW. The doses of MEMP were selected from the acute toxicity study findings.
The standard and test drugs were suspended in 0.5% w/v CMC and administered once daily through oral gavage for 21 consecutive days. Throughout the study, variations in experiment animals’ BW were monitored at regular intervals. Few drops of blood were collected through the retro-orbital plexus on 7th and 14th day of the experiment and immediately used for blood glucose determination. At the end of the study (i.e. 21st day), the blood sample was withdrawn from all the experimental rats through retro-orbital plexus puncture. The serum was separated from the blood sample and used for biochemical analysis. Later, the rats were sacrificed and their pancreas, liver and kidney were excised and kept in 10% formalin for histopathology studies. [Citation23–Citation26].
Body weight analysis
At regular intervals, body weight of experimental animals were recorded and change in body weight was calculated.
Biochemical analysis
At the end of the experiment, few millilitres (mL) of the blood sample were collected in the micro-centrifuge tube through retro-orbital plexus and the serum was separated by centrifuging at 3000 RPM for 20 minutes. The serum samples were used for estimation of biochemical parameters such as glucose, aspartate aminotransferase (AST), alanine aminotransferase (ALT), alkaline phosphatase (ALP), creatinine and urea and lipid parameters such total serum cholesterol (TC), TG and HDL cholesterol using Reflotron Plus biochemical analyzer (Roche Diagnostics, Germany) with the help of commercially available Reflotron strips.
LDL, HDL ratio, and atherogenic dyslipidemia (AD) were calculated mathematically. The LDL was calculated using Iranian formula (LDL = TC/1.19 + TG/1.9–HDL/1.1–38 [mg/dL]); HDL ratio was calculated using formula ([HDL-cholesterol/TC–HDL-cholesterol]×100 [%]); VLDL was calculated using formula LDL/5; AD level was calculated using formula (log[TG]/HDL-C) and kidney: BW ratio was calculated using formula (weight of both kidney [mg]/BW of the animal [g]) [Citation22,Citation27].
Gross pathology and organ weight analysis
End of the study, animals were sacrificed under mild ether anaesthesia followed by cervical dislocation. The animal was dissected and the gross pathology was observed. Absolute organ weights were measured and relative organ weight was calculated.
Histopathological analysis
At the end of the study, animals were sacrificed and liver, pancreas, and kidney were excised from all the experimental animals and subjected to histopathological analysis. After gross examination, representative sections were taken from the specimens and placed in 10 percent formalin. Paraffin-embedded tissue blocks were prepared and 4 to 5 µ section were taken with Microtome (Thermo Electron Corporation, England) for routine histopathology. Briefly, the sections for histopathology was placed on an albuminized glass slide, deparaffinized, rehydrated and stained with routine hematoxylin and eosin (H&E). After mounting with DPX and coverslip the slides were examined under a light microscope and reporting was done.
Statistical analysis
The mean ± standard error of the mean (SEM) values was calculated for each group. Statistical differences among the groups were determined using One-way ANOVA followed by Tukey’s post-hoc test. P < 0.05 was considered to be significant.
Results
The phytochemical analysis of the MEMP showed the presence of saponin, flavonoid, tannin, glycoside, alkaloid and phenolic compounds. The total phenolic content analysis of the Mimosa pudica were performed by using the Folin-Ciocalteu method. Total phenolic content of per gram methanolic extract of Mimosa pudica is 99.36 mg GAE/g. In acute toxicity study, MEMP did not show any mortality up to 2000mg/kg. Thus, the study was carried out at the dose levels of 125, 250, 500 mg/kg.
Effect of the glibenclamide, as well as the MEMP on the body weights of STZ-induced diabetes rats, were summarized in . Administration of the glibenclamide and MEMP to the rats with increasing doses of 125 mg/kg, 250 mg/kg and 500 mg/kg did not show any significant difference in the rats’ body weight variations.
Figure 1. Effect of MEPM on body weights of streptozotocin-induced diabetes rat.
All the values are expressed as mean ± SEM (n = 6).
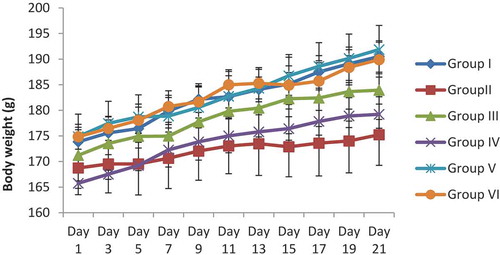
The rats administered with the STZ showed a significant increase in the levels of blood glucose level and lipid profile such as TC, TG, HDL, LDL, HDL ratio, VLDL and AD levels when compared with control. Glibenclamide and MEMP extract showed a significant decrease in the glucose level starting from week 1 onwards when compared with diabetic control () and also inhibited STZ-induced biochemical changes. STZ elevated the levels of AST, ALT, ALP, creatinine, and urea whereas glibenclamide and MEMP inhibited STZ-induced biochemical changes (). Glibenclamide and MEMP treated animals didn’t show any significant changes in lipid profile except for HDL-cholesterol when compared with normal control (). The animals treated with MEMP at 125 mg/kg, showed significant decrease in HDL-cholesterol when compared with normal control (). In organ weight analysis, no significant changes in absolute and relative organ weights were observed with glibenclamide and MEMP treated diabetic rats. Effect of glibenclamide and MEMP on absolute and relative organ weight is tabulated in .
Table 1. Effect of methanolic extract of Mimosa pudica on blood glucose.
Table 2. Effect of methanolic extract of Mimosa pudica on biochemical parameters.
Table 3. Effect of methanolic extract of Mimosa pudica on lipid profile.
Table 4. Effect of methanolic extract of Mimosa pudica on organ weights of SD rats.
Effect of glibenclamide and MEMP on histopathology of the pancreas, liver, and kidneys are shown in –. In diabetic rats, the pancreas showed atrophic islets with vacuolar degeneration as well as the cytoplasmic degranulation in many of the islet cells; liver showed central vein dilation and congestion with lymphocytic infiltration; kidney showed enlarged kidney glomeruli with the decrease in urinary space. Furthermore, the renal tubules showed cytoplasmic vacuoles. Compared with diabetic control, the MEMP treated animals showed a similar type of ameliorative effect on the pancreatic changes as the standard drug glibenclamide.
Figure 2. Histopathology of pancreas of experimental rats (a) Section from pancreas of normal rats showing normal histology of the islet. The islet cells have small, dark nuclei with granular cytoplasm [H & E × 400]; (b) Section from pancreas of diabetic rats showing atrophic islet. The islet cells have vacuolar degeneration [black arrow] [H & E × 400]; (c) Section from pancreas of glibenclamide treated rats showing normal histomorphology [H & E × 400]; (d) Section from pancreas of MEMP extract treated rats showing normal histomorphology [H & E × 400].
N: Nucleus; I: Islets cells; V: Vacuolation.
![Figure 2. Histopathology of pancreas of experimental rats (a) Section from pancreas of normal rats showing normal histology of the islet. The islet cells have small, dark nuclei with granular cytoplasm [H & E × 400]; (b) Section from pancreas of diabetic rats showing atrophic islet. The islet cells have vacuolar degeneration [black arrow] [H & E × 400]; (c) Section from pancreas of glibenclamide treated rats showing normal histomorphology [H & E × 400]; (d) Section from pancreas of MEMP extract treated rats showing normal histomorphology [H & E × 400].N: Nucleus; I: Islets cells; V: Vacuolation.](/cms/asset/2fa2b082-d55d-412c-93d9-243efa0c2e81/teba_a_1681660_f0002_oc.jpg)
Figure 3. Histopathology of liver of experimental rats (a) Section from liver of normal rats showing normal histology of the liver with central vein [H & E × 400]; (b) Section from liver of diabetic rats showing central vein dilation with some congestion [black arrow] and lymphocytic infiltration [red arrow] [H & E × 400]; (c) Section from liver of glibenclamide treated rats showing normal histomorphology [H & E × 400]; (d) Section from liver of MEMP extract treated rats showing normal histomorphology [H & E × 400].
CV: Central vein; R: Regeneration.
![Figure 3. Histopathology of liver of experimental rats (a) Section from liver of normal rats showing normal histology of the liver with central vein [H & E × 400]; (b) Section from liver of diabetic rats showing central vein dilation with some congestion [black arrow] and lymphocytic infiltration [red arrow] [H & E × 400]; (c) Section from liver of glibenclamide treated rats showing normal histomorphology [H & E × 400]; (d) Section from liver of MEMP extract treated rats showing normal histomorphology [H & E × 400].CV: Central vein; R: Regeneration.](/cms/asset/85e1781a-647f-40cf-94a6-0e2ffbeb20d0/teba_a_1681660_f0003_oc.jpg)
Figure 4. Histopathology of kidney of experimental rats (a) Section from kidney of normal histology of the kidney with normal structure of the glomeruli and tubules [H & E × 400]; (b) Section from kidney of diabetic rats showing hypertrophic glomeruli with reduction in the urinary space [red arrow]. The renal tubular epithelium showing some vacuolar degeneration [H & E × 400]; (c) Section from kidney of glibenclamide treated rats showing normal hepatic histomorphology [H & E × 400]; (d) Section from kidney of MEMP extract treated rats showing normal hepatic histomorphology [H & E × 400].
G: Glomerulus; T: Tubule
![Figure 4. Histopathology of kidney of experimental rats (a) Section from kidney of normal histology of the kidney with normal structure of the glomeruli and tubules [H & E × 400]; (b) Section from kidney of diabetic rats showing hypertrophic glomeruli with reduction in the urinary space [red arrow]. The renal tubular epithelium showing some vacuolar degeneration [H & E × 400]; (c) Section from kidney of glibenclamide treated rats showing normal hepatic histomorphology [H & E × 400]; (d) Section from kidney of MEMP extract treated rats showing normal hepatic histomorphology [H & E × 400].G: Glomerulus; T: Tubule](/cms/asset/d2c258eb-7a43-4c1e-ae91-7014e2ddee57/teba_a_1681660_f0004_oc.jpg)
Discussion
In the present study, the antidiabetic and antihyperlipidemic effect of the MEMP was studied against the STZ-induced diabetes mellitus model in the rats. STZ is a toxic glycoside which can be known as the glucosamine-nitrourea. It is obtained from the gram-positive bacterium, Streptomyces achromogene. This chemical agent can be used for the treatment of pancreatic beta cell carcinoma as well as to induce the diabetes mellitus [Citation28]. STZ causes DNA fragmentation, alkylation of cellular components and destroy the pancreatic beta-cells [Citation29]. Destruction of the pancreatic beta-cells will induce type 1 (insulin-dependent) diabetes mellitus in the experimental rats [Citation22].
There is a large influence of the gender of the experimental rats on the development of diabetes mellitus. The male rats are more sensitive to the STZ compared to the female rats. The female rats are more resistant to the low dose of STZ and this could be overcome by increasing the STZ dose [Citation23]. As the pancreatic islet beta cells of the male rats are more prone to the STZ-induced cytotoxicity, hence male rats were used in this study [Citation30]. Since different strains of the animals will display different sensitivities to the STZ, the Sprague-Dawley species of rats are reliably sensitive to this toxin [Citation30].
In the body weight analysis, no significant changes in body weight between the groups were observed. The animals in control and glibenclamide treated groups showed gradual increases in body weight and this may be due to the growth of the skeletal size, epididymal fat and the increases of body mass index [Citation31]. There were no significant changes in the absolute and relative weight of the visceral organs in the treated and the control groups. Organ weight changes are the sensitive indicator of the chemically induced changes to the organs [Citation32].
The MEMP and glibenclamide treated groups showed a reduction of the glucose levels compared to diabetic control. The decrease of the glucose levels may be due to the plasma insulin levels elevation or the enhancement of the blood glucose transportation in the peripheral tissue [Citation33]. Glibenclamide could enhance the insulin secretion from the pancreatic beta cells by the closure of KATP channels. As a result, the membrane will be depolarized and cause the activation of the voltage-dependent Ca2+ channels. The influx of the Ca2+ to the cells will initiate the secretion of insulin [Citation34]. The production of insulin could lower down the glucose level and reverse back the glycemic control. There was a significant elevation of the glucose levels in the diabetic control and this may due to the damage of the beta cells of the pancreas.
The diabetes rats showed increased levels of glucose, AST, ALT, ALP, creatinine, and urea. The elevation of the biochemical markers can be considered as the markers of liver and renal dysfunction [Citation33]. Diabetic rats also showed increased levels of serum cholesterol, TG, LDL, VLDL, and decreased HDL levels. Increased in the levels of TC, LDL and VLDL indicate the development of hyperlipidemia in rats and this may be due to an increase in the activity of hormone-sensitive lipase which catalyzes the mobilization of fatty acids from triacylglycerols stored in adipocytes [Citation35]. This finding was supported by the results of the histopathological analysis. The organs of diabetic rats showed STZ-induced cellular damage on pancreatic islets, liver histology, and renal glomeruli and tubules [Citation33]. The animal treated with glibenclamide and MEMP showed a reduction in the severity of the pathological changes induced by STZ indicating the protective effect of both glibenclamide and MEMP.
Phytochemicals are the bioactive principles that have been widely implicated in ameliorating varies clinical disorders or diseases in which their pathogenesis are remotely or directly connected with oxidative stress [Citation36]. Bioactive principles of the Mimosa pudica plant have been experimentally described to exhibit antioxidant activity. In the majority of the herbal products, the secondary metabolites are used in treatment of diabetes mellitus, and the pharmacological action mediated through several mechanisms, includes regulation of the insulin signaling pathways, translocation of GLUT-4 receptor or activation of the PPAPγ [Citation37]. In the studies, MEMP showed significant antidiabetic and antihyperlipidemic activity against the STZ-induced diabetes mellitus. This may due to the presence of alkaloids or flavonoids. The plant phytoconstituents such as flavonoids, terpenoids, alkaloids, and glycosides have significant antioxidant activity and frequently implicated with the antidiabetic properties [Citation37,Citation38]. The flavonoids that present in the Mimosa pudica could regenerate the damaged beta cells of the pancreases as they are the alpha-amylase inhibitors and the intermediary biosynthetic precursor [Citation39,Citation40]. In addition, the polyphenolic compounds and tannin that presence will inhibit the transportation of the glucose by inhibiting the sodium glucose co-transporter-1 (SGLUT-1) in the intestine of the rats [Citation41]. Thus, the antidiabetic effect of the Mimosa pudica may due to the presence of the flavonoids, polyphenolic compounds, alkaloids as well as the saponins in the methanolic extract.
Conclusion
Methanolic extract of Mimosa pudica showed significant antidiabetic and antihyperlipidemic activities on streptozotocin-induced diabetes mellitus in rats. The antidiabetic potential of the methanolic extract of Mimosa pudica is comparable with that of glibenclamide, which is evidenced by the restoration of blood glucose levels to normal levels.
Disclosure statement
No potential conflict of interest was reported by the authors.
References
- Karalliedde J, Gnudi L. Diabetes mellitus, a complex and heterogeneous disease, and the role of insulin resistance as a determinant of diabetic kidney disease. Nephrol Dialysis Transplantation. 2016;31:206–213.
- Warraich HJ, Wong ND, Rana JS. Role for combination therapy in diabetic dyslipidemia. Curr Cardiol Rep. 2015;17(5):32.
- Schofield JD, Liu Y, Rao-Balakrishna P, et al. Diabetes Dyslipidemia. Diabetes Ther. 2016;7(2):203–219.
- Warraich HJ, Rana JS. Diabetic dyslipidemia: epidemiology and prevention of cardiovascular disease and implications of newer therapies. Curr Cardiol Rep. 2018;20(12):125.
- Dixit AK, Dey R, Suresh A, et al. The prevalence of dyslipidemia in patients with diabetes mellitus of Ayurveda Hospital. J Diabetes Metab Disord. 2014;13:58.
- Goldberg IJ. Clinical review 124: diabetic dyslipidemia: causes and consequences. J Clin Endocrinol Metab. 2001;86(3):965–971.
- Feingold KR, Grunfeld C. Diabetes and Dyslipidemia. 2018 Jan 24 In: De Groot LJ, Chrousos G, Dungan K, et al., editors. Endotext [Internet]. South Dartmouth (MA): MDText.com, Inc.; 2000. PMID: 26247092. Available from: http://www.ncbi.nlm.nih.gov/books/NBK305900/
- Farag M, Ahmed WJ, Foud I, et al. The Role of Medicinal Plants in the Treatment of Type-2 Diabetes. University of Khartoum [Thesis]. [cited 2018 May 22]. Available from: http://khartoumspace.uofk.edu/handle/123456789/23509
- Rao UM, Sreenivasulu M, Chengaiah B, et al. Herbal medicines for diabetes mellitus: A review. Int J PharmTech Res. 2010;2(3):1883–1892.
- Marles RJ, Farnsworth NR. Antidiabetic plants and their active constituents. Phytomedicine. 1995;2(2):137–189.
- Singh P, Mishra A, Singh P, et al. Diabetes mellitus and use of medicinal plants for its treatment. Indian J Res Pharm Biotech. 2015;3(5):351–357.
- Ahmad H, Sehgal S, Mishra A, et al. Mimosa pudica L. (Laajvanti): an overview. Pharmacogn Rev. 2012;6(12):115–124.
- Azmi L, Singh MK, Akhtar AK. Pharmacological and biological overview on Mimosa pudica Linn. Int J Pharm Life Sci. 2011;2(11):1226–1234.
- Jagetia GC, Lalhmangaihi C. Phytochemical profiling and antioxidant activity of lajwanti Mimosa pudica Linn. In Vitro. Int J Plant Stu. 2018;1(1):1–13.
- Rajendiran D, Raghavan S, Kandaswamy S, et al. Hepatoprotective activity of ethanol extract of Mimosa pudica leaves in type 2 diabetic rats. Pharm Innov J. 2018;7(12):223–226.
- James JP, Priya S, Jyothi D. Effect of PLGA polymer on antimicrobial activity and the release studies of nanoparticle hydrogel containing Mimosa pudica extract. Res J Pharm Tech. 2018;11(7):2876–2880.
- Elemike EE, Onwudiwe DC, Ogeleka DF, et al. Phyto-assisted preparation of Ag and Ag–cuO nanoparticles using aqueous extracts of Mimosa pigra and their catalytic activities in the degradation of some common pollutants. J Inorg Organomet Polym Mater. 2019;29:1798–1806.
- Muhammad G, Hussain MA, Jantan I, et al. Mimosa pudica L., a high-value medicinal plant as a source of bioactives for pharmaceuticals. Compr Rev Food Sci Food Saf. 2016;15(2):303–315.
- Ahuchaogu AA, Chukwu OJ, Echeme JO. Secondary metabolites from Mimosa pudica: isolation, purification and NMR characterization. IOSR J Appl Chem. 2017;10(3):15–20.
- Yadav RNS, Agarwala M. Phytochemical analysis of some medicinal plants. J Phytol. 2011;3(12):10–14.
- Kumari D, Madhujith T, Chandrasekara A. Comparison of phenolic content and antioxidant activities of millet varieties grown in different locations in Sri Lanka. Food Sci Nutr. 2016;5(3):474–485.
- Parasuraman S, Balamurugan S, Christapher PV, et al. Evaluation of antidiabetic and antihyperlipidemic effects of hydroalcoholic extract of leaves of Ocimum tenuiflorum (Lamiaceae) and prediction of biological activity of its phytoconstituents. Pharmacogn Res. 2015;7(2):156.
- Deeds MC, Anderson JM, Armstrong AS, et al. Single dose streptozotocin-induced diabetes: considerations for study design in islet transplantation models. Lab Anim. 2011;45(3):131–140.
- Murthy NK, Pushpalatha KC, Joshi CG. Antioxidant activity and phytochemical analysis of endophytic fungi isolated from Lobelia nicotianifolia. J Chem Pharm Res. 2011;3(5):218–225.
- Dhananjayan I, Kathiroli S, Subramani S, et al. Ameliorating effect of betanin, a natural chromoalkaloid by modulating hepatic carbohydrate metabolic enzyme activities and glycogen content in streptozotocin–nicotinamide induced experimental rats. Biomed Pharmacother. 2017;88(1069–79):35.
- Gajdosik A, Gajdosikova A, Stefek M, et al. Streptozotocin-induced experimental diabetes in male Wistar rats. Gen Physiol Biophys. 1999;18:54–62.
- Hermans MP, Ahn SA, Rousseau MF. The atherogenic dyslipidemia ratio [log(TG)/HDL-C] is associated with residual vascular risk, beta-cell function loss and microangiopathy in type 2 diabetes females. Lipids Health Dis. 2012;11:132.
- Shirazi OU, Khan Khattak MMA, Shukri NAM, et al. Determination of total phenolic, flavonoid content and free radical scavenging activities of common herbs and spices. J Pharmacogn Phytoche. 2014;104(33):104–108.
- Eleazu CO, Eleazu KC, Chukwuma S, et al. Review of the mechanism of cell death resulting from streptozotocin challenge in experimental animals, its practical use and potential risk to humans. J Diabetes Metab Disord. 2013;12(1):60.
- Furman BL. Streptozotocin‐induced diabetic models in mice and rats. Curr Protoc Pharmacol. 2015;70(1):5–47.
- Wu KK, Huan Y. Streptozotocin-induced diabetic models in mice and rats. Curr Protoc Pharmacol. 2008. Chapter 5:Unit5.47. https://www.ncbi.nlm.nih.gov/pubmed/22294227
- Gromada J, Dissing S, Kofod H, et al. Effects of the hypoglycaemic drugs repaglinide and glibenclamide on ATP-sensitive potassium-channels and cytosolic calcium levels in β TC3 cells and rat pancreatic beta cells. Diabetologia. 1995;38(9):1025–1032.
- Petchi RR, Vijaya C, Parasuraman S. Antidiabetic activity of polyherbal formulation in streptozotocin–nicotinamide induced diabetic wistar rats. J Tradit Complement Med. 2014;4(2):108–117.
- Fridlyand LE, Jacobson DA, Philipson LH. Ion channels and regulation of insulin secretion in human β-cells: a computational systems analysis. Islets. 2013;5(1):1–15.
- Ahmadian M, Duncan RE, Jaworski K, et al. Triacylglycerol metabolism in adipose tissue. Future Lipidol. 2007;2(2):229–237.
- Ueda K, Komine J, Matsuo M, et al. Cooperative binding of ATP and MgADP in the sulfonylurea receptor is modulated by glibenclamide. Proc Natl Acad Sci. 1999;96(4):1268–1272.
- Ojiako OA, Chikezie PC, Ogbuji AC. Blood glucose level and lipid profile of alloxan-induced hyperglycemic rats treated with single and combinatorial herbal formulations. J Tradit Complement Med. 2016;6(2):184–192.
- Ota A, Ulrih NP. An overview of herbal products and secondary metabolites used for management of type two diabetes. Front Pharmacol. 2017;8:436.
- Thamizharasan S, Umamaheswari S, Hari R. ɑ - Amylase and ɑ - Glucosidase Activity of Mimosa Pudica. Linn Flowers. PARIPEX- Indian Journal Res. 2016;5:223–4.
- Tasnuva ST, Qamar UA, Ghafoor K, Sahena F, Jahurul MH, Rukshana AH, et al. ɑ-glucosidase inhibitors isolated from Mimosa pudica L. Nat Prod Res. 2019;33:1495–9.
- Manzano S, Williamson G. Polyphenols and phenolic acids from strawberry and apple decrease glucose uptake and transport by human intestinal Caco-2 cells. Mol Nutr Food Res. 2010;54(12):1773–80.